Abstract
Background
Orthohantaviruses are rodent-borne emerging viruses that cause haemorrhagic fever with renal syndrome (HFRS) in Eurasia and hantavirus pulmonary syndrome in America. Transmission between humans have been reported and the case-fatality rate ranges from 0.4% to 40% depending on virus strain. There is no specific and efficient treatment for patients with severe HFRS. Here, we characterised a fatal case of HFRS and sequenced the causing Puumala orthohantavirus (PUUV).
Methods
PUUV RNA and virus specific neutralising antibodies were quantified in plasma samples from the fatal case and other patients with non-fatal PUUV infection. To investigate if the causing PUUV strain was different from previously known strains, Sanger sequencing was performed directly from the patient’s plasma. Biopsies obtained from autopsy were stained for immunohistochemistry.
Results
The patient had approximately tenfold lower levels of PUUV neutralising antibodies and twice higher viral load than was normally seen for patients with less severe PUUV infection. We could demonstrate unique mutations in the S and M segments of the virus that could have had an impact on the severity of infection. Due to the severe course of infection, the patient was treated with the bradykinin receptor inhibitor icatibant to reduce bradykinin-mediated vessel permeability and maintain vascular circulation.
Conclusions
Our data suggest that bradykinin receptor inhibitor may not be highly efficient to treat patients that are at an advanced stage of HFRS. Low neutralising antibodies and high viral load at admission to the hospital were associated with the fatal outcome and may be useful for future predictions of disease outcome.
Introduction
Orthohantaviruses (genus orthohantavirus, family Hantaviridea) are rodent-borne viruses with a global impact due to their world-wide distribution and significant mortality rates [Citation1]. One of two acute febrile illnesses can develop depending on virus strain: haemorrhagic fever with renal syndrome (HFRS) in Europe and Asia, and hantavirus pulmonary syndrome (HPS) in the Americas, which is less common but has a mortality rate up to 40% [Citation1,Citation2].
Puumala orthohantavirus (PUUV) is endemic in the Central and Northern Europe with a peak incidence of >300 cases per 100,000 inhabitants with an overall case-fatality rate of 0.4%, and 2–6% for elderly patients [Citation3]. The symptoms include fever, headache, myalgia, abdominal pain, blurred vision and oliguria [Citation1]. Thrombocytopenia and renal impairment are frequent and diagnostic for the disease. One third of diagnosed patients are hospitalised and may develop hypotension due to capillary leakage [Citation4].
PUUV is an enveloped negative single stranded RNA virus composed of three circular genomic segments, Small (S), Medium (M), and Large (L). The S segment, encodes a structural nucleocapsid protein (N), and a non-structural protein (NS). The M segment, encodes two glycoproteins, Gn and Gc that are known to harbour important antigenic sites influencing the quality of the humoral immune response during human infection. The L segment, encodes the RNA-dependent RNA polymerase (RdRp) [Citation5].
Currently, there is no specific treatment available against human orthohantavirus infections. However, treatment with convalescent plasma has been tested [Citation6], and several studies have highlighted the protective activity of neutralising antibodies (NAb) against HFRS and HPS in order to clear the virus [Citation6–8]. A correlation between low levels of NAbs and severity of disease has been reported. Icatibant, a selective, competitive antagonist to bradykinin, used for inflammatory conditions with increased vascular leakage such as hereditary angioedema [Citation9], has been sporadically administered in severe PUUV infections with promising results [Citation10–12].
Herein, we describe a severe case of PUUV-infection, including an analysis of humoral immune responses, virus dissemination and results from viral sequencing.
Results
A 79-year-old male patient was admitted to the department of infectious diseases after less than 2 days of fever, rigours, shortness of breath and fatigue. He was a previous smoker, had a history of obstructive sleep apnoea syndrome and took oral medication for hypertension and type II diabetes. At admission, he had a body temperature of 38.7 °C, a pulse oximetry saturation of 86% on room air, a respiratory rate of 30 breaths per minute, and blood pressure of 110/70 mmHg. Laboratory results () revealed leukocytosis with neutrophilia and lymphopenia, together with thrombocytopenia, decreased serum albumin, and a increased level of C-reactive protein. PCR for influenza A and B was negative. Bacterial blood cultures were negative.
Table 1. Laboratory findings in a fatal case of PUUV infection day 2–5 after onset of disease symptoms.
