Abstract
Background: Nucleic acid-based methods are increasingly used for screening of gastrointestinal parasites. Microscopy is still used and Swedish routine protocol consists of formalin ethyl-acetate concentration and do not include screening for trophozoites or Cryptosporidium spp. This study aimed to compare detection with the Swedish routine microscopy method to an extended method that includes screening for trophozoites and Cryptosporidium. Furthermore, we also developed a method for DNA recovery from SAF-fixed faecal samples and compared the real-time PCR detection of Giardia intestinalis, Dientamoeba fragilis, Cryptosporidium spp., Entamoeba histolytica and Entamoeba dispar from SAF-fixed and unpreserved faecal samples. PCR results were then compared with microscopy results.
Methods: SAF-fixed and unpreserved faecal samples from 1000 patients at the Clinical microbiology laboratory in Region Jönköping County, Sweden, were included. Samples were analysed with routine formalin ethyl-acetate concentration, wet mounts from both concentrated and unconcentrated samples, Ziehl–Neelsen staining on patients with certain symptoms and real-time PCR.
Results: We found a significant higher detection rate of parasites with the extended microscopy method compared to the Swedish routine microscopy method when SAF-fixed samples were used. The detection rate with real-time PCR in SAF-fixed samples was equal to the detection rate in unpreserved samples. There was no significant difference in detection comparing extended microscopy and real-time PCR.
Conclusion: In conclusion, this study showed that the extended microscopy method increased detection of intestinal protozoa with detection of both trophozoites and Cryptosporidium spp. We also showed that SAF-fixative can be used for detection of parasite-DNA with real-time PCR.
Introduction/background
Protozoan infections significantly contribute to the burden of gastrointestinal illness worldwide [Citation1]. In Sweden Cryptosporidium spp., Giardia intestinalis, Dientamoeba fragilis and Entamoeba histolytica are the most common and important gastrointestinal protozoa [Citation2,Citation3].
The routine microscopy protocol for intestinal parasites is Formalin ethyl-acetate concentration (FEC) in Sweden. Wet mounts of the concentrated sample are examined both with Iodine stained and unstained sediment to examine for helminth ova and for protozoan cysts.
Cryptosporidium are small which can make detection in wet mounts challenging [Citation4,Citation5]. The recommended method in Sweden is acid fast staining with a modified Ziehl–Neelsen (Z-N) stain after FEC, which is usually done on request and not as screening. Cryptosporidium can cause severe symptoms in immunocompromised patients and a fast diagnosis is important to prevent complications [Citation6]. However, clinical awareness is generally low, resulting in few requests [Citation7–10]. In Sweden, the reported incidences of Cryptosporidium vary, and a recent study suggests both diagnostic methods and testing algorithm to be the cause [Citation7]. The majority of Cryptosporidium spp. cases in Sweden are domestic [Citation3] and several outbreaks have been reported including two large drinking water-borne in 2010 and 2011 [Citation11,Citation12]. The number of cases diagnosed in Europe has increased during the last decade, probably due to higher awareness and better diagnostic methods [Citation9,Citation13].
G. intestinalis is the most common gastroenteritis causing protozoa in Sweden. The majority of patients with G. intestinalis are infected abroad [Citation3]. E. histolytica infections are rare but in the past the reporting of E. histolytica has been hampered by reporting of microscopic findings of E. histolytica/E. dispar- complex as E. histolytica which has overestimated the incidence [Citation14,Citation15]. The use of molecular typing has decreased the over-reporting. Dientamoeba fragilis is common, but confirmation of virulence and the potential mechanisms of pathogenicity are yet to be determined [Citation16–20]. Reported prevalence figures vary depending on methods used, patient population and location [Citation20–23]. Trophozoites of G. intestinalis and E. histolytica are analysed either as motile in fresh samples or by staining of SAF-fixed samples, mainly only on request. The recommended method for D. fragilis is trichrome staining of fresh or SAF-fixed samples [Citation24–26] and is usually done only on request.
Nucleic acid-based (NA) detection by PCR is increasingly used for screening of gastrointestinal parasites, replacing microscopy [Citation27,Citation28]. NA based detection offers greater sensitivity and specificity over microscopic tests [Citation29–31] and can process more samples in less time. The most common gastrointestinal parasites included in NA-based diagnostics in Sweden are E. histolytica, G. intestinalis and Cryptosporidium spp. Different cell lysis methods have been used prior to protozoan DNA extraction to minimize inhibitors of the PCR process [Citation32,Citation33] and to disrupt robust cell walls [Citation34–40]. A reduced yield of parasite-DNA has been reported from fixed samples [Citation41,Citation42].
