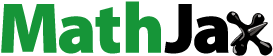
Abstract
Objectives
To characterise all bloodstream infections (BSIs) in a low antimicrobial resistance (AMR) prevalence setting with regard to the appropriateness of empirical antimicrobial therapy, compliance with the national clinical practice guideline, de-escalation practice and outcome.
Methods
A retrospective observational study including patients aged 18 years admitted to a university hospital in central Norway with positive blood culture in 2019.
Results
We included 756 BSI episodes in our analysis. Empirical antimicrobial therapy was in accordance with the national guideline in 534 (70.6%), and not in accordance in 190 (25.1%) of the BSI episodes. There was a statistically significant association between compliance with the national guideline and concordant empirical antimicrobial therapy (p = .001). De-escalation of antimicrobial therapy was possible but not done in 217 (31.1%) of the BSI episodes. Variables identified as independent predictors of discordant empirical antimicrobial therapy included hospital department, type of empirical antimicrobial regimen, bacterial species, and AMR. Independent predictors of intra-hospital case fatality rate were coverage of empirical antimicrobial therapy, CCI-score, SAPS-II score, site of infection, and type of empirical antimicrobial regimen. Furthermore, the intra-hospital and long-term unadjusted all-cause case fatality rates were increased (p < .001, log-rank test for overall difference in survival) for the patients who received discordant empirical antimicrobial therapy.
Conclusion
Our study shows that empirical antimicrobial therapy initiated in accordance with national guideline recommendations increases the likelihood of receiving concordant therapy. Discordant empirical antimicrobial therapy was associated with poorer outcomes, even in a setting with low AMR prevalence.
Introduction
Despite all advances in modern medicine bloodstream infection (BSI) is still a severe disease associated with high morbidity and mortality [Citation1]. BSI is often associated with sepsis syndrome [Citation2]. The outcome of BSI and sepsis is dependent on the prompt initiation of effective antimicrobial therapy [Citation3,Citation4]. Globally it is estimated that 31.5 million cases of sepsis are treated in hospitals each year, and sepsis contributes to an estimated 5.3 million deaths annually [Citation5]. The lowest incidence of sepsis is found in Northern Europe, including Norway [Citation5]. In Norway, it has been reported that sepsis contributed to 12.9% of hospital deaths in the period 2011–2012 [Citation6].
Early administration of adequate antimicrobial therapy is a cornerstone in the initial management of sepsis [Citation7]. Empirical antimicrobial therapy refers to the treatment administered before microbiological identification (ID) and antimicrobial susceptibility testing (AST), and such treatment is frequently based on clinical practice guideline recommendations, but also local empirical experience. The choice of empirical antimicrobial therapy should be broad enough to cover all likely pathogens, and therefore it might prove suboptimal for targeting the actual pathogen causing the infection [Citation8]. Concordant or discordant therapy refers to whether or not the administered empirical antimicrobials cover the detected pathogen and the pathogen's antimicrobial susceptibility profile.
Previous studies have shown that early broad-spectrum antimicrobial therapy reduces mortality in patients with severe sepsis and septic shock, and this initial antimicrobial therapy is described as one of the most important treatments for sepsis [Citation9]. However, the use of broad-spectrum antimicrobial therapy is also a known risk factor for the emergence of antimicrobial resistance (AMR) [Citation10]. Early reporting of microbial ID and AST provides opportunities for early targeting and de-escalation of antimicrobial therapy which is associated with decreased antimicrobial consumption and costs [Citation8]. De-escalation strategies have been shown to reduce the overall selection pressure for antimicrobial resistance, but have no overall effect on mortality [Citation11,Citation12].
The prevalence of AMR is low in Norway compared to most other countries. National surveillance on antimicrobial consumption and AMR in Norway in 2019, reported a prevalence of extended-spectrum beta-lactamase (ESBL) in Escherichia coli and Klebsiella pneumoniae isolated from blood culture of 7.1% and 5.7%, respectively [Citation13]. The rate of resistance to gentamicin was 5.9% for E. coli and 4.4% for K. pneumoniae. The report also showed that 0.8% of Staphylococcus aureus isolated from BSI was methicillin resistant (MRSA), and 2.2% of Enterococcus species were resistant to vancomycin. The Norwegian national guideline recommends a combination of penicillin and gentamicin as empirical therapy for sepsis with unknown aetiology and infection focus [Citation14]. This regimen covers the most common pathogenic bacteria causing BSI in Norway [Citation13]. Recommended as alternatives to this regimen is piperacillin/tazobactam or cefotaxime.