The patient initially received intravenous penicillin G under the suspicion of a bacterial pneumonia, as well as rehydration therapy, nasal cannula oxygen supplementation, and paracetamol intravenously. A chest X-ray demonstrated a minimum of unilateral pleural effusion. At day two post-onset of fever (POF) an anti-PUUV IgM rapid test (Reascan, Reagena, Helsinki, Finland) and a PUUV-specific quantitative RT-PCR from serum were found positive, and penicillin-treatment was discontinued. Day three POF the patient detoriated including developed hypotension with a systolic pressure of 50 mmHg. The patient became anuric and was transferred to the intensive care unit for vasoactive drug treatment, intubation and mechanical ventilation. During day four POF the circulatory dysfunction worsened with pronounced capillary leakage and vasoplegia. Cardiac ultrasound revealed hypovolemia but with normal biventricular function. The patient developed refractory circulatory shock and a single dose of the bradykinin receptor inhibitor icatibant (30 mg) was given subcutaneously, although without any apparent beneficiary effect. The patient succumbed in circulatory shock with multiorgan failure 12 h later.
At autopsy scattered punctuate haemorrhages <1 mm in size were detected in the ventriculus and duodenum, but no other haemorrhages were found. Post-mortem investigation of biopsies revealed neutrophil infiltration in multiple organs and abundance of PUUV infected endothelial cells (). The highest levels of PUUV RNA quantified with qRT-PCR was found in the lungs, kidneys and spleen (supplementary Table 1). Furthermore, PUUV RNA was also found in the liver, lymph nodes, heart and bone marrow. In addition, the patient manifested with higher viremia than normally found in PUUV-caused HFRS patients during the five first days of disease (). We also found that the patient had a delayed PUUV-specific antibody response, in comparison with individuals where the PUUV-infection had a non-fatal outcome (). Details of the methods are provided in the supplementary material.
Figure 1. Presence of PUUV and inflammatory cells in the peripheral lung of a fatal case of PUUV infection. (A) PUUV nucleocapsid protein detected by polyclonal antibody. (B) Control, staining with isotype antibody for unspecific binding. (C) Neutrophils staining for elastase. (D) CD68 positive cells. Biopsies were embedded in glycol methacrylate (GMA) and used for immunohistochemistry staining. Photos are taken at ×10 (A–C) and ×20 (D) magnification and scale bars represents 200 µm and 100 µm, respectively. For details on antibodies see Supplementary table 4.
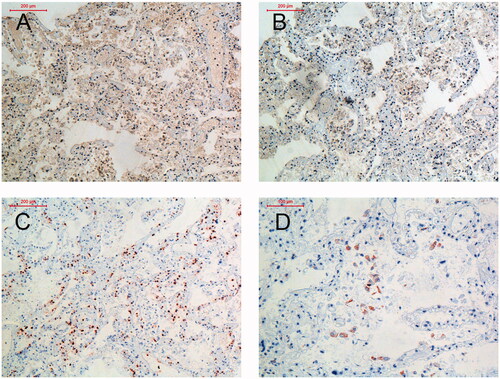
Figure 2. Viral load in plasma by qPCR for the fatal case in relation to non-fatal PUUV cases (n = 20 sampled median 6 days, range 3–7 days, post-onset of disease) (A). The kinetics of viral load is shown for the fatal case in comparison to three other patients with severe disease (B).
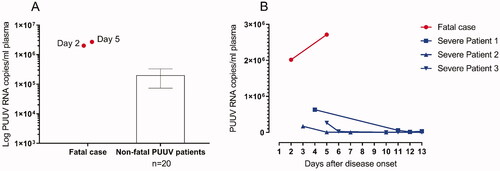
Figure 3. Neutralisation titres against PUUV for the fatal case are indicated with red dots for day two and five post disease onset and are compared with the mean neutralisation titres of non-fatal cases (n = 20 sampled median 6 days, range 2–13 days, post-onset of disease.) (A). The kinetics for neutralising antibodies show lower titres for the fatal case compared to patients with mild and severe infection (n = 5) (B). Neutralising antibodies in plasma were measured by Trophos using Vero E6 cells infected with the Kazan strain of PUUV.