The aim of this study was to compare an extended method for microscopy with the routine method and real-time PCR using both SAF-fixed and unpreserved faecal samples. The extended method, in addition to the routine method, included screening for trophozoites in filtered wet mounts from unconcentrated samples and for Cryptosporidium in concentrated wet mounts, and an algorithm for staining with Z-N not only on request but based on symptoms. Furthermore, we developed and evaluated a method for DNA extraction from both SAF-fixed and unpreserved faecal samples for G. intestinalis, D. fragilis, Cryptosporidium spp, E. histolytica and E. dispar.
Methods
Study population and samples
Two faecal samples each from 1000 sampling occasions of patients with suspected parasite infection were included. The patient received a package to collect stools and instructions to mix part of the stools immediately with 7 mL Sodium acetate acetic acid formaldehyde (SAF) solution (sample type A) and the other part was added to a sterile container (sample type B). The samples were sent to the Clinical microbiology laboratory in Region Jönköping County from March through August 2012. One part of sample type B was fixed in 7 mL of SAF at the laboratory (sample type C).
Microscopy
Formalin ethyl acetate concentration (FEC)
All samples of type A and C were homogenized, diluted to 10 mL with NaCl (0, 9%) containing 1% triton-X and then filtered through a metal sieve with 425 μm pores. Then about 7 mL was transferred to a centrifuge tube and 3 mL of ethyl acetate was added and the tubes were put in a head-over shaker for 1 min followed by centrifugation for 3 min at 1500×g. The formed plug of debris was removed and 1 mL of NaCl (0.9%) was added to the sediment. Microscopy was done with a Nikon eclipse 50i microscope (Nikon instruments Europe B.V, Amsterdam, The Netherlands) on one drop (approximately 100 µL) of sediment treated with D’Antoni’s Iodine stain (400×) and on one unstained drop (mainly 100×, larger magnification on findings) on the same slide with a 24 × 60 mm cover glass. Samples that appeared watery or mucoid were not concentrated, only filtered. In samples from patients with a suspicion of helminth infection, the whole deposit was used for microscopy.
Additional testing (Swedish routine protocol)
On request and on samples from immunocompromised patients or suspected outbreaks a modified Z-N stain was done to enable detection of Cryptosporidium spp. On request, a Van Gieson stain was done to enable detection of D. fragilis or trichrome stain for detection of trophozoites of Entamoeba spp. On request, direct wet mounts from fresh samples were done for motile trophozoite detection.
Additional testing (extended method)
The remaining filtered faecal suspension was analysed by analysing at least one drop of the unconcentrated material (400×). D. fragilis, G. intestinalis and Entamoeba spp. trophozoites were detected in wet mounts based on the characteristic appearance, shape and size. A van Gieson stain was used to verify D. fragilis nuclei appearance. Entamoeba spp. trophozoites with intracellular erythrocytes were considered E. histolytica, Entamoeba spp. trophozoites with no intracellular erythrocytes were not differentiated with microscopy. The unconcentrated sediment was put on the same slide as the concentrated and a 24 × 60 mm cover glass was used.
All concentrated samples were carefully screened in D’Antonis stained wet mounts (400×) for the characteristic unstained appearance of Cryptosporidium spp. Samples from patients with a history of acute diarrhoea (lasting < 1 month), fever, vomiting, muscle pain, animal contact, immunosuppression, when an outbreak was suspected or with suspected wet mount findings was stained with modified Z-N for detection and verification of Cryptosporidium spp.
The PCR results were not available for the microscopist. After analysis, discrepant results for real-time PCR and extended microscopy were re-evaluated with both methods.
Isolation of DNA
Cell lysis
The SAF-fixed samples A and C were homogenized and filtered as described for microscopy. Thereafter, 1 mL was centrifuged for 1 min at 10,000 g. The supernatant was discarded, and the sediment was suspended in 1 ml of Phosphate-buffered saline (PBS) and then centrifuged for 1 min at 10,000 g. The supernatant was discarded, and the sediment was re-suspended in 280 µL of AL buffer (Qiagen, Hilden, Germany) and 20 µL of proteinaseK (Qiagen). The re-suspension was then incubated at 56 °C for 1 h with gentle agitation followed by treatment for 1 h in liquid nitrogen, repeated once and was then incubated at 98 °C with gentle agitation for 20 min. Finally, the material was centrifuged for 1 min at 10,000 g and the supernatants were transferred to new tubes and stored in −20 °C prior to extraction.
About 300 µL of sample type B was transferred with a sterile loop to a micro centrifuge tube containing 280 µL of AL buffer (Qiagen) and 20 µL of proteinaseK (Qiagen). Then the samples were analysed as described for re-suspended sample types A and C.
