Abstract
Background
The viral kinetics of SARS-CoV-2 has been considered clinically important. While remdesivir and corticosteroids are recommended for COVID-19 patients requiring oxygen support, there is a limited number of published reports on viral kinetics in hospitalised patients with COVID-19 treated with remdesivir or corticosteroids.
Methods
We conducted a retrospective study by collecting longitudinal samples from the nasopharynx/throat of 123 hospitalised patients (median age 55 years, 74% male) with COVID-19, to evaluate the effects of remdesivir and corticosteroid treatment on viral RNA levels. The subjects were divided into four groups: those receiving remdesivir (n = 25), betamethasone (n = 41), both (n = 15), or neither (n = 42). Time to viral RNA clearance was analysed using Kaplan-Meier plots, categorical data were analysed using Fisher’s exact test, and Kruskal-Wallis for continuous data. Viral RNA decline rate was analysed using a mixed effect model.
Results
We found no significant difference in SARS-CoV-2 RNA decline rate or time to SARS-CoV-2 RNA clearance between the groups. Moreover, clinical status at baseline was not correlated with time to viral clearance.
Conclusions
Since SARS-CoV-2 RNA kinetics was not affected by treatment, repeated sampling from the upper respiratory tract cannot be used to evaluate treatment response.
Introduction
Coronavirus disease 2019 (COVID-19) is an acute respiratory tract infection caused by the Severe Acute Respiratory Syndrome Coronavirus 2 (SARS-CoV-2). The viral kinetics of SARS-CoV-2 have been suggested as clinically important, considering observed correlations between viral load in the nasopharynx and mortality [Citation1], viral load in plasma and mortality [Citation2], and a positive association between sputum viral load, disease severity, and risk of progression [Citation3]. However, other studies found no correlation between viral load and progression [Citation4–6]. Although the number of studies on this topic is steadily increasing, there is a limited number of published studies on viral kinetics in hospitalised patients with COVID-19 treated with remdesivir [Citation7–11], or with corticosteroids [Citation12–15]. Increased knowledge of SARS-CoV-2 ribonucleic acid (RNA) kinetics in COVID-19 is a key to better understanding the transmission, prognosis, and therapy of the disease.
Remdesivir (GS-5734) is an adenosine analogue pro-drug whose active metabolite inhibits viral RNA polymerases with efficacy against Coronaviridae in vitro and in animal models [Citation16–18]. Betamethasone is a synthetic steroid that modifies gene expression by binding to intracellular glucocorticoid receptors, altering the synthesis of pro- and anti-inflammatory proteins, resulting in anti-inflammatory and immunosuppressive effects [Citation19]. Of seven publications studying remdesivir in the treatment of hospitalised patients with COVID-19 [Citation7–9,Citation11,Citation20–22], only two reported reduced mortality [Citation9,Citation20]. The existing evidence for reduced mortality is more robust for corticosteroids [Citation23–25]. Both remdesivir and corticosteroids are recommended for the treatment of patients with COVID-19 who require oxygen support, according to the Infectious Diseases Society of America (IDSA) guidelines [Citation26] and national Swedish guidelines (https://infektion.net/wp-content/uploads/2021/12/nationellt-vardprogram-covid-version-3-4.pdf).
So far two randomised studies have been published, indicating no effect on SARS-CoV-2 RNA clearance with remdesivir treatment compared to placebo [Citation7] or compared to standard of care [Citation8]. In addition, a retrospective observational study also reported no such effect [Citation10]. On the contrary, a large retrospective observational study found a slightly shorter time (9 vs 10 days) to achieve low viral loads (defined as cycle threshold (Ct) ≥ 35). However, time to viral clearance was not significantly different between the two groups [Citation9]. Furthermore, a smaller, retrospective observational study reported significantly steeper increases in the Ct value in the cohort treated with remdesivir [Citation11]. Thus, the effect of remdesivir on SARS-CoV-2 in humans is uncertain and requires further investigation. This is also true for corticosteroids, since existing data on the viral dynamics of SARS-CoV-2 is scarce and inconclusive [Citation23], with conflicting results reporting no effect on viral clearance [Citation12,Citation13], as well as delayed clearance [Citation14,Citation15]. Corticosteroid treatment has previously been associated with delayed CoV RNA clearance in SARS [Citation27] and Middle East Respiratory Syndrome (MERS) [Citation28]. The present study aimed to evaluate the effects of remdesivir and corticosteroid treatment on SARS-CoV-2 RNA kinetics in samples from the upper respiratory tract.
