Abstract
Background
Bacterial infections are associated with acute exacerbations of chronic obstructive pulmonary disease (AECOPD), but the mechanism is incompletely understood.
Method
In a COPD observational study (NCT01360398), sputum samples were collected monthly at the stable state and exacerbation. Post-hoc analyses of 1307 non-typeable Haemophilus influenzae (NTHi) isolates from 20 patients and 756 Moraxella catarrhalis isolates from 38 patients in one year of follow-up were conducted by multilocus sequence typing (MLST). All isolates came from cultured sputum samples that were analyzed for bacterial species presence, apparition (infection not detected at the preceding visit), or acquisition (first-time infection), with the first study visit as a baseline. Strain apparition or new strain acquisition was analyzed by MLST. The odds ratio (OR) of experiencing an exacerbation vs. stable state was estimated by conditional logistic regression modelling, stratified by patient.
Results
The culture results confirmed a significant association with exacerbation only for NTHi species presence (OR 2.28; 95% confidence interval [CI]: 1.12–4.64) and strain apparition (OR 2.38; 95% CI: 1.08–5.27). For M. catarrhalis, although confidence intervals overlapped, the association with exacerbation for first-time species acquisition (OR 5.99; 2.75–13.02) appeared stronger than species presence (OR 3.67; 2.10–6.40), new strain acquisition (OR 2.94; 1.43–6.04), species apparition (OR 4.18; 2.29–7.63), and strain apparition (OR 2.78; 1.42–5.42). This may suggest that previous M. catarrhalis colonization may modify the risk of exacerbation associated with M. catarrhalis infection.
Conclusions
The results confirm that NTHi and M. catarrhalis infections are associated with AECOPD but suggest different dynamic mechanisms in triggering exacerbations.
Introduction
Infection with bacterial pathogens is an important risk factor for acute exacerbations of chronic obstructive pulmonary disease (AECOPD) [Citation1]. Exacerbation events are often associated with a shift in microbiome composition in terms of both chronic colonization and bacterial acquisition in the lower airway [Citation2–6]. However, the mechanism for this association is incompletely understood.
In the observational study, Acute Exacerbation and Respiratory InfectionS in COPD (AERIS), patients with COPD were followed for up to 2 years [Citation7,Citation8]. The most prevalent potentially pathogenic bacterial species identified from sputum samples were non-typeable Haemophilus influenzae (NTHi), Moraxella catarrhalis, and Streptococcus pneumoniae at stable state and exacerbation. H. influenzae and M. catarrhalis identification was associated with an increased risk of exacerbation [Citation7], consistent with other studies demonstrating changes in the prevalence of these airway bacteria with exacerbation [Citation2,Citation4,Citation9–16]. Additionally, the odds ratio of being in an exacerbation rather than a stable state was significant for NTHi or M. catarrhalis detection after a negative sputum sample at the previous visit (apparition) [Citation7]. An association between exacerbation and isolation of a new strain of H. influenzae or M. catarrhalis was first described in 2002 [Citation6]. A more recent sputum sampling study of COPD patients found that frequent exacerbators are more likely to be infected with Haemophilus and Moraxella than infrequent exacerbators [Citation3]. These observations suggest a major role for bacterial acquisition in the respiratory dysbiosis associated with AECOPD.
We conducted post-hoc analyses of sputum samples collected longitudinally during the AERIS study to examine the mechanism of bacterial strain acquisition as a trigger of exacerbation among patients with COPD. Culture-based assays were used to detect the presence of NTHi and M. catarrhalis at stable-state COPD and exacerbation, distinguishing between acquisition (detection for the first time during the course of the study) and apparition (presence not detected at the preceding study visit but may have been detected at some point before the preceding visit). To characterize infections further according to bacterial strain, whole genome sequencing (WGS) and multilocus sequence typing (MLST) were used to genotype NTHi and M. catarrhalis strains. These MLST data were used to determine the occurrence of new strain acquisition and strain apparition. While no firm conclusions can be drawn from the results of these post-hoc analyses, these initial investigations of the association with exacerbation events point towards a link between NTHi species presence or new strain apparition and AECOPD. The results also indicate that previous colonization with M. catarrhalis may modify the exacerbation risk associated with the detection of a new M. catarrhalis strain.