The aim of this study was to investigate empirical antimicrobial therapy in patients with BSI admitted to a tertiary care university hospital in Norway in 2019, and proportions that were concordant or discordant. Furthermore, we wanted to describe whether the empirical antimicrobial therapy administered was compliant with the national guideline recommendations, to what extent the antimicrobial therapy was targeted and de-escalated after the AST report was received, and whether discordant empirical antimicrobial therapy affected the outcome.
Materials and methods
Study population and study design
The study population in this retrospective observational study consisted of patients aged 18 years admitted to St. Olavs Hospital, a tertiary care university hospital in Trondheim, Norway, with a positive blood culture collected in the period 01.01.2019 to 31.12.2019. All patients that had blood cultures drawn and that turned out positive during this period were evaluated for inclusion.
An episode was excluded from the study if the microbe isolated from the blood culture was not considered to be the cause of the clinical infection, there was fungal growth in the blood culture, the patient was declared terminally ill before antimicrobial therapy was started, if necessary information was missing, or the patient was transferred to another hospital before antimicrobial therapy was initiated. A study group, consisting of a medical microbiologist, an infectious disease specialist, and two medical students evaluated the AST report and concluded whether or not the isolated microbe was classified as a contaminant. Typically, blood cultures with coagulase-negative staphylococci, Corynebacterium spp, and Bacillus cereus were classified as contaminants and excluded from further analyses if there was no information in the electronic patient record indicating that the isolated microbe should be considered the cause of the current clinical infection.
Laboratory procedures
Blood cultures, normally two sets of one BACTEC Plus/F Aerobic and one BACTEC Plus/F Anaerobic bottle with up to 10 mL blood in each bottle, were collected and incubated in an automated blood culture system BD BACTEC FX (Becton Dickinson, BD Diagnostics, USA). Bacteria and yeast isolated were identified using Maldi-TOF MS on a Microflex LT mass spectrometer (Bruker Daltonics) with the MBT 7854 MSP Library, and other standard methods [Citation15]. The standard EUCAST disc diffusion method was used to perform AST [Citation16]. In addition, the agar gradient method with Liofilchem® MIC test strips (Liofilchem, Italy) was used for minimum inhibitory concentration (MIC) determination in selected cases. Interpretation of results was done according to breakpoints from EUCAST and NordicAST [Citation17,Citation18].
Definitions
A BSI episode was defined by a positive blood culture with a plausible pathogenic microorganism and concomitant clinical infection, as judged and documented by the attending physician. Positive blood culture was defined by the growth of one or more microbial species in one or more blood culture bottles. Further, a monomicrobial BSI episode was defined as growth in the blood culture of one microbial species during an episode of clinical infection, while a polymicrobial BSI episode was defined as the growth of a second microbial species in the same or subsequent blood culture bottles collected within 72 h of the first detected microbial species. A new BSI episode in the same patient was defined as growth of a new microbial species in a sample collected more than 72 h after the initial BSI episode, or if there was growth of the same microbial species in a new blood culture sampled more than 30 days after the initial BSI episode, with a period of no symptoms of infection in between [Citation19,Citation20].
The BSI episode was classified as hospital-acquired if the patient had been admitted to the hospital more than 48 h prior to blood culture collection [Citation21]. A BSI episode was classified as procedure-related if the patient acquired the infection outside the hospital after having been subject to in-hospital procedures, and the clinician had concluded that it was due to this procedure. Typically, these procedures were prostate biopsies, transurethral resection of the prostate, and transurethral resection of bladder tumour. An episode was classified as community-acquired if it did not fall into either of the two first categories. Bacterial isolates belonging to the order Enterobacterales were classified as multidrug-resistant (MDR) if they were found to be resistant to three or more classes of antimicrobial drugs.