Materials and methods
Study design and participants
A total of 123 patients with COVID-19 verified by Polymerase Chain Reaction (PCR) admitted to the Department of Infectious Diseases, Sahlgrenska University Hospital, Gothenburg, Sweden, from March 1 to December 18, 2020, participated in a prospective sampling study [Citation29] and were retrospectively selected for inclusion in the current study. Participants were divided into four groups, based on treatment regimen: 1) remdesivir (n = 25), 2) remdesivir and betamethasone (n = 15), 3) betamethasone (n = 41), and 4) controls (n = 42). The control group (i.e. patients not receiving remdesivir or betamethasone) was matched for age, sex, and comorbidities and was mainly recruited during the spring of 2020 when neither corticosteroids nor remdesivir was considered the standard of care. Remdesivir was administered intravenously as a 200 mg loading dose on day 1, followed by 100 mg once daily for up to 9 additional days. Betamethasone, 6 mg once daily, was administered orally or intravenously for up to 10 days. The date of disease onset was defined as the first day of self-reported symptoms of COVID-19. All interventions and length of treatment were decided by the treating physician and not influenced by the study design.
Sample collection and laboratory analyses
COVID-19 was diagnosed by PCR at the Department of Clinical Microbiology, Sahlgrenska University Hospital, Gothenburg, Sweden. Serial upper respiratory tract samples were collected in a tube with 1 mL of transport medium. Nucleic acids were extracted from 200 µL of sample in a Magnapure instrument (Roche Molecular, Branchburg, USA), and 10 µL of the purified sample was used for one-step RT-PCR in a QuantStudio 6 real-time PCR instrument. Thirty minutes of reverse transcription were performed at 46 °C with 45 cycles of amplification and 58 °C annealing temperature, using RdRP_F, GTCATGTGTGGCGGTTCACT and RdRP_R, CAACACTATTAGCATAAGCAGTTGT as primers, and RdRP_P, CAGGTGGAACCTCATCAGGAGATGC as a fluorescent hydrolysis probe. This method was complemented with the Cepheid GeneXpert Xpress SARS-CoV-2 assay analysed on a GeneXpert Dx instrument in order to obtain more rapid results when required. Viral loads were expressed as cycle threshold (Ct) values, which are inversely correlated with the concentration of RNA present in the sample. Ct ≥ 40 was considered negative for both methods. Samples were collected on days 1, 3, 6, 10, and 14, then once every 7th day. For practical reasons, the testing schedule was not possible to carry out for all study participants.
Outcomes
The primary endpoints were time to SARS-CoV-2 RNA clearance in the upper respiratory tract from symptom onset and from therapy initiation, and SARS-CoV-2 RNA decline rate measured from therapy initiation. Viral clearance was defined as the time point when SARS-CoV-2 RNA was no longer detectable by PCR (Ct ≥ 40). We also recorded baseline clinical status (i.e. at admission [control group], or at therapy initiation [remdesivir and/or betamethasone]), length of hospitalisation, and 28-day mortality rate. The study was not designed to assess differences in clinical outcomes.