Methods
Study design
Sputum samples were obtained by spontaneous expectoration or induced from patients with COPD participating in the prospective, observational AERIS study conducted at University Hospital Southampton, UK (ClinicalTrials.gov registration, NCT01360398). The study methods were described previously [Citation7,Citation8,Citation17]. Briefly, patients aged 40–85 years with a confirmed diagnosis of moderate, severe, or very severe COPD [Citation17,Citation18] were followed for 24 months, undergoing sputum sampling monthly in the stable state and at exacerbation for the detection of bacteria and viruses. All patients were reviewed within 72 h of onset of AECOPD symptoms detected using daily electronic diary cards. As described previously [Citation7], AECOPD was defined as worsening of at least two major symptoms (dyspnea, sputum volume, and sputum purulence) or worsening of at least one major symptom and one minor symptom (wheeze, sore throat, cold symptoms, cough, and fever without other cause) on at least two consecutive days. AERIS was conducted in accordance with the Declaration of Helsinki and Good Clinical Practice and was approved by the Southampton and South West Hampshire Research Ethics Committee (Research Ethics Committee reference number: 11/H0502/9). All patients provided written informed consent.
Incidences of bacterial and viral pathogens and eosinophilic inflammation in AECOPD and stable state have already been reported from the AERIS study [Citation7,Citation8,Citation19–22]. In this post-hoc analysis, we focussed on the potentially pathogenic bacterial species that were most prevalent in COPD patients: NTHi and M. catarrhalis. NTHi results are presented for sputum samples taken from a sub-cohort of 36 patients in the first year of follow-up, who were the first patients to be enrolled in the study and with sputum samples available; the characteristics of this sub-cohort were similar to those of the 127 patients in the original full cohort (Supplementary Table S1) [Citation7]. For M. catarrhalis, few positive timepoints were identified in the same sub-cohort. Results are therefore presented for both the sub-cohort (see Supplementary Table S2) and for full cohort patients with sputum samples available (122 patients) in the first year of follow-up [Citation7].
Sputum sample processing
Fresh sputum samples were cultured in accordance with Public Health England’s UK Standards for Microbiology Investigations, as described previously [Citation7]. Sputum samples initially identified by culture as containing H. influenzae were retested using a molecular approach developed by GSK, the lgtC/P6 duplex real-time Polymerase Chain Reaction (PCR), which targets the glycosyltransferase encoding gene (lgtC) and the outer membrane protein P6 encoding gene (omp P6) [Citation23]. H. influenzae species identification by assessing the presence of both lgtC and omp P6 previously showed an agreement of 96.61% against ‘gold standard’ multigene phylogenetic trees [Citation24,Citation25]. An absence of H. influenzae was assumed to indicate H. haemolyticus presence, based on previous observations published by Murphy et al. [Citation26], and as confirmed by WGS [Citation21].
Genetic analyses of bacterial isolates
From the cultured samples, NTHi and M. catarrhalis colonies (∼10 for each species per timepoint) were analyzed by WGS and MLST. Nucleic acids were extracted from NTHi isolates using the QIAamp DNA Mini Kit (QIAGEN, Manchester, UK) and from M. catarrhalis isolates using the MagNA Pure 96 DNA and Viral NA Small Volume Kit (Roche Diagnostics, Risch-Rotkreuz, Switzerland), as per the manufacturers’ instructions. Genomic DNA from the isolates was purified further with AMPure XP Beads (Beckman Coulter, CA, USA) before being processed for library generation using the Nextera XT (Illumina, CA, USA), following the manufacturer’s recommendations and using 0.2 ng/µL of genomic DNA and 15 PCR cycles (12 cycles for NTHi).
Sequencing was performed on a MiSeq instrument (Illumina, CA, USA) using the MiSeq Reagent V2 kit according to the manufacturer’s recommendations for paired-end sequencing (2 × 250 cycles) with a target of minimum 30 × mapping coverage of MLST genes. adk, pgi, fucK, atpG, frdB, mdh, and recA genes were used for H. influenzae and glyRS, ppa, efp, fumC, tprE, mutY, adk, and abcZ for M. catarrhalis. MLST and identification of genes for speciation were performed using SRST2 (version 0.1.5) [Citation27] on WGS data from each isolate. Here, paired-end FASTQs were mapped to reference sequences [Citation28]. All parameters were used by default except for minimum coverage (minimum percentage coverage cut-off for gene reporting was, by default, 90%). To ensure the presence of genes (complete, partial, or recombinant sequences), a minimum coverage cut-off of 60% was used.