Comorbidity recorded in the electronic patient record at the time of admission was used to calculate the Charlson Comorbidity Index for each BSI episode [Citation22–24]. The probability of survival in relation to each episode was scored according to the Severe acute physiology score (SAPS) II at the time when the blood culture was collected [Citation25–27]. Since the information was lacking in the electronic patient journal for some of the variables, the calculation of the SAPS-II score was based on some modifications: PaO2/FiO2 data was not used for assessing the severity of the disease if the patient was on mechanical ventilation or CPAP (n = 39, 5.2%). In addition, variables with no data recorded in the electronic patient record were classified as normal based on the assumption that in the hospital routine clinicians commonly do not request data or analyses for functions where they do not suspect abnormal values and where they consider there is no clinical indication.
To describe the appropriateness of administered antimicrobial therapy, several methods were applied. The Norwegian national guideline for the appropriate use of antimicrobial therapy in hospitals was used to evaluate whether the initiated empirical regimen was compliant or not [Citation28]. Empirical antimicrobial therapy was considered concordant or discordant depending on whether or not the patient received antimicrobial therapy that covered the detected pathogen based on ID and AST. If the microbe had not been tested for any of the given empirical antimicrobials, inferred values from antimicrobials within the same class were used, as recommended by EUCAST and NordicAST in 2019 [Citation29]. If the AST report did not contain sufficient information for definite classification, the concordance of empirical antimicrobial therapy was classified as partial or uncertain. Targeted antimicrobial therapy was defined as any antimicrobial therapy given after the AST report was released by the microbiology department. Dosing of antimicrobial therapy was registered only for episodes with BSIs caused by S. aureus and treated with third-generation cephalosporins. In all other cases, dosing regimens were presumed to follow the standard regimen for each drug.
Data collection
Administrative, clinical, diagnostic, and therapy data were collected from the hospital's electronic patient records in a spreadsheet before analysis. The information on positive blood cultures was retrieved from the laboratory information system. The study group jointly concluded how the results should be classified in case of inconsistency in recorded data, or if the data should be classified as insufficient or inconclusive.
Statistical analyses
Binomial logistic regression with the calculation of odds ratios (ORs) with 95% confidence intervals (Cis) was used to investigate the associations between episode characteristics and discordance of empirical antimicrobial therapy, intra-hospital case fatality rate, and long-term case fatality rate. Fisher’s exact test was used to examine the association between compliance with the national guideline and concordance of empirical antimicrobial therapy. Log-rank test was used to compare the survival of the patients who received discordant empirical antimicrobial therapy versus the patients who received concordant empirical antimicrobial therapy. Statistical analyses were performed using IBM SPSS Statistics (Version 27.0.1.0 Armonk, NY: IBM Corp). A p-value < .05 was considered statistically significant.
Results
Episode characteristics
During the one-year study period, 924 patients had one or more blood cultures drawn that showed the growth of bacteria or fungi. Of these, 65 were excluded due to a lack of consent. Further, one or more exclusion criteria were met for 187 BSI episodes, as described in . The remaining 756 BSI episodes were eligible for inclusion, of which 642 (84.9%) occurred as unique, single BSI incidents, and 114 (15.1%) as multiple BSI incidents with two or more BSI episodes.
Figure 1. Flowchart showing the inclusion process and selection of patients and episodes, and the number of episodes per patient.
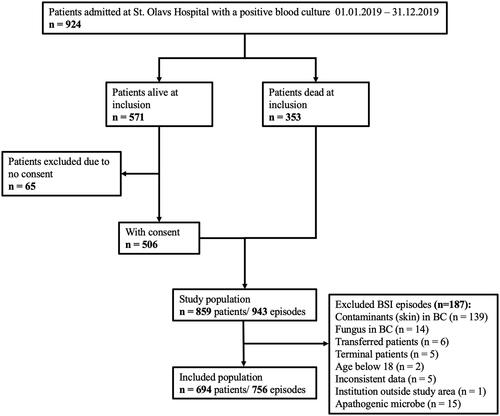
Episode characteristics are presented in and Table S1. The majority of patients with BSI episodes were admitted to a medical department (73.7%) while fewer were admitted to a surgical department (26.3%). The median age in this study was 74 years, with an interquartile range of 63–82 years. The most common comorbidity was diabetes mellitus. Penicillin allergy was reported in 5.2% of the patients.
Table 1. Characteristics of 756 episodes of bloodstream infection.