Clinical status was assessed on a 7 point ordinal scale, including 1) death, 2) hospitalisation requiring invasive mechanical ventilation or Extracorporeal Membrane Oxygenation (ECMO), 3) hospitalisation requiring Non-Invasive Ventilation (NIV) or High Flow Nasal Cannula (HFNC), 4) hospitalisation requiring supplemental oxygen, 5) hospitalisation not requiring supplemental oxygen but continued medical care, 6) hospitalisation not requiring supplemental oxygen or continued medical care, 7) not hospitalised. This scale has been used in previous studies on remdesivir in COVID-19 [Citation21,Citation30], and resembles an inversion of the scale suggested by the World Health Organisation (WHO) [Citation31]. Four participants who died were excluded when calculating the mean length of hospitalisation in order not to bias the results.
Statistical analyses
Statistical analyses were performed using GraphPad Prism 8 (GraphPad Software Inc, San Diego, California, USA) and R 4.0.0. SARS-CoV-2 RNA decline rates were derived using a mixed effect model. Time to SARS-CoV-2 RNA clearance was analysed using Kaplan-Meier plots and log-rank tests comparing treatment groups and clinical status groups. Cox regression was used to adjust for baseline Ct values, age, and sex. Categorical data were analysed using Fisher’s exact test, and Kruskal-Wallis for continuous data. The statistical significance level was α = 0.05.
Ethics approval and consent to participate
The study protocol was approved by the Swedish Ethical Review Authority (Dnr: 2020 01771), and written informed consent was obtained from all participants.
Results
Patient characteristics
A total of 91 males (74%) and 32 females (26%), with a median age of 55 years (interquartile range [IQR] 47–63), were included in the study. Median body mass index (BMI) was 29.0 kg/m2 (IQR 26.1–31.9). The most common comorbidities were obesity (41.8%), hypertension (35.8%), and diabetes (14.6%). Overall, 26.8% of the study participants had no registered comorbidity ().
Table 1. Background variables.
Baseline variables
Participants had had median symptom duration of 8.0 days (IQR 7.0–10.0) at admission. The median symptom duration at therapy initiation was 10.0 days (IQR 7.3–12.0) for remdesivir and 9.5 days (IQR 8.0–11.8) for betamethasone. The median first registered Ct value was 25.7 (IQR 21.7–29.4). The median treatment duration was 5.0 days (IQR 5.0–8.0) for remdesivir and 10.0 days (IQR 6.3–10.0) for betamethasone (). At baseline, the clinical status of participants ranged from 2 (hospitalisation requiring invasive mechanical ventilation or ECMO) to 5 (hospitalisation not requiring supplemental oxygen but continued medical care). Mean clinical status at therapy initiation was 3.6 (95% confidence interval [CI] 3.3–3.9) in the remdesivir group, 3.6 (95% CI 3.4–3.8) in the betamethasone group, and 3.4 (95% CI 2.9–3.9) in the group treated with both remdesivir and betamethasone. At admission, the mean clinical status in the control group was 4.1 (95% CI 3.8–4.4), (p = .003) ().
Primary endpoints: viral RNA clearance and rate of decline
The median time from symptom onset to viral RNA clearance was 24 days (95% CI 21.3–26.7) in the control group, 23 days (95% CI 21.2–24.8) in the remdesivir group, 23 days (95% CI 19.7–26.3) in the betamethasone group, and 27 days (95% CI 22.8–31.2) in the group receiving both remdesivir and betamethasone. There were no significant differences between the groups (p = .7) (). There was no significant difference in viral RNA clearance measured as a function of days after the start of treatment (p = .8) (), and no significant difference in the rate of SARS-CoV-2 RNA decline (p = .73) (). The regression lines were derived based on patients with at least 2 Ct values within day 21 after the start of treatment (treatment groups), or after hospital admission (control group). We found an approximately tenfold weekly decline rate in SARS-CoV-2 RNA (roughly equivalent to a three-point increase in Ct value), regardless of treatment (). Clinical status at admission (control group), or at therapy initiation (remdesivir and/or betamethasone) did not correlate with time to viral RNA clearance (p = .5) (), with a median of 21 days (95% CI 16.6–25.4) in the group that did not require supplemental oxygen but continued medical care, 22 days (95% CI 19.6–24.4) in the group requiring supplemental oxygen, 25 days (95% CI 21.1–28.9) in the group requiring NIV or HFNC, and 27 days (95% CI 20.7–33.3) in the group requiring mechanical ventilation or ECMO. All differences remained non-significant after adjusting for baseline Ct values, age, and sex.