Statistical analysis
The odds of experiencing an exacerbation rather than being in a stable state were estimated by a post-hoc conditional logistic regression model, stratified by the patient. This examined the effect of the presence of a bacterial species identified by culture, acquisition, or apparition of a bacterial species identified by culture, or acquisition or apparition of a bacterial strain identified by MLST on the likelihood of exacerbation. Using infection status at the first study visit as a baseline, the bacterial acquisition was defined as an infection or strain detected for the first time in the patient during the course of the study, and the bacterial apparition was defined as an infection or strain that was detected after a negative sputum sample from the patient at the preceding study visit. For apparition, the bacterial species or strain may have been detected at a visit before the preceding visit and the patient’s COPD status (stable or exacerbation) at the preceding study visit was not relevant.
H. influenzae and M. catarrhalis, each assessed as bacterial species presence, acquisition, or apparition and new strain acquisition or strain apparition, were included in the model to assess their association with AECOPD. For bacterial species or bacterial strain acquisition and apparition, if presence was not evaluated at the preceding study visit (for example, because no sputum sample was collected), the last value observed before this visit was used.
The seasonal effect was assessed by dividing the seasons into two: the ‘cold season’ (October to February) and ‘warm season’ (March to September). The first model we assessed included the bacterial species, the season, and the interaction term between the bacterium (NTHi or M. catarrhalis) and the season. If the interaction term was not significant (significance level set at α = 0.10), it was removed from the model. Thus, the second model that was assessed did not include the interaction term but included the season as a covariate (significance level set at α = 0.05). If the season was not significant, it was excluded from the model and the model without adjustment for the season was selected. Since the interaction term between bacteria and season was not significant in any of the models but the season as a covariate was significant in all models, the final models were adjusted for the season.
Statistical analysis was performed using the SAS Drug Development platform version 4.3.2 (SAS Institute, Cary, NC, USA).
Results
Sample processing and bacterial identification patterns
Out of 36 patients in the sub-cohort, 20 were positive by culture for NTHi at one timepoint or more (nine enrolment visits, 73 stable visits, and 45 exacerbation visits) (). A total of 1307 NTHi isolates were processed, with MLST data obtained for 1222 isolates. Some NTHi (and M. catarrhalis) isolates could not be re-cultured for nucleic acid extraction or MLST data could not be obtained due to mixed or unreadable sequences, suggesting a mix of strains. Thirty-seven different MLSTs were obtained; nine were not previously described and one (composed of 56 NTHi isolates from a single patient) could not be assigned due to the absence of the fucK gene. Five types were shared between two patients and one was identified in five different patients. Only 7.7% (10 of 129) analyzed sputum samples showed co-colonization of two or more different NTHi strains. Taking infection status at the first study visit as a baseline, of the 20 patients with NTHi MLST data available, 17 had NTHi strain acquisition (first detection in patient) and 18 had NTHi strain apparition (detection after negative sputum sample at the preceding visit) at one or more timepoint.
Table 1. The number of patients and study timepoints for which sputum samples were positive for Haemophilus influenzae (NTHi) or Moraxella catarrhalis presence, acquisition, or apparition by culture, or acquisition or apparition of a strain identified by MLST (NTHi, sub-cohort; M. catarrhalis, full cohort).
Of the 122 patients with sputum samples available in the full cohort, 46 were positive by culture for M. catarrhalis at one timepoint or more (eight enrolment visits, 42 stable visits, and 40 exacerbation visits) (). A total of 761 M. catarrhalis isolates were processed and MLST data were obtained for 756 isolates. Forty-seven different MLSTs were identified, with 31 not previously described. Twelve MLSTs were shared between two patients and one was detected in four patients. Two M. catarrhalis strains in the same sputum sample were detected in 2.2% (two of 90) samples. One patient was excluded from the MLST analyses of acquisition and apparition because only one sputum sample was available. Of 121 patients considered for the analyses, 38 had M. catarrhalis MLST data available. Acquisition of an M. catarrhalis strain was detected in 30 patients and apparition of an M. catarrhalis strain was detected in 35 patients at one timepoint or more.
shows the number of patients and study visit timepoints for which the presence, acquisition, or apparition of NTHi or M. catarrhalis was observed.
Longitudinal profiling of strain colonization
Longitudinal analysis of NTHi strain colonization in the sub-cohort and M. catarrhalis strain colonization in the full cohort show different patterns for the two pathogens (). MLST data were available for 20 NTHi-positive patients and 38 M. catarrhalis-positive patients. Most NTHi-colonized patients displayed sustained colonization during the year of follow-up, with six out of 20 (30.0%) colonized by NTHi at 10 visits or more and only three (15.0%) colonized at a single visit. During the study, most (15; 75.0%) patients were colonized by more than one NTHi strain (at least one new strain acquisition), while one patient was colonized by the same strain at 10 visits. In contrast, 18 out of 38 (47.4%) M. catarrhalis-positive patients were colonized at a single visit. The remaining patients were either colonized at different visits by different M. catarrhalis strains (14 patients; 36.8%) or were colonized by the same strain at more than one visit (six patients; 15.8%). The average number of visits per strain in the same patient was 1.3 for M. catarrhalis vs. 3.3 for NTHi.