DNA extraction
Sample types A, B and C were subjected to the same DNA extraction protocol, done with a MagAttract DNA Mini M48 kit (Qiagen) in an M48 instrument (Qiagen), according to the manufacturer’s instructions. The elution volume was 200 µL.
Multiplex real-time PCR
Real-time PCR was done with a LightCycler 480 II instrument (Roche Diagnostics GmbH, Mannheim, Germany). Primer and probe sequences and references are indicated in . Thermal cycling protocol was 95 °C for 5 min followed by 50 cycles of 95 °C for 5 s, 60 °C for 15 s and 72 °C for 15 s. Two PCR mixtures was used, the first included primer and probes specific for E. dispar, E. histolytica and D. fragilis, and the second specific for Cryptosporidium spp., G. intestinalis and the PCR inhibition control Phocine herpesvirus (PhHV). Template volume was 5 µL in a total volume of 25 µL which include LightCycler 480 Probes Master (Roche Diagnostics GmbH) and additionally 2 µL 25 mM MgCl2. Detection limit was tested with 10-fold dilution series of plasmid controls for all targets both as single and multiplex.
Table 1. Primer and probe sequences used.
Reproducibility real-time PCR
To determine the reproducibility in isolation of DNA and real-time PCR for the different sample types, a pool of positive unpreserved samples was done for each parasite (G. intestinalis, E. dispar, D. fragilis and Cryptosporidium spp.). Each pool was divided in two portions and one was fixed in SAF. All samples were kept in 4 °C for a minimum of 24 h and a maximum of 72 h prior to extraction of DNA. From each portion, 10 suspensions were done, and all followed the cell lysis and DNA extraction described previously. The suspensions were then analysed with the real-time PCR and the Ct-values were compared.
Statistical analysis
A chi-squared test was used to compare the detection of parasites with the different methods. A Mann Whitney test was used to compare the Ct-values for the different sample types. Statistical analysis was done with Statistica 13.3.1 (StatSoft, Inc. Tulsa, OK) and a significance level of .05 was used.
Results
Cryptosporidium spp
Results are shown in . There was no difference in detection between sample types. The extended microscopy method detected statistically significantly more Cryptosporidium compared to the routine method (p < .01).
Table 2. Results of microscopy and real-time PCR for sample types A, B and C.
With the extended method a total of 21 Cryptosporidium was detected, 18 of them were detected in wet mount screening () and verified with Z-N and 3 were only detected in Z-N performed according to the extended staining algorithm due to presence of specific symptoms. The three that were not found in wet mount screening but with staining contained few oocysts (in two samples) and one was a sample that contained many leukocytes. Six of the Cryptosporidium detected in wet mount screening lacked clinical data, the other were also stained because of the extended staining algorithm due to the presence of specific symptoms. In total, 237 samples were stained due to clinical data. Of the Cryptosporidium spp. positive samples with the routine method, two where clinically suspected and three were part of a small known Cryptosporidium outbreak.
Figure 1. Cryptosporidium in D’Antonis stained wet mount of ethyl-acetate concentrated faecal sample (Black arrows). 400× magnification.
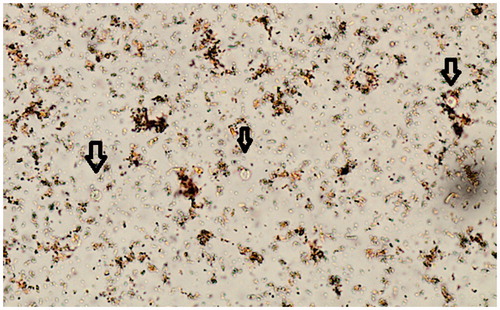
There was no difference in detection between the extended microscopy method and real-time PCR. One sample was negative for Cryptosporidium spp. in microscopy but positive in real-time PCR (high Ct-value). Another sample was positive in microscopy but negative by real-time PCR. A sample taken another day from the same patient was positive in both methods.
Entamoeba histolytica/dispar
Results are shown in . No fresh samples were submitted during the study. No request for E. histolytica trophozoites was made.
There was no statistically significant difference in detection of Entamoeba histolytica/dispar cysts between the different sample types for the microscopy methods. There was a statistically significant difference in detection of Entamoeba spp. trophozoites between the sample types (p < .01) for the extended method. No ingested erythrocytes were observed. Two E. histolytica/E. dispar microscopy positive samples (cysts) were negative in the real-time PCR. Out of the eight Entamoeba spp. trophozoites detected with the extended method, one was positive for E. histolytica-DNA and one for E. dispar-DNA. There was no difference in detection of E. histolytica/dispar- DNA between the sample types for real-time PCR.
Giardia intestinalis
No requests for G. intestinalis trophozoites were made. Results are shown in .