Microbe characteristics
Monomicrobial episodes constituted 693 of 756 (91.7%) BSIs. In 50 (6.6%) of the BSIs two different bacterial species were identified and in 13 (1.7%) three or more species were identified. The most frequently isolated bacterial pathogens were E. coli with 269 out of 756 (35.6%), followed by S. aureus with 129 out of 756 (17.1%) episodes, as shown in . Gram-negative, gram-positive and anaerobic pathogens constituted 443 (58.6%), 327 (43.3%) and 69 (9.1%) of the BSI episodes, respectively.
Table 2. Microbe characteristics.
Among the E. coli and K. pneumoniae isolates, 5.2% and 9.7% were ESBL producers, respectively. Furthermore, of the 23 BSI cases with ESBL-producing bacteria, 3 strains (two E. coli and one Enterobacter cloacae complex) were also classified as MDR. S. aureus which was found to be methicillin resistant (MRSA) was isolated from one BSI episode (0.8%), and one of 49 (2.0%) enterococcal isolates was vancomycin-resistant (VRE).
Empirical antimicrobial therapy
Overall, 536 of 756 (70.6%) BSI episodes were prescribed empirical antimicrobial therapy in line with therapy recommendations in the national clinical practice guideline. On the other hand, 190 of 756 (25.1%) episodes were prescribed other regimens inconsistent with the guideline recommendations. For the remaining 32 BSI episodes, specific therapy recommendations were either lacking in the guideline (0.3%), the BSI episode was too complex to be ascribed to a specific therapy recommendation (0.3%), or the patient did not receive empirical antimicrobial therapy (3.7%).
The empirical antimicrobial therapy was discordant in 121 (16.0%) and concordant in 594 (78.6%) BSI episodes. For BSI episodes, in which empirical antimicrobial therapy was compliant with national guideline recommendations, concordant and discordant therapy was observed in 452 (85.8%) and 75 (14.2%) episodes, respectively (). In contrast, for BSI episodes not compliant with the national guideline, 129 (73.7%) and 46 (26.3%) episodes received concordant and discordant therapy, respectively. There was a statistically significant association between compliance with the national guideline and concordant empirical antimicrobial therapy (Fisher’s exact test, p = .001).
Figure 2. Association between compliance with national clinical practice guideline (NCPG) and concordant empirical antimicrobial therapy. Of the episodes receiving empirical antimicrobial therapy compliant with NCPG, 452 (85.8%) and 75 (14.2%) were concordant and discordant, respectively. Of the episodes receiving empirical antimicrobial therapy not compliant with NCPG, 129 (73.7%) and 46 (26.3%) were concordant and discordant, respectively. Episodes with empirical antimicrobial therapy classified as either partial or uncertain coverage were excluded from the analysis. Asterisk indicates a statistically significant association between compliance with NCPG and concordant empirical antimicrobial therapy (Fisher’s exact test, p = .001).
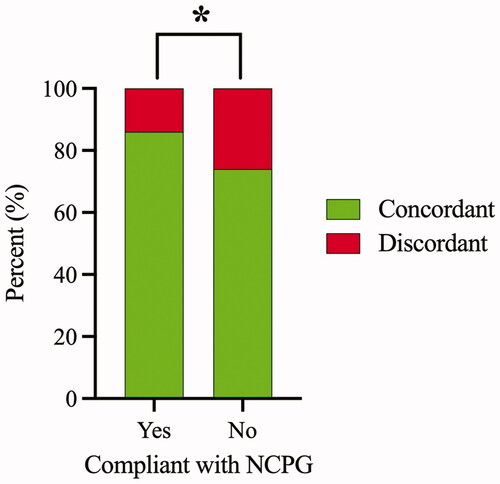
Predictors of discordant empirical therapy were established using univariable and multivariable logistic regression analysis. Variables identified as independent predictors of discordant empirical antimicrobial therapy included hospital department, type of antimicrobial regimen, bacterial species, and antimicrobial resistance profile ( and Table S2). Third-generation cephalosporins in monotherapy were the strongest predictor of discordant empirical antimicrobial therapy among the empirical regimens, with a ten-fold increase in the rate of discordant therapy compared with narrow-spectrum penicillin combined with an aminoglycoside. Compared to narrow-spectrum penicillin combined with an aminoglycoside, all the other empirical antimicrobial regimens had an increase in the frequency of discordant empirical therapy.