Figure 1. The proportion of undetectable SARS-CoV-2 RNA in the upper respiratory tract as a function of days after symptom onset. Longitudinal RT-PCR in 123 hospitalised COVID-19 patients treated with betamethasone (n = 41, red line), remdesivir (n = 25, blue line), both (n = 15, purple line) or neither (n = 42, grey line).
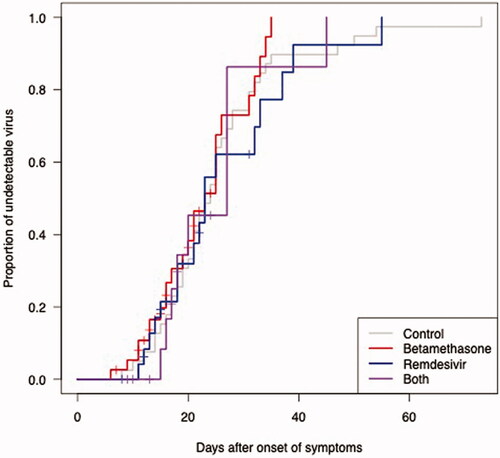
Figure 2. The proportion of undetectable SARS-CoV-2 RNA in the upper respiratory tract as a function of days after the start of treatment. Longitudinal RT-PCR in 81 hospitalised COVID-19 patients treated with betamethasone (n = 41, red line), remdesivir (n = 25, blue line) or both (n = 15, purple line).
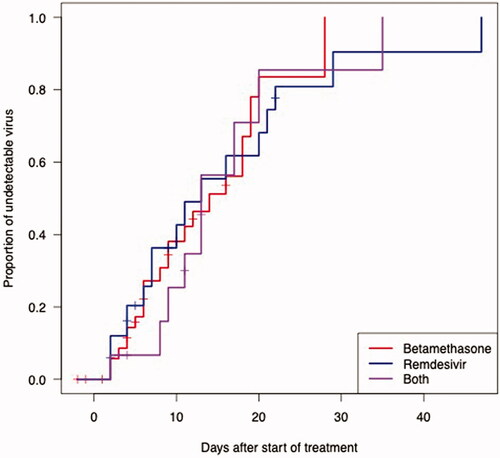
Figure 3. SARS-CoV-2 RNA decline rate. Regression lines are based on subjects with at least 2 data points in the interval 0 to 21 days from the start of treatment (treatment groups), and hospital admission (control group), respectively. Longitudinal RT-PCR in 92 hospitalised COVID-19 patients treated with betamethasone (n = 23), remdesivir (n = 21), both (n = 9) or neither treatment (n = 39).
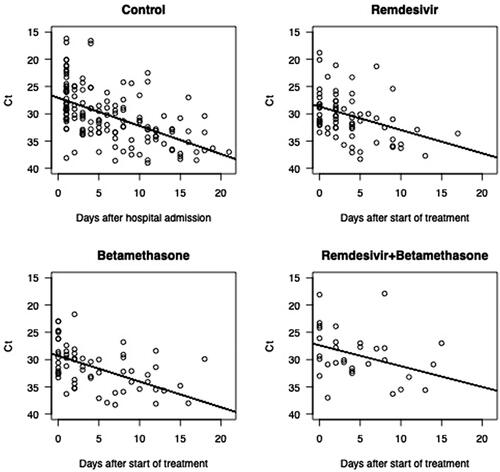
Figure 4. The proportion of undetectable SARS-CoV-2 RNA in the upper respiratory tract as a function of days after symptom onset. Longitudinal RT-PCR in 123 hospitalised COVID-19 patients, divided by baseline clinical status: invasive mechanical ventilation (n = 12, red line), non-invasive ventilation (NIV) or high flow nasal cannula (HFNC) (n = 24, orange line), supplemental oxygen (n = 71, yellow line), or no oxygen, but receiving continued medical care (n = 16, green line).