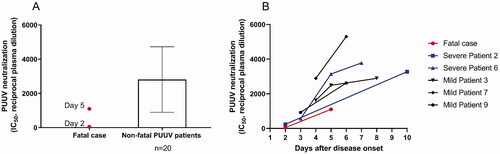
To investigate whether the causing PUUV was different from previously described strains, Sanger sequencing was performed on amplicons derived from a nested-PCR using viral PUUV RNA extracted directly from the patient’s plasma. Hence, no in vitro propagation of the virus isolate was done prior to sequencing, implying that the obtained sequence is the wild-type PUUV sequence. The S segment (accession number OM372469) showed the closest phylogenetic relationship to the Pålböle-strain AM746328.1 based on a comparison of published Swedish S sequences mainly from bank vole (see supplementary Figure 1 for phylogenetic tree of the S segment). An amino acid alignment of the N protein revealed only one amino acid substitution (F413L) between the Pålböle-strain AM746328 and our case isolate (alignment available on request). Only the S segment sequence of the Pålböle-strain, however, is known, why further genomic comparison is not possible. Furthermore, the strain is derived from the bank vole, and therefore the following sequence comparisons refer to human PUUV strains. Compared to five other human isolates and the Swedish reference strain Umea/hu the N-protein harboured a single amino acid substitution, P233A. An alanine residue at position 233 is, however, a common finding among PUUV isolates from bank vole (multiple sequence alignment available on request) and the Vranica strain QOC68546.1 (host species not specified) was even 100% identical to our isolate (see supplementary Table 2). In the alternative ORF of the S segment, encoding the 90 amino acid long NS-protein, four additional amino acid substitutions were identified; D9G, D50E, K60R and N79S, when compared to the Swedish reference strain Umea/hu. A multiple sequence alignment of published NS protein sequences revealed an almost identical match with QOC68547.1 only differing at two locations (K59R and R79S) (multiple sequence alignment available on request).
The M segment (accession number OM372470) contained in total 21 amino acid substitutions compared to Umea/hu (supplemental Table 3). Of these, seven of the mutations remained unique when compared to five other human M sequences (JN831948 and JN831951 derived from the same patient’s lungs and kidneys, respectively; MH251332; MK496160; MK496163). When compared to published sequences not restricted to host species, the highest similarity was found with the Vranica strain QOC68545.1, differing at 15 amino acid positions (supplementary Table 2). Two amino acid substitutions of the case isolate were unique in the sense that they were not to be found in other published isolates, namely position T75 and position V248 (supplementary Table 3).
Discussion
Herein we present a severe case of PUUV infection with a fatal outcome despite an attempt with icatibant-treatment. A fatal outcome is a rare event of PUUV infection, only affecting approximately 0.4% of the patients, and the case-fatality rate increases with age [Citation3]. In addition to advanced age, male sex is a risk factor with a reported male: female ratio for PUUV-caused HFRS cases ranging from 1.52 to 5 according to previous studies [Citation1,Citation3]. Smoking has been reported to be a risk factor to be infected with PUUV [Citation13–15]. It is however, not known if a history of smoking has influenced the course of events in this case.
The patient had low levels of NAbs during the acute infection, which is believed to have contributed to the poor outcome of the infection. In humans, high titres of NAbs correlates with a more favourable outcome for both PUUV and Sin Nombre virus infection [Citation7,Citation16]. In line with the assumption that low levels of NAbs implies a higher risk of severe disease is the high viral titres of this patient indicating a high level of viral replication. PUUV RNA levels the first days after onset of disease have previously been shown to be of the dimension ∼1 × 105 PUUV RNA copies/mL [Citation7]. In the case described herein, however, the viral load in plasma was as high as >2 × 106 PUUV RNA copies/mL when the patient died from the infection. Hantaan virus RNA (vRNA) load has been reported to correlate with disease severity [Citation17], whereas such correlation has not been seen in Swedish NE patients [Citation7]. Taken together, these findings indicate that monoclonal antibodies against orthohantaviruses may be a potential alternative treatment in the future which is supported by a recent study including animal infection models [Citation18].
The autopsy revealed extensive tissue edoema in lungs and bowel, together with effusions in pleura and abdominal viscera, indicating vascular leakage, a key-finding of PUUV pathophysiology. The highest vRNA-levels were seen in the lungs, kidneys and spleen, whereof the two former organs are known to be of importance for PUUV replication [Citation19]. The most prominent biochemical results at day four POF showed significant elevations of serum C-reactive protein, creatinine, liver transaminases, and leucocyte count, in combination with thrombocytopenia, and are all in line with the course of severe PUUV-caused HFRS [Citation20]. In addition, D-dimer and troponin T were also elevated indicating the severity of infection.