Figure 1. Longitudinal analysis of non-typeable Haemophilus influenzae (NTHi) strain colonization in the sub-cohort and Moraxella catarrhalis strain colonization in the full cohort. Data is available from 20 NTHi-positive patients and 38 M. catarrhalis-positive patients. *Exacerbation. Strain changes in each patient indicated by successive changes in circle fill colour:
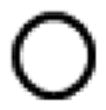
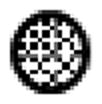
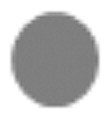
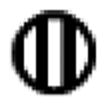
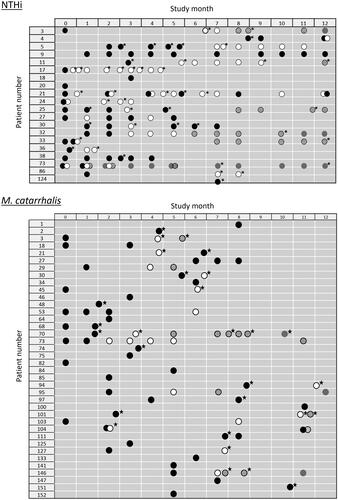
Association with exacerbation: NTHi
A significant association with exacerbation (compared to a stable state) was observed for NTHi presence by culture (odds ratio 2.28; 95% confidence interval [CI]: 1.12–4.64) (). Nine cases of NTHi species acquisition, 27 cases of NTHi species apparition, and 31 cases of acquisition of a new strain of NTHi were detected (); none of these events had a significant association with exacerbation vs. stable state (). Fifty-one events of NTHi strain apparition (strain not detected at the preceding visit but may have occurred at an earlier visit) were detected with a significant association with exacerbation vs. stable state (odds ratio 2.38; 95% CI: 1.08–5.27) ().
Figure 2. Effect of non-typeable Haemophilus influenzae (NTHi) presence, acquisition, or apparition by culture, or acquisition or apparition of a strain identified by MLST on the odds of being in exacerbation vs. stable state (sub-cohort of 36 patients). Odds ratio results from case-control analyses performed on sputum culture results, using a logistic model stratified by subject, adjusted for the season. MLST: multilocus sequence typing; NS: not significant; 95% CI: 95% confidence interval.
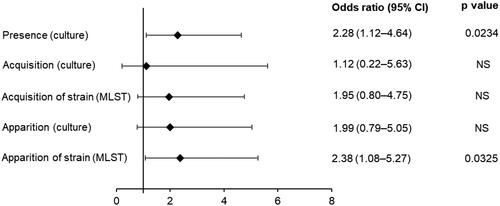
Association with exacerbation: M. catarrhalis
A significant association between exacerbation vs. stable state was observed when M. catarrhalis was present by culture (odds ratio 3.67; 95% CI: 2.10–6.40) (). Significant associations with exacerbation were also detected for the acquisition and apparition of M. catarrhalis infection, as well as for M. catarrhalis new strain acquisition and strain apparition (). Contrary to what was observed for NTHi (), the odds of being in exacerbation vs. stable state tended to be higher for M. catarrhalis species acquisition (5.99; 95% CI: 2.75–13.02) than for species apparition (4.18; 95% CI: 2.29–7.63), M. catarrhalis strain acquisition (2.94; 95% CI: 1.43–6.04), and strain apparition (2.78; 95% CI: 1.42–5.42).
Figure 3. Effect of Moraxella catarrhalis presence, acquisition, or apparition by culture, or acquisition or apparition of a strain identified by MLST on the odds of being in exacerbation vs. stable state (full cohort of 122 [culture] or 121 [MLST] patients). Odds ratio results from case-control analyses performed on sputum culture results, using a logistic model stratified by subject, adjusted for the season. MLST: multilocus sequence typing; 95% CI: 95% confidence interval.