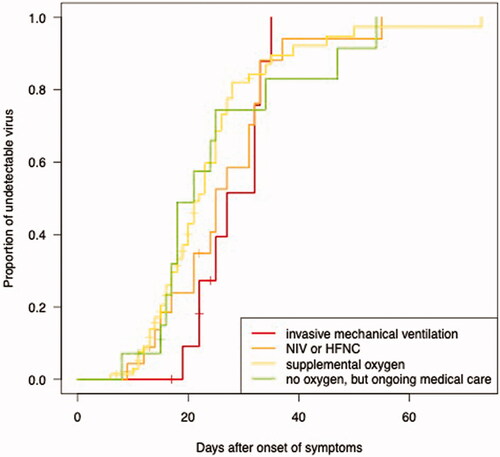
Clinical outcomes: length of hospitalisation and 28-day mortality
Overall, the mean hospital stay was 19.4 (95% CI 14.7–24.7) days. No significant differences between the groups were observed (p = .23). The 28-day mortality rate was 0% in the remdesivir group, 2.4% (n = 1) in the betamethasone group, 2.4% (n = 1) in the control group, and 13.3% (n = 2) in the remdesivir/betamethasone group (p = .16) ().
Table 2. Clinical outcomes.
Discussion
Our study longitudinally compared SARS-CoV-2 RNA levels in the upper respiratory tract of hospitalised patients with COVID-19. We found no effect of treatment with remdesivir, betamethasone, or both regarding SARS-CoV-2 RNA decline rate or time to SARS-CoV-2 RNA clearance. Moreover, the baseline clinical status of patients did not correlate with time to viral RNA clearance. Recent clinical studies have shown improvements in clinical status in hospitalised patients with COVID-19 after treatment with remdesivir [Citation9,Citation11,Citation20,Citation21,Citation32]. Considering this and the potential ability of remdesivir to inhibit SARS-CoV-2 RNA polymerases in humans [Citation33], one would expect a shorter duration of viral RNA shedding in patients receiving remdesivir. However, we found no effect on viral RNA clearance with remdesivir treatment. This is in agreement with most previous reports [Citation7–9]. There are possible explanations for this lack of effects such as a misrepresentative sample site (upper respiratory tract rather than lower respiratory tract) and an alternative mechanism of action of remdesivir. However, the most likely explanation is that the drug must be administered early after symptom onset to be effective. This is supported by the fact that the highest viral loads occur during the first days of symptoms [Citation4,Citation5,Citation34], indicating better treatment potential with early therapy initiation. In the current study, remdesivir was started at median of 10 days after symptom onset, which may be too late. This might also explain the modest clinical outcomes reported in several studies, where remdesivir treatment was initiated on a median day 9 to 10 after symptom onset [Citation7,Citation20,Citation21]. In two studies, remdesivir treatment was initiated earlier, and beneficial effects on clinical outcomes [Citation9], and SARS-CoV-2 RNA decline [Citation11] were reported. Patients with severe COVID-19 do not usually require hospitalisation before day 7 [Citation35], leaving only a small window of opportunity for the efficacy of remdesivir.
In our study, betamethasone did not prolong viral RNA shedding, in contrast to SARS [Citation27], MERS [Citation28], and influenza [Citation36], although some prior reports suggested delayed viral RNA clearance in COVID-19 [Citation15,Citation23]. The approaches to corticosteroid treatment have, however, diverged with regard to choice of steroid, dose, duration of therapy, and timing [Citation23], making it difficult to draw firm conclusions. Further research is needed.