There was no statistically significant difference in detection of G. intestinalis cysts between the sample types for the routine method or the extended microscopy method. There was a statistically significant difference in detection of G. intestinalis trophozoites between the sample types in the extended method (p = .007). There was no difference in detection of G. intestinalis-DNA between the sample types for real-time PCR.
There was no statistically significant difference in detection of G. intestinalis between extended microscopy and real-time PCR (p = .69)
Two of the samples with G. intestinalis that were negative with extended microscopy but positive with real-time PCR were confirmed to be microscopy positive in a re-evaluation. Both samples contained numerous B. hominis and many apathogenic cysts. The remaining PCR-positive but microscopy-negative samples had Ct-values of >36 and could not be found in re-evaluation.
Dientamoeba fragilis
Results are shown in . There was a statistically significant difference in detection between the routine method and the extended method (p < .01). There was a statistically significant difference in detection of D. fragilis trophozoites between the sample types for the extended method (p < .01). There was no difference in detection of D. fragilis-DNA between sample types for real-time PCR.
There was no statistically significant difference in detection between extended method and real-time PCR (p = .07).
The real-time positive but microscopy negative samples were not positive in re-evaluation of the samples.
Real-time PCR
There was no difference in detection between sample types. The detection limit was ≤10 copies for all parasites included. The Ct values obtained from testing the dilution series of each pathogen as single and as multiplex assay were similar, and the same analytical sensitivity was achieved. The performance of the assays was not influenced by the presence of other primers, the presence of DNA from the internal control, or the presence of DNA from the other targets.
Reproducibility Ct-value
Median and 25th and 75th percentile and maximum and minimum Ct-values for the different parasites and different sample types in the reproducibility testing are shown in . No statistically significant difference between sample types and Ct-value was found (p = .75) and the mean CV of Ct-values between the different suspensions of the same sample was 2.8%.
Discussion
The routine microscopy method used in Sweden and in this study referred to as the routine method is similar to routine methods described in other studies in Europe [Citation24,Citation43–45].
Cryptosporidium spp. is one of the most important protozoan infections in Sweden and detection is necessary to determine the true incidence, learn more about the burden of this infection and to prevent secondary transmissions and outbreaks [Citation7,Citation46]. In this study, the routine microscopy method included staining for Cryptosporidium when requested and no extended wet mount screening was done. Some laboratories in Sweden use wet mount screening and some do not [Citation7] and in this study we chose to compare our extended method with no wet mount screening for Cryptosporidium. In the present study Cryptosporidium was requested during a known small outbreak and only very sporadically on other symptomatic patients. The extended method adds specific screening, for unstained, refractile, spherical objects with the small size (4–6µm), of the Iodine stained sediment () and an extended staining algorithm that includes staining based on symptoms and not specific request only. Few studies support the use of wet mounts for screening of Cryptosporidium, but it is recognized that experienced technicians can detect them in wet mounts [Citation4,Citation5] and since wet mounts are always used for screening for other parasites in Sweden, it is an advantage to use them for Cryptosporidium screening as well. In a clinical laboratory, the majority of samples are from symptomatic patients, even if symptoms vary, and symptomatic patients often have number of oocysts high enough for detection in wet mounts [Citation4], and even if the risk of missing asymptomatic carriers or patients recently having an infection, wet mount screening is still useful as first line diagnostics if microscopy is used. Training in appearance of Cryptosporidium in wet mounts is not commonly included in basic training, but we can show here that if it is, it can increase detection. It has been part of basic training here for more than a decade and the incidence in this region is among the highest incidences of Cryptosporidium in Sweden [Citation7]. All suspected wet mount findings are verified with Z-N. The amount of Cryptosporidium oocysts in this study varied from multiple per vision field to a few per slide. It is important for sediments to have a minimum of faecal debris, especially when detecting the small Cryptosporidium and the properties of FEC vary and several factors influence the ability to decrease faecal debris in the sediment and thereby the result, for instance centrifugation time and speed, filtration pore size, preservative and sample quality [Citation47]. The use of metal sieves may have improved yield, studies have shown that Cryptosporidium can end up in the gauze that is traditionally used for filtration [Citation48], but it was not part of the study.