![Figure 3. Effect of Moraxella catarrhalis presence, acquisition, or apparition by culture, or acquisition or apparition of a strain identified by MLST on the odds of being in exacerbation vs. stable state (full cohort of 122 [culture] or 121 [MLST] patients). Odds ratio results from case-control analyses performed on sputum culture results, using a logistic model stratified by subject, adjusted for the season. MLST: multilocus sequence typing; 95% CI: 95% confidence interval.](/cms/asset/62a90086-bec1-4711-a042-d848164678b1/infd_a_2092648_f0003_b.jpg)
In the sub-cohort (see Supplementary Table S2), we observed only 10, 16, 11, and 13 events of M. catarrhalis acquisition, apparition, strain acquisition, and strain apparition, respectively. The odds of being in exacerbation associated with M. catarrhalis were in line with those for the full cohort for species acquisition and apparition, and the odds ratios were similar, but non-significant in the sub-cohort, for strain acquisition and apparition. However, the sub-cohort results are less robust due to the much smaller sample size (see Methods).
Discussion
These results confirm there is an association between NTHi and M. catarrhalis respiratory tract infections and the risk of exacerbation, as reported previously from the AERIS study [Citation7]. Both pathogens stimulate several neutrophil-related components, which are likely to enhance airway inflammation and contribute to COPD exacerbations and progression [Citation29,Citation30]. The mechanism for this association may be complex and our observations suggest it is different for NTHi and M. catarrhalis.
In this analysis, we used MLST to characterize infections detected by culture according to bacterial strain. This allowed us to differentiate between a strain that was not present at the previous study visit but may have been present at an earlier visit (strain apparition) and the acquisition of a new strain. For NTHi, the likelihood of experiencing an exacerbation in patients with strain apparition was 2.4 times higher than in patients without NTHi strain apparition. In our analysis, new NTHi strain acquisition detected by MLST and NTHi species acquisition and apparition detected by culture had no significant association with exacerbation. The number of samples with the acquisition was small due to chronic NTHi colonization in many patients. NTHi often colonizes the lungs of COPD patients, becoming a component of the microbiota due to its ability to persist in the airways and evade host immune recognition and clearance mechanisms [Citation31]. This may explain the discrepancy with results from previous studies which reported a significant association between NTHi acquisition and exacerbation [Citation6,Citation26]. However, if confirmed, our observations suggest that a change in NTHi strain between subsequent visits is associated with exacerbation. An exacerbation associated with NTHi may therefore be triggered by a change in NTHi antigen exposure, perhaps due to altered bacterial load or the presence of different antigen molecules.
We observed a significant association with exacerbation compared to stable state for new strain acquisition and strain apparition detected by MLST and for the presence, acquisition, and apparition of M. catarrhalis species detected by culture, which is in line with findings from other studies [Citation3,Citation4,Citation6,Citation13,Citation16]. Since M. catarrhalis infections tend to display greater temporal variability than NTHi infections in patients with COPD, acquisition, and apparition of M. catarrhalis could be expected to occur more frequently. This was the case in our study (), although different cohort sizes were assessed. Longitudinal analysis showed most NTHi-colonized patients had sustained colonization during the year of follow-up, while almost half of M. catarrhalis-positive patients were colonized at a single visit, suggesting M. catarrhalis has a more acute pattern of infection than NTHi.
The highest odds of being in exacerbation was for M. catarrhalis species acquisition, as detected by culture, with odds six times higher than in patients without M. catarrhalis acquisition. M. catarrhalis strain acquisition detected by MLST was also associated with increased odds of being in exacerbation, albeit to a lesser extent (nearly three times higher than in those without M. catarrhalis strain acquisition). These observations warrant a long-term investigation of infection patterns and evaluation of the possible role of immunity to conserved M. catarrhalis antigens or specific M. catarrhalis strains in reducing the risk of exacerbation in patients with COPD.
The model used in this study does not take into account possible correlations between successive measures within each subject. However, tests of the fit of generalized estimating equations and generalized linear mixed models with the same logit link indicated that models assuming independence between observations within each subject provided a fit similar to those taking correlations into account. By stratifying by subject, the conditional logistic model has the added advantage of considering any confounding factors that remain constant over time (such as age, gender, and COPD status) for each subject. Moreover, previous results from the AERIS study demonstrated a seasonal peak in exacerbations that was associated with a combination of higher incidence of seasonal pathogens, a seasonal interaction between NTHi and viral infection, and greater bacterial loads [Citation7]. Our modelling analyses confirmed the important role played by the season in terms of its association with AECOPD and bacterial infection (see Methods). The season was shown to be a confounder in the association between AECOPD and bacterial infection and was adjusted for in the statistical models.