Our finding of no difference in viral RNA clearance both between treatment groups and clinical status could reflect that the replicative phase of the disease has ended by the time most immunocompetent patients are admitted to the hospital. This is supported by the fact that no viable virus has been detected in the upper respiratory tract beyond day 9 of illness, despite persistently detectable viral loads [Citation37].
The significant difference in baseline clinical status between the groups, with less severe disease in the control group, may be due to the clinical assessment being done at different time points, i.e. at admission in the control group but at therapy initiation in the treatment groups. However, in agreement with other studies [Citation4–6], we noted no association between disease severity and time to viral RNA clearance. Thus, it is unlikely that the difference between groups might have affected the results.
The main limitations of the present study are its retrospective nature, its non-randomised design with the risk of channelling bias, the differences in the number of subjects and the distribution of clinical status, and the relatively small number of subjects. A larger number of participants would be preferable to resolve the inconsistency of the current evidence on the effects of remdesivir and corticosteroid treatment on SARS-CoV-2 RNA kinetics. However, our study is of a similar size compared to most other studies addressing this issue [Citation7–11], and we believe that our results are valid and that they contribute to the aggregated knowledge.
Since samples were not collected daily, there is uncertainty as to baseline Ct value at therapy initiation (remdesivir and/or betamethasone), at admission (control group), and regarding the time point of viral RNA clearance. However, this is unlikely to affect our conclusion since, in addition to no difference in the viral RNA clearance, there was no difference in the viral RNA decline rate.
Today the IDSA not only recommends remdesivir for patients suffering from a severe disease (defined as those with SpO2 ≤ 94% on room air, and those who require supplemental oxygen, mechanical ventilation, or ECMO), but also suggests remdesivir for mild to moderate COVID-19 patients at high risk of progression to severe disease. For this indication, a shorter course of three days is recommended. However, it is unlikely that a shorter treatment course would affect SARS-CoV-2 decline and clearance when a longer course does not. We primarily included severely ill patients; SARS-CoV-2 kinetics might behave differently in patients with mild disease, however, we found no correlation between baseline clinical status and SARS-CoV-2 RNA clearance. Finally, it should be pointed out that Ct is a semi-quantitative indicator. Also, neither corticosteroids nor remdesivir was standard of care when most patients in the control group were recruited.
Conclusions
We found no evidence that either remdesivir or betamethasone had an effect on SARS-CoV-2 RNA decline rate or clearance in the upper respiratory tract in hospitalised patients with COVID-19. Hence, repeated sampling cannot be used to evaluate treatment response. In addition, there was no correlation between baseline clinical status and SARS-CoV-2 RNA clearance, suggesting that disease severity does not influence the time of SARS-COV-2 RNA shedding.
Author contributions
BS and MG were responsible for the design of the study, as well as the acquisition of data. BS, MG, MB, NS, EM and AY recruited the participants. SN, MG, and BS performed the statistical analyses. ML performed the SARS-CoV-2 PCR analyses. All authors participated in drafting the manuscript and approved the final version. Corresponding author information on title page.
Disclosure statement
MG has received research grants from Gilead Sciences and Janssen-Cilag and honoraria as a speaker or scientific advisor from Amgen, Biogen, Bristol-Myers Squibb, Gilead Sciences, GlaxoSmithKline/ViiV, Janssen-Cilag, MSD, Novocure, and Novo Nordic.
Data availability statement
The dataset analysed during the current study is available from the corresponding author on reasonable request.
Additional information
Funding
References
- Neant N, Lingas G, Le Hingrat Q, et al. Modeling SARS-CoV-2 viral kinetics and association with mortality in hospitalized patients from the French COVID cohort. Proc Natl Acad Sci USA. 2021;118(8):e2017962118.