Table 3. Predictors for discordant empirical antimicrobial therapy when compared with causative bacterial species and its susceptibility profile*.
Targeted antimicrobial therapy
The mean time from blood culture sampling to the finalisation of the AST was 2.65 days (95% CI 2.53–2.76, median 2 days). The antimicrobial therapy administered after the AST results were released (hereby termed targeted antimicrobial therapy) was concordant in 697 (92.2%) of the 756 BSI episodes. However, 13 of 756 (1.7%) BSI episodes still received discordant antimicrobial therapy. For the remaining BSI episodes, empirical antimicrobial therapy was either discontinued without targeted therapy (n = 15, 2.0%), antimicrobial therapy was initiated with uncertain efficacy due to lack of AST (n = 10, 1.3%), or the patient had deceased before targeted therapy (n = 21, 2.8%).
De-escalation from empirical antimicrobial therapy to a more narrow-spectrum targeted regimen according to the AST report, was done in 480 (68.9%) of 697 eligible BSI episodes. In contrast, in 217 (31.1%) of 697 eligible BSI episodes, broad-spectrum antimicrobials were continued although the AST result indicated that conversion to narrow-spectrum antimicrobial therapy would have been feasible.
Case fatality rate
Discordant empirical antimicrobial therapy was significantly associated both with increased intra-hospital () and long-term () unadjusted all-cause case fatality rate (p-value < .001, log-rank test for overall difference in survival). The intra-hospital case fatality rate was 19.0% versus 7.4% for patients receiving discordant or concordant empirical antimicrobial therapy, respectively. The median follow-up time for long-time survival was 577.5 days (interquartile range 156–718.5 days).
Figure 3. Kaplan-Meier plot showing the cumulative intra-hospital (A) and long-term (B) survival of patients with BSI who received concordant or discordant empirical antimicrobial therapy. BSI episodes with empirical antimicrobial therapy classified as uncertain coverage, partial coverage and no empirical antimicrobial therapy given were excluded from the analysis.
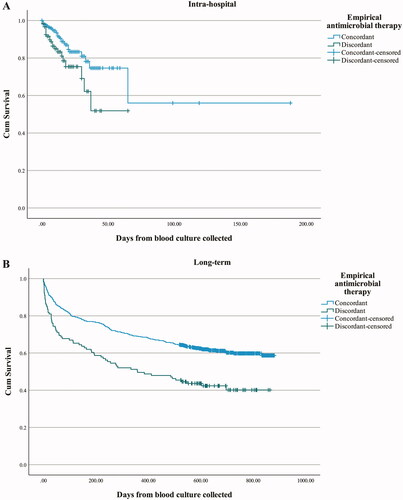
Coverage of empirical antimicrobial therapy, CCI-score, SAPS-II score, site of infection, and type of empirical antimicrobial regimen, were identified as independent predictors of intra-hospital case fatality rate ( and Table S3). The intra-hospital case fatality rate was about three times higher for patients receiving discordant empirical antimicrobial therapy, as compared to patients receiving concordant empirical antimicrobial therapy (OR 3.01, 95% CI 1.54–5.88).
Table 4. Predictors for intra-hospital case fatality rate.
Discussion
In this retrospective observational study of 756 BSI episodes over a one-year period at a tertiary care university teaching hospital in Norway with low AMR prevalence, we have shown that 70.6% received antimicrobial therapy compliant with national guideline recommendations. Of these, 14.2% were discordant. However, in BSI-episodes that received empirical antimicrobial therapy not compliant with the national guideline, 26.3% were discordant. We found a strong association between receiving a guideline-based regimen and concordant antimicrobial therapy. We have also reported that antimicrobial therapy was de-escalated, based on the AST report, to a more preferred regimen in 68.9% of BSI episodes while it was not de-escalated in 31.1% of BSI episodes. More importantly, we found that the intra-hospital and long-term case fatality rate was significantly higher for BSI episodes that received discordant therapy.