With the extended method detection of E. histolytica/dispar, G. intestinalis and D. fragilis trophozoites increased compared to routine microscopy. No trophozoites were detected in unpreserved (sample type C), confirming that prompt fixation is essential for preserving trophozoite morphology [Citation24] and that fixation at the laboratory is not enough. Few trophozoites were detected in concentrated samples, and FEC was not suitable for trophozoites. Six of the eight samples that contained only trophozoites of G. intestinalis was from a group of people that had been in India and came back with severe diarrhoea, the other two lacked clinical data. The one sample with only E. histolytica trophozoites had clinical data of diarrhoea, but no other history was given. No fresh samples were submitted during the study, so its usefulness could not be evaluated. No requests for trophozoites were made. Even though the trophozoites are immobile, direct wet (filtered material) mounts were found useful for trophozoite detection, and adds clinical value since trophozoite excretion of G. intestinalis and E. histolytica is more common in diarrheal patients [Citation28]. Other methods can then be used for verification, both permanent staining and NA-methods but for screening, the filtered wet mounts are time-effective and sensitive. In this study, relying on only clinical request for trophozoites of G. intestinalis and Entamoeba histolytica would have led to missed cases. Only one out of 36 E. histolytica/E. dispar cysts was positive for E. histolytica with real-time PCR, which underlines the need for typing of these species [Citation14,Citation49,Citation50]. The pathogenic role of D. fragilis has been debated [Citation16–18,Citation21,Citation51,Citation52] and the need for screening is questioned. A recent study from Finland also shows that wet mount screening was useful for D. fragilis detection [Citation20].
In the present study, no difference in detection of parasite-DNA between SAF-fixed faecal samples and unpreserved faecal samples was found. In previous studies, fixation with formaldehyde had negative effects on DNA recovery [Citation42]. However, other studies have successfully used formalin fixed samples for PCR [Citation53]. The time between fixation and lysis/extraction was between 1 and 5 days in the present study. Longer time of fixation may impact the diagnostic accuracy, but this has not been investigated in the present study. Lysis and extraction of DNA are important and can influence the results [Citation39,Citation40,Citation54]. However, the method in this study showed no statistically significant difference in reproducibility between fixed and unpreserved samples. The highest CV was found in with E. dispar and G. intestinalis in sample type B. The samples were formed and hard to homogenize and did contain a mix of different positive samples. There was no significant difference between extended microscopy (sample type A) and real-time PCR for the detection. This is contradictory to other studies comparing microscopy and real-time PCR [Citation30,Citation31,Citation55]. The PCR method used here has been shown to be both robust and sensitive in previous studies [Citation25,Citation30,Citation56] and the sensitivity when plasmid dilution series was tested showed limit of detection of 10 copies in this study.
To actively screen for trophozoites and Cryptosporidium increased detection with microscopy and – like all other parasitological microscopy – it requires a well-trained trained technician. Learning microscopy is time-consuming and demanding, but in our opinion it should not add much in the total training to include Cryptosporidium in wet mounts and trophozoites in wet mounts. Total hands-on time including preparation for the extended method in our is setting estimated to 7–12 min per sample which is less than reported by others [Citation57]. Time of examination per slide is dependent both on the experience and competence of the microscopist, but also on the amount of faecal debris, and optimization of the properties of FEC can decrease time [Citation47] and also, experience decrease time spent per sample. The addition of more staining can be optimized by staining in batch, so that all steps only need to be done once. Certain samples can be more time-consuming for example if specific suspicion of some helminths is present. It is uncommon enough not to influence the mean time. The time added per sample with the extended method compared to routine is estimated to 2–4 minutes per sample. One slide is read per sample, with both concentrated and unconcentrated material. There is however a maximum of number of samples that can be read by one person within a workday to maintain diagnostic quality. With the standard method all patients are screened for cysts and ova of helminths. A more rational approach would be to, like with the extended method and with NA detection, to screen for all stages of G. intestinalis, E. histolytica, D. fragilis and for Cryptosporidium in addition to other parasites. The most common (and pathogen) should not require special requests, the diagnostics (both NA-based and microscopy) should be adjusted to fit the needs of the setting. The use of only the Swedish routine microscopy method has little diagnostic value which is also shown in other settings with similar routine microscopy protocol [Citation45,Citation58].
Despite the fact that we found no statistically significant difference in detection between extended microscopy and PCR in this study, NA detection has obvious advantages. It is not dependent on development stage of the parasite, fixation or the same amount of competence for staff and it allows for further characterization of species and subspecies. Depending on platform and assays used, the number of samples that can be analysed within a workday can vary, but the hands-on time per sample will be less compared to microscopy and the quality of results is not dependent on number of samples. For NA detection it is believed that one sample is enough [Citation59] compared to recommendations of using multiple samples for microscopy [Citation4,Citation24].
If microscopy is used less, skilled clinical parasitologists who are familiar with microscopic diagnosis of parasitic diseases will be lost [Citation27]. The clinical awareness of the most common parasites is shown in this study and in other studies [Citation8,Citation9] to be low. With increased travels, increased immigration and possible climate changes [Citation60], which parasites are found in specific settings can change and that kind of change can be hard to detect if only PCR is used. An increased knowledge of parasitic infections is needed and NA-detection of more uncommon parasites needs evaluation in different settings and specific patient groups to evaluate if there is a need of screening for other parasites.