- Prebensen C, Myhre PL, Jonassen C, et al. SARS-CoV-2 RNA in plasma is associated with ICU admission and mortality in patients hospitalized with COVID-19. Clin Infect Dis. 2020;73(3):e799–e802.
- Yu X, Sun S, Shi Y, et al. SARS-CoV-2 viral load in sputum correlates with risk of COVID-19 progression. Crit Care. 2020;24(1):170.
- Yilmaz A, Marklund E, Andersson M, et al. Upper respiratory tract levels of severe acute respiratory syndrome coronavirus 2 RNA and duration of viral RNA shedding do not differ between patients with mild and severe/critical coronavirus disease 2019. J Infect Dis. 2021;223(1):15–18.
- Zou L, Ruan F, Huang M, et al. SARS-CoV-2 viral load in upper respiratory specimens of infected patients. N Engl J Med. 2020;382(12):1177–1179.
- Lui G, Ling L, Lai CK, et al. Viral dynamics of SARS-CoV-2 across a spectrum of disease severity in COVID-19. J Infect. 2020;81(2):318–356.
- Wang Y, Zhang D, Du G, et al. Remdesivir in adults with severe COVID-19: a randomised, double-blind, placebo-controlled, multicentre trial. Lancet. 2020;395(10236):1569–1578.
- Barratt-Due A, Olsen IC, Nezvalova-Henriksen K, et al. Evaluation of the effects of remdesivir and hydroxychloroquine on viral clearance in COVID-19: a randomized trial. Ann Intern Med. 2021;174(9):1261–1269.
- Wong CKH, Lau KTK, Au ICH, et al. Clinical improvement, outcomes, antiviral activity, and costs associated with early treatment with remdesivir for patients with COVID-19. Clin Infect Dis. 2021;74(8):1450–1458.
- Goldberg E, Ben Zvi H, Sheena L, et al. A real-life setting evaluation of the effect of remdesivir on viral load in COVID-19 patients admitted to a large tertiary centre in Israel. Clin Microbiol Infect. 2021;27(6):917 e1–e4.
- Joo EJ, Ko JH, Kim SE, et al. Clinical and virologic effectiveness of remdesivir treatment for severe coronavirus disease 2019 (COVID-19) in Korea: a nationwide multicenter retrospective cohort study. J Korean Med Sci. 2021;36(11):e83.
- Muhlemann B, Thibeault C, Hillus D, et al. Impact of dexamethasone on SARS-CoV-2 concentration kinetics and antibody response in hospitalized COVID-19 patients: results from a prospective observational study. Clin Microbiol Infect. 2021;27(10):1520 e7–e10.
- Masia M, Fernandez-Gonzalez M, Garcia JA, et al. Lack of detrimental effect of corticosteroids on antibody responses to SARS-CoV-2 and viral clearance in patients hospitalized with COVID-19. J Infect. 2021;82(3):414–451.
- Huang R, Zhu C, Jian W, et al. Corticosteroid therapy is associated with the delay of SARS-CoV-2 clearance in COVID-19 patients. Eur J Pharmacol. 2020;889:173556.
- Li J, Liao X, Zhou Y, et al. Association between glucocorticoids treatment and viral clearance delay in patients with COVID-19: a systematic review and meta-analysis. BMC Infect Dis. 2021;21(1):1063.
- Sheahan TP, Sims AC, Graham RL, et al. Broad-spectrum antiviral GS-5734 inhibits both epidemic and zoonotic coronaviruses. Sci Transl Med. 2017;9(396):eaal3653.
- Pizzorno A, Padey B, Dubois J, et al. In vitro evaluation of antiviral activity of single and combined repurposable drugs against SARS-CoV-2. Antiviral Res. 2020;181:104878.
- Williamson BN, Feldmann F, Schwarz B, et al. Clinical benefit of remdesivir in rhesus macaques infected with SARS-CoV-2. Nature. 2020;585(7824):273–276.