Interpretation of our results is limited by the post-hoc nature of the analyses, and the small number of samples in certain categories, particularly in the sub-cohort for M. catarrhalis (Table S2) and NTHi species acquisition (). Also, the sub-cohort of 36 patients used in the NTHi analysis can be considered a convenience sample due to the non-random nature of the sampling method applied since it comprised the first 36 patients enrolled in the study, although demographic characteristics of the sub-cohort were similar to those of the full cohort. Nevertheless, the analysis may be biased towards highly NTHi-colonized patients with COPD. Also, since the lung microbiome is known to be temporally dynamic [Citation2,Citation32], the patterns we observed in bacterial presence, acquisition, and apparition over one year need to be confirmed in larger and longer-term prospective longitudinal studies of patients with COPD. Additional functional studies of the interaction between bacteria and the immune system and species-specific interactions in COPD [Citation33] are also required to understand the mechanism of pathogenesis and identify biomarkers for the development of better therapeutic strategies. Any risk of pathogen replacement and microbiome dysbiosis may need to be assessed for new therapeutic approaches.
Conclusions
These initial results suggest that changes in NTHi antigen exposure and previous colonization with M. catarrhalis may have an impact on the risk of exacerbation in patients with COPD. Moreover, our findings confirm NTHi and M. catarrhalis as key targets in the development of drug or vaccine approaches to reducing the risk of AECOPD.
Trademarks
QIAamp is a trademark owned by or licenced to the QIAGEN Group. MagNA Pure is a trademark owned by or licenced to Roche. AMPure is a trademark owned by or licenced to Beckman Coulter, Inc. Nextera and MiSeq are trademarks owned by or licenced to Illumina Inc. SAS is a trademark owned by or licenced to SAS Institute Inc.
Author contributions
LM, AY, KO, TGP, SCC, LT, TMAW, JMD, and ND conceived and designed the study. LM, AY, KO, DWC, BS, TGP, VW, SCC, TMAW, JMD, and ND collected or generated the data. LM, AY, MCM, KO, DWC, BS, TGP, VW, SCC, TMAW, JMD, and ND analyzed or interpreted the data. All authors contributed substantially to the development of the manuscript and read and approved the final version.
Supplemental Material
Download MS Word (14 KB)Acknowledgements
Members of the AERIS Study Group: J. Alnajar (University of Southampton, Southampton, UK), R. Anderson (University of Southampton, Southampton, UK), E. Aris (GSK, Wavre, Belgium), W.R. Ballou (GSK, Rockville, MD, USA), A. Barton (University Hospital Southampton NHS Foundation Trust, Southampton, UK), S. Bourne (University of Southampton, Southampton, and Portsmouth Hospitals NHS Trust, Portsmouth, UK), M. Caubet (GSK, Wavre, Belgium), S.C. Clarke (University of Southampton, Southampton, UK), D.W. Cleary (University of Southampton, Southampton, UK), C. Cohet (GSK, Wavre, Belgium), N.A. Coombs (University of Southampton, Southampton, UK), K. Osman (University of Southampton, Southampton, UK), J-M. Devaster (GSK, Rixensart, Belgium), V. Devine (University of Southampton, Southampton, UK), N. Devos (GSK, Rixensart, Belgium), E. Dineen (University Hospital Southampton NHS Foundation Trust, Southampton, UK), T. Elliott (University of Southampton, Southampton, UK), R. Gladstone (Wellcome Sanger Institute, Hinxton, UK), S. Harden (University Hospital Southampton NHS Foundation Trust, Southampton, UK), J. Jefferies (University of Southampton, Southampton, UK), V.L. Kim (University of Southampton and University Hospital Southampton NHS Foundation Trust, Southampton, UK), P. Moris (GSK, Rixensart, Belgium), K.K. Ostridge (University of Southampton and University Hospital Southampton NHS Foundation Trust, Southampton, UK), T.G. Pascal (GSK, Wavre, Belgium), M. Peeters (GSK, Rixensart, Belgium), S. Schoonbroodt (GSK, Rixensart, Belgium), B. Sente (GSK, Rixensart, Belgium), K.J. Staples (University of Southampton and University Hospital Southampton NHS Foundation Trust, Southampton, UK), A.C. Tuck (University of Southampton, Southampton, UK), L. Welch (Solent NHS Trust, Southampton, UK), V. Weynants (GSK, Wavre, Belgium), T.M.A. Wilkinson (University of Southampton and University Hospital Southampton NHS Foundation Trust, Southampton, UK), A.P. Williams (University of Southampton, Southampton, UK), N.P. Williams (University of Southampton and University Hospital Southampton NHS Foundation Trust, Southampton, UK), C. Woelk (University of Southampton, Southampton, UK), M. Wojtas (University of Southampton, Southampton, UK), and S.A. Wootton (University Hospital Southampton NHS Foundation Trust, Southampton, UK). All members of the AERIS Study Group were involved in the planning, conduct, and/or reporting of the work described in the article.