In conclusion, routine microscopy can be optimized with the extended microscopy which increases detection of the most important protozoa in Sweden; Cryptosporidium, E. histolytica, G. intestinalis and D. fragilis compared to Swedish routine microscopy and shows comparable sensitivity to real-time PCR. Furthermore, we show that SAF-fixative can be used for detection of parasite-DNA, which can facilitate the use of the same sample for both microscopy and real-time PCR. This enables the differentiation between Entamoeba spp. and clinically suspected cases with negative microscopy.
Disclosure statement
No potential conflict of interest was reported by the authors.
References
- Fletcher SM, Stark D, Harkness J, et al. Enteric protozoa in the developed world: a public health perspective. Clin Microbiol Rev. 2012;25(3):420–449.
- Insulander M, Silverlas C, Lebbad M, et al. Molecular epidemiology and clinical manifestations of human cryptosporidiosis in Sweden. Epidemiol Infect. 2013;141(5):1009–1020.
- Public Health Agency of Sweden. Surveillance of communicable diseases Sweden, on Public health agency of Sweden. Available from: https://www.folkhalsomyndigheten.se/folkhalsorapportering-statistik/statistikdatabaser-och-visualisering/sjukdomsstatistik/
- Garcia LS, Arrowood M, Kokoskin E, et al. Laboratory diagnosis of parasites from the gastrointestinal tract. Clin Microbiol Reviews. 2018;31:e00025–e00017.
- Khurana S, Chaudhary P. Laboratory diagnosis of cryptosporidiosis. Trop Parasitol. 2018;8(1):2–7.
- Checkley W, White AC Jr, Jaganath D, et al. A review of the global burden, novel diagnostics, therapeutics, and vaccine targets for cryptosporidium. Lancet Infect Dis. 2015;15(1):85–94.
- Harvala H, Ogren J, Boman P, et al. Cryptosporidium infections in Sweden-understanding the regional differences in reported incidence. Clin Microbiol Infect. 2016;22(12):1012–1013.
- Munoz FM, Attias E, Czinn SJ, et al. Paediatrician and caregiver awareness of cryptosporidiosis and giardiasis in children: US survey responses. Zoonoses Public Health. 2016;63(5):410–419.
- Cacciò SM, Chalmers RM. Human cryptosporidiosis in Europe. Clin Microbiol Infect. 2016;22(6):471–480.
- McHardy IH, Wu M, Shimizu-Cohen R, et al. Detection of intestinal protozoa in the clinical laboratory. J Clin Microbiol. 2014;52(3):712–720.
- Rehn M, Wallensten A, Widerstrom M, et al. Post-infection symptoms following two large waterborne outbreaks of Cryptosporidium hominis in Northern Sweden, 2010-2011. BMC Public Health. 2015;15:529.
- Widerstrom M, Schonning C, Lilja M, et al. Large outbreak of Cryptosporidium hominis infection transmitted through the public water supply, Sweden. Emerg Infect Dis. 2014;20:581–589.
- Fournet N, Deege MP, Urbanus AT, et al. 2013. Simultaneous increase of Cryptosporidium infections in the Netherlands, the United Kingdom and Germany in late summer season. Robert Koch-Institut, Infektionsepidemiologie; 2012.
- Lebbad M, Svard SG. PCR differentiation of Entamoeba histolytica and Entamoeba dispar from patients with amoeba infection initially diagnosed by microscopy. Scand J Infect Dis. 2005;37(9):680–685.
- Shirley D-A, Farr L, Watanabe K, et al. A review of the global burden, new diagnostics, and current therapeutics for amebiasis. Open Forum Infect Dis. 2018;5(7):ofy161–ofy161.
- Ogren J, Dienus O, Lofgren S, et al. Dientamoeba fragilis prevalence coincides with gastrointestinal symptoms in children less than 11 years old in Sweden. Eur J Clin Microbiol Infect Dis. 2015;34:1995–1998.
- Barratt JL, Harkness J, Marriott D, et al. A review of Dientamoeba fragilis carriage in humans: several reasons why this organism should be considered in the diagnosis of gastrointestinal illness. Gut Microbes. 2011;2(1):3–12.
- Holtman GA, Kranenberg JJ, Blanker MH, et al. Dientamoeba fragilis colonization is not associated with gastrointestinal symptoms in children at primary care level. FAMPRJ. 2017;34(1):25–29.
- Stark D, Garcia LS, Barratt JL, et al. Description of Dientamoeba fragilis cyst and precystic forms from human samples. J Clin Microbiol. 2014;52(7):2680–2683.
- Pietilä J-P, Meri T, Siikamäki H, et al. Dientamoeba fragilis – the most common intestinal protozoan in the Helsinki Metropolitan Area, Finland, 2007 to 2017. Eurosurveillance. 2019;24(29):1800546.