Vascular pathology is central in HFRS, and icatibant, a selective bradykinin type 2-receptor antagonist limiting vascular leakage in inflammation, has been suggested beneficiary in severe PUUV infection [Citation9–12]. This, however, could not be documented in the current case despite extensive visceral edoema. The bioavailability of icatibant following subcutaneous administration is nearly 100% and a single dose of 30 mg has previously been used successfully in another case. In the present case, icatibant was administered at day four POF, compared to day eight POF [Citation10], but the patient already suffered from circulatory shock once icatibant was given. It could be that icatibant needs to be administered earlier during infection in order to be effective.
Complete S segment sequencing of the PUUV isolate derived from the patient’s blood plasma, without any previous propagation in cell culture, revealed five amino acid substitutions compared to the Swedish reference strain Umea/hu: P233A in the N protein and D9G, D50E, K59R and N79S in the NS protein. The N protein is the most abundantly expressed PUUV protein and a major antigenic determinant, e.g. the major viral target antigen recognised by T cells [Citation21] and has been shown in in vivo studies to harbour immunogenic domains throughout its length [Citation22]. It has been suggested to influence the severity of endothelial permeability and capillary leakage, and antibodies against the N protein also appear before antibodies against the glycoproteins Gc and Gn [Citation21]. The 90 amino acid long NS-protein encoded by an alternative internal ORF in the S segment is expressed by certain orthohantaviruses including PUUV, and is suggested to be important for down-regulating the interferon response during infection [Citation23]. Whether these four amino acid substitutions of the NS-protein would have had an effect on the interferon response and thereby on the clinical outcome of the patient is unknown. The significance of these amino acid substitutions needs to be further studied in order to address this question.
The translated M sequence of this fatal case was to > 98% identical to two Vranica strains (QOC68545.1 and AAB01661.1) and the Umea/hu strain (AAS19473.1). The high degree of genomic resemblance between Swedish PUUV strains and Vranica strain has been reported previously, in particular the high resemblance between Vranica strain and Hällnäs B1 strain (M32750.1) [Citation24].
Two unique amino acid substitutions were identified (T75 and V248), located in the N terminal of the Gn ectodomain [Citation25]. The viral glycoproteins harbour antigenic sites influencing humoral immune response and mediates cell entry. Interferon transcription is also manipulated by the glycoproteins [Citation26]. Whether the amino acid substitutions identified in this case are of immunological and clinical impact remains to be studied.
In conclusion, we herein describe a severe case of PUUV infection with a notably high level of viremia, low levels of NAbs, and a single dose regime of icatibant, yet resulting in a fatal outcome. Virus sequencing from the patient’s plasma revealed a high degree of homology with S and M segments from lineages from the Northern Scandinavian linage and the Vranica lineage. The clinical impact of the amino acid substitutions identified and their relevance in infection require further investigation. The incoherence between viremia and NAbs might have influenced the fatal outcome of this patient. More studies are needed to address these questions further.
Ethical approval
The study was approved by the Regional Ethics Committee of Umeå University (application numbers 04-37-32 M and 07-162 M). Patients were included after informed consent.
Supplemental Material
Download EPS Image (122.5 KB)Supplemental Material
Download MS Word (52.2 KB)Disclosure statement
No potential conflict of interest was reported by the author(s).
Additional information
Funding
References
- Vapalahti O, Mustonen J, Lundkvist A, et al. Hantavirus infections in Europe. Lancet Infect Dis. 2003;3(10):653–661.
- Laenen L, Vergote V, Calisher CH, et al. Hantaviridae: current classification and future perspectives. Viruses. 2019;11(9):788.
- Hjertqvist M, Klein SL, Ahlm C, et al. Mortality rate patterns for hemorrhagic fever with renal syndrome caused by Puumala virus. Emerg Infect Dis. 2010;16(10):1584–1586.
- Pettersson L, Boman J, Juto P, et al. Outbreak of Puumala virus infection, Sweden. Emerg Infect Dis. 2008;14(5):808–810.
- Johansson P, Olsson M, Lindgren L, et al. Complete gene sequence of a human Puumala hantavirus isolate, Puumala Umeå/hu: sequence comparison and characterisation of encoded gene products. Virus Res. 2004;105(2):147–155.