The authors thank Stephanie Van Horn and Stephano Censini for their contributions to Moraxella catarrhalis sequencing. The authors also thank Business & Decision Life Sciences and Modis platforms for editorial assistance, manuscript coordination, and writing support, on behalf of GSK. Salomé Murinello (Modis, on behalf of GSK) and Bruno Baudoux (Business & Decision Life Sciences, on behalf of GSK) coordinated manuscript development and editorial support. Joanne Knowles (independent medical writer for Modis and Business & Decision Life Sciences) provided medical writing support.
Disclosure statement
LM, MCM, BS, TGP, VW, LT, JMD, and ND are employed by GSK, and LT, TGP, VW, JMD, and ND hold shares in GSK. VW and JMD are designated inventors on patents owned by GSK. AY was a consultant of ICON for GSK during the conduct of the study and is now employed by GSK. KO received a grant from GSK during the conduct of her Ph.D. DWC reports a grant from GSK during the conduct of this study. SCC received a grant from Pfizer outside of the submitted work and a grant from GSK during the conduct of the study. TMAW has received grants and non-financial support from GSK during the conduct of the study as well as grants from AstraZeneca, Synairgen, and MyMHealth and fees, reimbursement for travel, and meeting attendance from Boehringer Ingelheim, Chiesi, AstraZeneca, Synairgen, and MyMHealth outside of the submitted work. In addition, TMAW has a patent 2018 US Patent 62/479562—Immunogenic Composition, Use and Methods of Treatment—A novel vaccine to prevent exacerbations of COPD pending to GSK and is Founder and Director of MyMHealth Ltd. All authors declare having no other financial and non-financial relationships and activities.
Data availability statement
Anonymized individual subject data and study documents can be requested for further research from www.clinicalstudydatarequest.com.
Additional information
Funding
References
- Mammen MJ, Sethi S. COPD and the microbiome. Respirology. 2016;21(4):590–599.
- Wang Z, Singh R, Miller BE, et al. Sputum microbiome temporal variability and dysbiosis in chronic obstructive pulmonary disease exacerbations: an analysis of the COPDMAP study. Thorax. 2018;73(4):331–338.
- Pragman AA, Knutson KA, Gould TJ, et al. Chronic obstructive pulmonary disease upper airway microbiota alpha diversity is associated with exacerbation phenotype: a case-control observational study. Respir Res. 2019;20(1):114.
- Huang YJ, Sethi S, Murphy T, et al. Airway microbiome dynamics in exacerbations of chronic obstructive pulmonary disease. J Clin Microbiol. 2014;52(8):2813–2823.
- Sethi S, Murphy TF. Infection in the pathogenesis and course of chronic obstructive pulmonary disease. N Engl J Med. 2008;359(22):2355–2365.
- Sethi S, Evans N, Grant BJ, et al. New strains of bacteria and exacerbations of chronic obstructive pulmonary disease. N Engl J Med. 2002;347(7):465–471.
- Wilkinson TMA, Aris E, Bourne S, et al. A prospective, observational cohort study of the seasonal dynamics of airway pathogens in the aetiology of exacerbations in COPD. Thorax. 2017;72(10):919–927.
- Wilkinson TMA, Aris E, Bourne SC, et al. Drivers of year-to-year variation in exacerbation frequency of COPD: analysis of the AERIS cohort. ERJ Open Res. 2019;5(1):00248-2018.
- Garcha DS, Thurston SJ, Patel AR, et al. Changes in prevalence and load of airway bacteria using quantitative PCR in stable and exacerbated COPD. Thorax. 2012;67(12):1075–1080.
- Wang H, Gu X, Weng Y, et al. Quantitative analysis of pathogens in the lower respiratory tract of patients with chronic obstructive pulmonary disease. BMC Pulm Med. 2015;15:94.
- Molyneaux PL, Mallia P, Cox MJ, et al. Outgrowth of the bacterial airway microbiome after rhinovirus exacerbation of chronic obstructive pulmonary disease. Am J Respir Crit Care Med. 2013;188(10):1224–1231.
- Millares L, Ferrari R, Gallego M, et al. Bronchial microbiome of severe COPD patients colonised by Pseudomonas aeruginosa. Eur J Clin Microbiol Infect Dis. 2014;33(7):1101–1111.