- Jokelainen P, Hebbelstrup Jensen B, Andreassen BU, et al. Dientamoeba fragilis, a commensal in children in Danish day care centers. J Clin Microbiol. 2017;55(6):1707–1713.
- Bartolini A, Zorzi G, Besutti V. Prevalence of intestinal parasitoses detected in Padua teaching hospital, Italy, March 2011 - February 2013. Infez Med. 2017;25(2):133–141.
- van Hattem JM, Arcilla MS, Grobusch MP, et al. Travel-related acquisition of diarrhoeagenic bacteria, enteral viruses and parasites in a prospective cohort of 98 Dutch travellers. Travel Med Infect Dis. 2017;19:33–36.
- van Gool T, Weijts R, Lommerse E, et al. Triple Faeces Test: an effective tool for detection of intestinal parasites in routine clinical practice. Eur J Clin Microbiol Infect Dis. 2003;22(5):284–290.
- Verweij JJ, Mulder B, Poell B, et al. Real-time PCR for the detection of Dientamoeba fragilis in fecal samples. Mol Cell Probes. 2007;21(5–6):400–404.
- Stark D, Barratt J, Roberts T, et al. Comparison of microscopy, two xenic culture techniques, conventional and real-time PCR for the detection of Dientamoeba fragilis in clinical stool samples. Eur J Clin Microbiol Infect Dis. 2010;29(4):411–416.
- van Lieshout L, Roestenberg M. Clinical consequences of new diagnostic tools for intestinal parasites. Clin Microbiol Infect. 2015;21(6):520–528.
- Garcia LS, Arrowood M, Kokoskin E, et al. Laboratory diagnosis of parasites from the gastrointestinal tract. Clin Microbiol Rev. 2018;31(1):e00025-17.
- Schuurman T, Lankamp P, van Belkum A, et al. Comparison of microscopy, real-time PCR and a rapid immunoassay for the detection of Giardia lamblia in human stool specimens. Clin Microbiol Infect. 2007;13(12):1186–1191.
- Verweij JJ, Blange RA, Templeton K, et al. Simultaneous detection of Entamoeba histolytica, Giardia lamblia, and Cryptosporidium parvum in fecal samples by using multiplex real-time PCR. J Clin Microbiol. 2004;42(3):1220–1223.
- Stark D, Al-Qassab SE, Barratt JL, et al. Evaluation of multiplex tandem real-time PCR for detection of Cryptosporidium spp., Dientamoeba fragilis, Entamoeba histolytica, and Giardia intestinalis in clinical stool samples. J Clin Microbiol. 2011;49(1):257–262.
- Hawash Y. DNA extraction from protozoan oocysts/cysts in feces for diagnostic PCR. Korean J Parasitol. 2014;52(3):263–271.
- Monteiro L, Bonnemaison D, Vekris A, et al. Complex polysaccharides as PCR inhibitors in feces: Helicobacter pylori model. J Clin Microbiol. 1997;35(4):995–998.
- Elwin K, Robinson G, Hadfield SJ, et al. A comparison of two approaches to extracting Cryptosporidium DNA from human stools as measured by a real-time PCR assay. J Microbiol Methods. 2012;89(1):38–40.
- Halstead FD, Lee AV, Couto-Parada X, et al. Universal extraction method for gastrointestinal pathogens. J Med Microbiol. 2013;62(Pt 10):1535–1539.
- Babaei Z, Oormazdi H, Rezaie S, et al. Giardia intestinalis: DNA extraction approaches to improve PCR results. Exp Parasitol. 2011;128(2):159–162.
- Forsell J, Koskiniemi S, Hedberg I, et al. Evaluation of factors affecting real-time PCR performance for diagnosis of Entamoeba histolytica and Entamoeba dispar in clinical stool samples. J Med Microbiol. 2015;64(9):1053–1062.
- Vianna EN, Costa JO, Santos CK, et al. An alternative method for DNA extraction and PCR identification of Entamoeba histolytica and E. dispar in fecal samples. Parasitology. 2009;136(7):765–769.
- Menu E, Mary C, Toga I, et al. Evaluation of two DNA extraction methods for the PCR-based detection of eukaryotic enteric pathogens in fecal samples. BMC Res Notes. 2018;11(1):206.
- Adamska M, Leońska-Duniec A, Maciejewska A, et al. Comparison of efficiency of various DNA extraction methods from cysts of Giardia intestinalis measured by PCR and TaqMan real time PCR. Parasite. 2010;17(4):299–305.