- Vial PA, Valdivieso F, Calvo M, et al. A non-randomized multicentre trial of human immune plasma for treatment of hantavirus cardiopulmonary syndrome caused by Andes virus. Antivir Ther. 2015;20(4):377–386.
- Pettersson L, Thunberg T, Rocklov J, et al. Viral load and humoral immune response in association with disease severity in Puumala hantavirus-infected patients–implications for treatment. Clin Microbiol Infect. 2014;20(3):235–241.
- Valdivieso F, Vial P, Ferres M, et al. Neutralizing antibodies in survivors of Sin Nombre and Andes hantavirus infection. Emerg Infect Dis. 2006;12(1):166–168.
- Charignon D, Spath P, Martin L, et al. Icatibant, the bradykinin B2 receptor antagonist with target to the interconnected kinin systems. Expert Opin Pharmacother. 2012;13(15):2233–2247.
- Antonen J, Leppanen I, Tenhunen J, et al. A severe case of Puumala hantavirus infection successfully treated with bradykinin receptor antagonist icatibant. Scand J Infect Dis. 2013;45(6):494–496.
- Vaheri A, Strandin T, Jaaskelainen AJ, et al. Pathophysiology of a severe case of Puumala hantavirus infection successfully treated with bradykinin receptor antagonist icatibant. Antiviral Res. 2014;111:23–25.
- Laine O, Leppanen I, Koskela S, et al. Severe Puumala virus infection in a patient with a lymphoproliferative disease treated with icatibant. Infect Dis (Lond). 2015;47(2):107–111.
- Bergstedt Oscarsson K, Brorstad A, Baudin M, et al. Human Puumala hantavirus infection in Northern Sweden; increased seroprevalence and association to risk and health factors. BMC Infect Dis. 2016;16(1):566.
- Vapalahti K, Virtala AM, Vaheri A, et al. Case-control study on Puumala virus infection: smoking is a risk factor. Epidemiol Infect. 2010;138(4):576–584.
- Gherasim A, Hjertqvist M, Lundkvist A, et al. Risk factors and potential preventive measures for nephropatia epidemica in Sweden 2011–2012: a case-control study. Infect Ecol Epidemiol. 2015;5:27698.
- Bharadwaj M, Nofchissey R, Goade D, et al. Humoral immune responses in the hantavirus cardiopulmonary syndrome. J Infect Dis. 2000;182(1):43–48.
- Yi J, Xu Z, Zhuang R, et al. Hantaan virus RNA load in patients having hemorrhagic fever with renal syndrome: correlation with disease severity. J Infect Dis. 2013;207(9):1457–1461.
- Mittler E, Wec AZ, Tynell J, et al. Human antibody recognizing a quaternary epitope in the Puumala virus glycoprotein provides broad protection against orthohantaviruses. Sci Transl Med. 2022;14(636):eabl5399.
- Plyusnina A, Razzauti M, Sironen T, et al. Analysis of complete Puumala virus genome, Finland. Emerg Infect Dis. 2012;18(12):2070–2072.
- Pal E, Korva M, Resman Rus K, et al. Sequential assessment of clinical and laboratory parameters in patients with hemorrhagic fever with renal syndrome. PLoS One. 2018;13(5):e0197661.
- Mir MA. Hantaviruses. Clin Lab Med. 2010;30(1):67–91.
- Lundkvist A, Kallio-Kokko H, Sjolander KB, et al. Characterization of Puumala virus nucleocapsid protein: identification of B-cell epitopes and domains involved in protective immunity. Virology. 1996;216(2):397–406.
- Matthys V, Mackow ER. Hantavirus regulation of type I interferon responses. Adv Virol. 2012;2012:524024.
- Reip A, Haring B, Sibold C, et al. Coding strategy of the S and M genomic segments of a hantavirus representing a new subtype of the Puumala serotype. Arch Virol. 1995;140(11):2011–2026.
- Serris A, Stass R, Bignon EA, et al. The hantavirus surface glycoprotein lattice and its fusion control mechanism. Cell. 2020;183(2):442–456 e16.
- Muyangwa M, Martynova EV, Khaiboullina SF, et al. Hantaviral proteins: structure, functions, and role in hantavirus infection. Front Microbiol. 2015;6:1326.