- Williams DM. Clinical pharmacology of corticosteroids. Respir Care. 2018;63(6):655–670.
- Beigel JH, Tomashek KM, Dodd LE, et al. Remdesivir for the treatment of covid-19 – final report. N Engl J Med. 2020;383(19):1813–1826.
- Spinner CD, Gottlieb RL, Criner GJ, et al. Effect of remdesivir vs standard care on clinical status at 11 days in patients with moderate COVID-19: a randomized clinical trial. JAMA. 2020;324(11):1048–1057.
- Pan H, Peto R, Henao-Restrepo AM, et al. Repurposed antiviral drugs for covid-19 – interim WHO solidarity trial results. N Engl J Med. 2021;384(6):497–511.
- van Paassen J, Vos JS, Hoekstra EM, et al. Corticosteroid use in COVID-19 patients: a systematic review and meta-analysis on clinical outcomes. Crit Care. 2020;24(1):696.
- Group RC, Horby P, Lim WS, et al. Dexamethasone in hospitalized patients with covid-19. N Engl J Med. 2021;384(8):693–704.
- Monedero P, Gea A, Castro P, et al. Early corticosteroids are associated with lower mortality in critically ill patients with COVID-19: a cohort study. Crit Care. 2021;25(1):2.
- Bhimraj A, Morgan RL, Shumaker AH, et al. Infectious diseases society of America guidelines on the treatment and management of patients with COVID-19. Clin Infect Dis. 2020:ciaa478.
- Stockman LJ, Bellamy R, Garner P. SARS: systematic review of treatment effects. PLOS Med. 2006;3(9):e343.
- Arabi YM, Mandourah Y, Al-Hameed F, et al. Corticosteroid therapy for critically ill patients with middle east respiratory syndrome. Am J Respir Crit Care Med. 2018;197(6):757–767.
- Marklund E, Leach S, Axelsson H, et al. Serum-IgG responses to SARS-CoV-2 after mild and severe COVID-19 infection and analysis of IgG non-responders. PLOS One. 2020;15(10):e0241104.
- Goldman JD, Lye DCB, Hui DS, et al. Remdesivir for 5 or 10 days in patients with severe covid-19. N Engl J Med. 2020;383(19):1827–1837.
- Organization WH. COVID-19 Therapeutic Trial Synopsis 2020. [updated February 18. Available from: http://www.who.int/publications-detail/covid-19-therapeutic-trial-synopsis.
- Garibaldi BT, Wang K, Robinson ML, et al. Comparison of time to clinical improvement with vs without remdesivir treatment in hospitalized patients with COVID-19. JAMA Netw Open. 2021;4(3):e213071.
- Pruijssers AJ, George AS, Schafer A, et al. Remdesivir inhibits SARS-CoV-2 in human lung cells and chimeric SARS-CoV expressing the SARS-CoV-2 RNA polymerase in mice. Cell Rep. 2020;32(3):107940.
- To KK, Tsang OT, Leung WS, et al. Temporal profiles of viral load in posterior oropharyngeal saliva samples and serum antibody responses during infection by SARS-CoV-2: an observational cohort study. Lancet Infect Dis. 2020;20(5):565–574.
- Huang C, Wang Y, Li X, et al. Clinical features of patients infected with 2019 novel coronavirus in Wuhan, China. Lancet. 2020;395(10223):497–506.
- Lee N, Chan PK, Hui DS, et al. Viral loads and duration of viral shedding in adult patients hospitalized with influenza. J Infect Dis. 2009;200(4):492–500.
- Cevik M, Tate M, Lloyd O, et al. SARS-CoV-2, SARS-CoV, and MERS-CoV viral load dynamics, duration of viral shedding, and infectiousness: a systematic review and meta-analysis. Lancet Microbe. 2021;2(1):e13–e22.