- Troll H, Marti H, Weiss N. Simple differential detection of Entamoeba histolytica and Entamoeba dispar in fresh stool specimens by sodium acetate-acetic acid-formalin concentration and PCR. J Clin Microbiol. 1997;35(7):1701–1705.
- Ramos F, Zurabian R, Morán P, et al. The effect of formalin fixation on the polymerase chain reaction characterization of Entamoeba histolytica. Trans R Soc Trop Med Hyg. 1999;93(3):335–336.
- Vandenberg O, Van Laethem Y, Souayah H, et al. Improvement of routine diagnosis of intestinal parasites with multiple sampling and SAF-fixative in the triple-faeces-test. Acta Gastroenterol Belg. 2006;69(4):361–366.
- Manser M, Granlund M, Edwards H, et al. Detection of Cryptosporidium and Giardia in clinical laboratories in Europe-a comparative study. Clin Microbiol Infect. 2014;20(1):O65–O71.
- Ten Hove R, Schuurman T, Kooistra M, et al. Detection of diarrhoea-causing protozoa in general practice patients in The Netherlands by multiplex real-time PCR. Clin Microbiol Infect. 2007;13(10):1001–1007.
- Vanathy K, Parija SC, Mandal J, et al. Cryptosporidiosis: a mini review. Trop Parasitol. 2017;7(2):72–80.
- Manser MM, Saez ACS, Chiodini PL. Faecal parasitology: concentration methodology needs to be better standardised. PLoS Negl Trop Dis. 2016;10(4):e0004579.
- Weber R, Bryan RT, Bishop HS, et al. Threshold of detection of Cryptosporidium oocysts in human stool specimens: evidence for low sensitivity of current diagnostic methods. J Clin Microbiol. 1991;29(7):1323–1327.
- Visser LG, Verweij JJ, Van Esbroeck M, et al. Diagnostic methods for differentiation of Entamoeba histolytica and Entamoeba dispar in carriers: performance and clinical implications in a non-endemic setting. Int J Med Microbiol. 2006;296(6):397–403.
- Van Den Broucke S, Verschueren J, Van Esbroeck M, et al. Clinical and microscopic predictors of Entamoeba histolytica intestinal infection in travelers and migrants diagnosed with Entamoeba histolytica/dispar infection. PLoS Negl Trop Dis. 2018;12(10):e0006892.
- Wong ZW, Faulder K, Robinson JL. Does Dientamoeba fragilis cause diarrhea? A systematic review. Parasitol Res. 2018;117(4):971–980.
- Roser D, Simonsen J, Nielsen HV, et al. Dientamoeba fragilis in Denmark: epidemiological experience derived from four years of routine real-time PCR. Eur J Clin Microbiol Infect Dis. 2013;32:1303–1310.
- Lee MF, Lindo JF, Auer H, et al. Successful extraction and PCR amplification of Giardia DNA from formalin-fixed stool samples. Exp Parasitol. 2019;198:26–30.
- Autier B, Belaz S, Razakandrainibe R, et al. Comparison of three commercial multiplex PCR assays for the diagnosis of intestinal protozoa. Parasite. 2018;25:48.
- Bruijnesteijn van Coppenraet LE, Wallinga JA, Ruijs GJ, et al. Parasitological diagnosis combining an internally controlled real-time PCR assay for the detection of four protozoa in stool samples with a testing algorithm for microscopy. Clin Microbiol Infect. 2009;15(9):869–874.
- Boadi S, Polley SD, Kilburn S, et al. A critical assessment of two real-time PCR assays targeting the (SSU) rRNA and gdh genes for the molecular identification of Giardia intestinalis in a clinical laboratory. J Clin Pathol. 2014;67:811.
- Svraka-Latifovic S, Bouter S, Naus H, et al. Impact of transition from microscopy to molecular screening for detection of intestinal protozoa in Dutch patients. Clin Microbiol Infect. 2014;20(11):O969–O971.
- Stensvold CR, Nielsen HV. Comparison of microscopy and PCR for detection of intestinal parasites in Danish patients supports an incentive for molecular screening platforms. J Clin Microbiol. 2012;50(2):540–541.
- Rijsman LH, Monkelbaan JF, Kusters JG. Clinical consequences of polymerase chain reaction-based diagnosis of intestinal parasitic infections. J Gastroenterol Hepatol. 2016;31(11):1808–1815.
- Short EE, Caminade C, Thomas BN. Climate change contribution to the emergence or re-emergence of parasitic diseases. Infect Dis (Auckl). 2017;10:1–7.
- Fontaine M, Guillot E. Development of a TaqMan quantitative PCR assay specific for Cryptosporidium parvum. FEMS Microbiol Lett. 2002;214(1):13–17.
- Niesters HG. Molecular and diagnostic clinical virology in real time. Clin Microbiol Infect. 2004;10(1):5–11.