- Jubinville E, Veillette M, Milot J, et al. Exacerbation induces a microbiota shift in sputa of COPD patients. PLOS One. 2018;13(3):e0194355.
- López Caro JC, Santibáñez M, García Rivero JL, et al. Sputum microbiome dynamics in chronic obstructive pulmonary disease patients during an exacerbation event and post-stabilization. Respiration. 2019;98(5):447–454.
- Haldar K, Bafadhel M, Lau K, et al. Microbiome balance in sputum determined by PCR stratifies COPD exacerbations and shows potential for selective use of antibiotics. PLOS One. 2017;12(8):e0182833.
- Wang Z, Bafadhel M, Haldar K, et al. Lung microbiome dynamics in COPD exacerbations. Eur Respir J. 2016;47(4):1082–1092.
- Bourne S, Cohet C, Kim V, et al. Acute exacerbation and respiratory InfectionS in COPD (AERIS): protocol for a prospective, observational cohort study. BMJ Open. 2014;4(3):e004546.
- Vestbo J, Hurd SS, Agusti AG, et al. Global strategy for the diagnosis, management, and prevention of chronic obstructive pulmonary disease: GOLD executive summary. Am J Respir Crit Care Med. 2013;187(4):347–365.
- Mayhew D, Devos N, Lambert C, et al. Longitudinal profiling of the lung microbiome in the AERIS study demonstrates repeatability of bacterial and eosinophilic COPD exacerbations. Thorax. 2018;73(5):422–430.
- Kim VL, Coombs NA, Staples KJ, et al. Impact and associations of eosinophilic inflammation in COPD: analysis of the AERIS cohort. Eur Respir J. 2017;50(4):1700853.
- Osman KL, Jefferies JMC, Woelk CH, et al. Patients with chronic obstructive pulmonary disease harbour a variation of Haemophilus species. Sci Rep. 2018;8(1):14734.
- Williams NP, Ostridge K, Devaster JM, et al. Impact of radiologically stratified exacerbations: insights into pneumonia aetiology in COPD. Respir Res. 2018;19(1):143.
- van den Bergh MR, Spijkerman J, Swinnen KM, et al. Effects of the 10-valent pneumococcal nontypeable Haemophilus influenzae protein D-conjugate vaccine on nasopharyngeal bacterial colonization in young children: a randomized controlled trial. Clin Infect Dis. 2013;56(3):e30–e39.
- Sandstedt SA, Zhang L, Patel M, et al. Comparison of laboratory-based and phylogenetic methods to distinguish between Haemophilus influenzae and H. haemolyticus. J Microbiol Methods. 2008;75(2):369–371.
- McCrea KW, Xie J, LaCross N, et al. Relationships of nontypeable Haemophilus influenzae strains to hemolytic and nonhemolytic Haemophilus haemolyticus strains. J Clin Microbiol. 2008;46(2):406–416.
- Murphy TF, Brauer AL, Sethi S, et al. Haemophilus haemolyticus: a human respiratory tract commensal to be distinguished from Haemophilus influenzae. J Infect Dis. 2007;195(1):81–89.
- Inouye M, Dashnow H, Raven LA, et al. SRST2: rapid genomic surveillance for public health and hospital microbiology labs. Genome Med. 2014;6(11):90.
- Meats E, Feil EJ, Stringer S, et al. Characterization of encapsulated and noncapsulated Haemophilus influenzae and determination of phylogenetic relationships by multilocus sequence typing. J Clin Microbiol. 2003;41(4):1623–1636.
- Parameswaran GI, Wrona CT, Murphy TF, et al. Moraxella catarrhalis acquisition, airway inflammation and protease-antiprotease balance in chronic obstructive pulmonary disease. BMC Infect Dis. 2009;9(1):178.
- Wang Z, Liu H, Wang F, et al. A refined view of airway microbiome in chronic obstructive pulmonary disease at species and strain-levels. Front Microbiol. 2020;11:1758.
- Ahearn CP, Gallo MC, Murphy TF. Insights on persistent airway infection by non-typeable Haemophilus influenzae in chronic obstructive pulmonary disease. Pathog Dis. 2017;75(4):ftx042.
- Dickson RP, Erb-Downward JR, Martinez FJ, et al. The microbiome and the respiratory tract. Annu Rev Physiol. 2016;78:481–504.
- Jacobs DM, Ochs-Balcom HM, Zhao J, et al. Lower airway bacterial colonization patterns and species-specific interactions in chronic obstructive pulmonary disease. J Clin Microbiol. 2018;56(10):e00330-18.