Surveillance of AMR prevalence is of paramount importance for the validity of therapy recommendations [Citation30]. During the two decades where we have had national systematic countrywide AMR surveillance, the prevalence of important resistant pathogens like MRSA, ESBL-producing bacteria, and VRE have been low in Norway [Citation13]. Based on decades of experience with clinical use combined with continued low AMR prevalence, narrow-spectrum beta-lactam therapy is still promoted in Norway for treatment of BSI and sepsis, often in combination with an aminoglycoside. The fact that 25.1% of included cases in our study were prescribed other regimens inconsistent with guideline recommendations, raises some concern. However, mechanisms that have impacted the prescribing habits among physicians were not a subject of this study.
We found a statistically significant association between compliance with guideline recommendations and concordant therapy. This finding supports that it is important to encourage the implementation of standardised therapy recommendations for specific clinical syndromes, as outlined in a previous antimicrobial stewardship guideline [Citation31]. A recent systematic review of nine stewardship objectives from nearly 150 studies concluded that guideline-adherent empirical antimicrobial therapy was associated with a relative risk reduction for mortality of 35% [Citation32].
Another finding is that 16.0% of BSI episodes received discordant empirical antimicrobial therapy. In a much larger American study, antimicrobial therapy was discordant in 19% of over 21.000 included BSI episodes [Citation2]. In that study, infection with an AMR pathogen strongly predicted receiving discordant antimicrobial therapy. In our study, we also found that ESBL-producing Enterobacterales were independently associated with discordant therapy, although very few BSI episodes were due to ESBL-producing strains. Another predictor of discordant therapy was monotherapy regimens consisting of a third-generation cephalosporin or piperacillin-tazobactam, as compared to a narrow-spectrum beta-lactam in combination with an aminoglycoside. This is probably because we, according to EUCAST, classified the standard dosing of cefotaxime and ceftriaxone as insufficient to cover for S. aureus infections [Citation17]. Of the 129 episodes with S. aureus infection in this study, 17 received a high dose of cefotaxime or ceftriaxone, while 6 received a standard dose. BSI episodes caused by Pseudomonas spp, Enterococcus spp, Enterobacter spp, Proteus spp, and as already mentioned S. aureus also predicted discordant therapy. This finding underscores that conditions other than AMR, including dosages of antimicrobial therapy, also need attention in order to reduce discordant therapy proportions.
In this study, we used clinical breakpoints for gentamicin as recommended by NordicAST in 2019. The gentamicin breakpoints were at that time classified as valid for all infections with Enterobacterales and staphylococci. In 2020 NordicAST changed its recommendation regarding gentamicin clinical breakpoints for Enterobacterales to be valid only for infections originating in the urinary tract. In addition, they stated that aminoglycoside treatment should be supported by other active treatments in case of systemic infections [Citation33]. This study was not designed to compare results for gentamicin treatment between patients with infection origin in the urinary tract vs other site of infection. However, we believe that the results of this study are relevant despite these changes.
Early conversion from empirical to targeted antimicrobial therapy is uniformly recommended. This also applies to severe infections [Citation34]. A recent meta-analysis concluded that antimicrobial monotherapy is non-inferior to duo therapy for severe infections, including sepsis and septic shock [Citation35]. In our study, antimicrobial therapy was changed to pathogen-directed, targeted monotherapy with a more narrow-spectrum antimicrobial in 68.9% of eligible BSI episodes, whilst 31.1% were continued on broad-spectrum regimens. We were not able to identify reasons for deferring de-escalation strategies. Of note, 5.2% of included BSI episodes were labelled penicillin-allergic, but this can hardly explain the reasons for broad-spectrum non-targeted therapy.
Data in our study indicate that the case fatality rate was significantly increased among BSI episodes that received discordant therapy. This is consistent with several previous clinical studies [Citation2,Citation36–43] and reviews [Citation34,Citation44]. Unlike most similar studies, we applied inclusion criteria that did not select between specific pathogens or patient groups at risk but rather assessed all BSI episodes occurring over a 12-month period. The intra-hospital all-cause case fatality rate among BSI episodes receiving discordant or concordant empirical antimicrobial therapy in our study was 19.0% and 7.4% respectively. The fact that we observed such a difference in case fatality rate depending on concordance of empirical antimicrobial therapy even in a low AMR prevalence setting, is noteworthy and provides support for the importance of early concordant antimicrobial therapy.
Predictors of case fatality rate in multivariable analysis in our study were discordant empirical therapy, comorbidity, infection severity at presentation, site of infection, and choice of antimicrobial regimen. This is comparable to previous studies [Citation2,Citation36–43]. Of note, we were unable to assess clinical stages in sepsis, and BSI episodes presenting with especially pronounced clinical signs may have been allocated to broad-spectrum antimicrobial therapy. We therefore cannot rule out confounding by indication.
We consider it to be a strength of this study that the BSI episodes were identified based on laboratory-confirmed blood cultures systematically registered in the laboratory information system, and that the study included a high number of consecutive, unselected cases at a single centre tertiary care university teaching hospital in Norway where all types of inpatient departments and medical specialities are represented. However, there were also some limitations of this study. A subset of eligible patients still alive when the study started did not consent to participate and this may have led to the overrepresentation of diseased patients. Retrospective collection of clinical data depended on the availability and accuracy of such data in electronic patient records, and microbiology results from samples other than blood culture were not recorded in this study. Our practice of classifying missing values for the calculation of SAPS-II score as normal may have led to the underestimation of the SAPS-II score. Dosages of antimicrobial therapy were only available on scanned paper charts and therefore difficult to access. Such information was only registered for the use of third-generation cephalosporins in staphylococcal infections in this study. In addition, it is possible in a retrospective study that factors not recorded may have influenced the results obtained. Finally, as this is a single-centre study from a low antimicrobial resistance setting, whether the results from this study can be extrapolated to international settings needs further investigation.
In conclusion, this study showed that empirical antimicrobial therapy for BSI is more concordant with AST reports if such therapy is in accordance with national clinical practice guideline recommendations. The case fatality rate was significantly higher for BSI episodes that received discordant therapy. In this study, we disclosed predictors for discordant antimicrobial therapy. Antimicrobial stewardship programmes in general encourage health professionals and authorities to assess predictors for discordant empirical antimicrobial therapy carefully and implement antimicrobial stewardship measures to facilitate therapy prescribing. Measures should include strategies that target prompt identification and AST, guideline-based initiation of therapy, and compulsory early therapy assessments. Regardless of AMR prevalence, we encourage others to investigate concordant antimicrobial therapy in order to secure rigorous clinical guideline recommendations.
Ethical considerations
The Regional Committee for Medical and Health Research Ethics, health region mid-Norway (REK midt 210524) approved the study. Deceased patients at the start of study in June 2021 were included in the study without consent, while patients still alive received a written study invitation with opportunity to withdraw from the study. A data protection impact assessment was performed and approved by the hospital before start of study.
Author contributions
This study was conceived and designed by KG, EH, BW and JEA. KG, EH and BW acquired the data. KG, EH, BW and ØS analysed and interpreted the data. The study was supervised by BW and JEA, with input from JKD. KG and EH drafted the article, which was critically revised by BW, JKD, and JEA.
Supplemental Material
Download MS Word (45.2 KB)Acknowledgements
We express our sincerest gratitude towards Frode Width Gran for help with patient identification and Kjersti Haugum, Nina Bäcklund and the Department of Medical Microbiology, St. Olavs hospital for help with dispatch of invitation letters to the patients.
Disclosure statement
Author Bjørn Waagsbø is a representative and holds a 20% position to the directory group in the Norwegian Directorate of Health that seek to revise recommendations in the national clinical practice guideline for antimicrobial therapy.
Data availability statement
The datasets used and/or analysed during the current study are not publicly available due to restrictions from The Regional Committee for Medical and Health Research Ethics, health region mid-Norway (REK midt 210524).
Additional information
Funding
References
- Goto M, Al-Hasan MN. Overall burden of bloodstream infection and nosocomial bloodstream infection in North America and Europe. Clin Microbiol Infect. 2013;19(6):501–509.
- Kadri SS, Lai YL, Warner S, et al. Inappropriate empirical antibiotic therapy for bloodstream infections based on discordant in-vitro susceptibilities: a retrospective cohort analysis of prevalence, predictors, and mortality risk in US hospitals. Lancet Infect Dis. 2021;21(2):241–251.
- Marquet K, Liesenborgs A, Bergs J, et al. Incidence and outcome of inappropriate in-hospital empiric antibiotics for severe infection: a systematic review and meta-analysis. Crit Care. 2015;19(1):63.
- Paul M, Shani V, Muchtar E, et al. Systematic review and meta-analysis of the efficacy of appropriate empiric antibiotic therapy for sepsis. Antimicrob Agents Chemother. 2010;54(11):4851–4863.
- Fleischmann C, Scherag A, Adhikari NK, et al. Assessment of global incidence and mortality of hospital-treated sepsis. Current estimates and limitations. Am J Respir Crit Care Med. 2016;193(3):259–272.
- Knoop ST, Skrede S, Langeland N, et al. Epidemiology and impact on all-cause mortality of sepsis in norwegian hospitals: a national retrospective study. PLOS One. 2017;12(11):e0187990.
- Esposito S, De Simone G, Boccia G, et al. Sepsis and septic shock: new definitions, new diagnostic and therapeutic approaches. J Glob Antimicrob Resist. 2017;10:204–212.
- Berild D, Mohseni A, Diep LM, et al. Adjustment of antibiotic treatment according to the results of blood cultures leads to decreased antibiotic use and costs. J Antimicrob Chemother. 2006;57(2):326–330.
- Ferrer R, Martin-Loeches I, Phillips G, et al. Empiric antibiotic treatment reduces mortality in severe sepsis and septic shock from the first hour: results from a guideline-based performance improvement program*. Crit Care Med. 2014;42(8):1749–1755.
- Abushaheen MA, Fatani AJ, Alosaimi M, et al. Antimicrobial resistance, mechanisms and its clinical significance. Dis Mon. 2020;66(6):100971.
- Guo Y, Gao W, Yang H, et al. De-escalation of empiric antibiotics in patients with severe sepsis or septic shock: a meta-analysis. Heart Lung. 2016;45(5):454–459.
- Turnidge J. Impact of antibiotic resistance on the treatment of sepsis. Scand J Infect Dis. 2003;35(9):677–682.
- NORM/NORM-VET 2019. Usage of antimicrobial agents and occurence of antimicrobial resistance in Norway. Tromsø/Oslo; 2020.
- Antibiotika i sykehus Oslo: Helsedirektoratet; 2018. [cited 2021 9.11]. Available from: https://www.helsedirektoratet.no/retningslinjer/antibiotika-i-sykehus/sepsis.
- Jakovljev A, Bergh K. Development of a rapid and simplified protocol for direct bacterial identification from positive blood cultures by using matrix assisted laser desorption ionization time-of- flight mass spectrometry. BMC Microbiol. 2015;15:258.
- Matuschek E, Brown DF, Kahlmeter G. Development of the EUCAST disk diffusion antimicrobial susceptibility testing method and its implementation in routine microbiology laboratories. Clin Microbiol Infect. 2014;20(4):O255–66.
- The European Committee on Antimicrobial Susceptibility Testing. Breakpoint tables for interpretation of MICs and zone diameters, version 10.0, 2020. [cited 2021 Oct 11]. Available from: https://www.eucast.org.
- Nordic Committee on Antimicrobial Suceptibility Testing NordicAST; 2020. [cited 2021 Oct 11]. Available from: http://www.nordicast.org/index.
- Mehl A, Asvold BO, Kummel A, et al. Trends in antimicrobial resistance and empiric antibiotic therapy of bloodstream infections at a general hospital in Mid-Norway: a prospective observational study. BMC Infect Dis. 2017;17(1):116.
- Mehl A, Åsvold BO, Lydersen S, et al. Burden of bloodstream infection in an area of Mid-Norway 2002-2013: a prospective population-based observational study. BMC Infect Dis. 2017;17(1):205.
- World Health Organization. Prevention of hospital-acquired infections: a practical guide/editors. G. Ducel, J. Fabry and L. Nicolle, 2nd. ed. World Health Organization 2002. Available from https://apps.who.int/iris/handle/10665/67350.
- Charlson ME, Pompei P, Ales KL, et al. A new method of classifying prognostic comorbidity in longitudinal studies: development and validation. J Chronic Dis. 1987;40(5):373–383.
- Quan H, Li B, Couris CM, et al. Updating and validating the charlson comorbidity index and score for risk adjustment in hospital discharge abstracts using data from 6 countries. Am J Epidemiol. 2011;173(6):676–682.
- Radovanovic D, Seifert B, Urban P, et al. Validity of charlson comorbidity index in patients hospitalised with acute coronary syndrome. Insights from the nationwide AMIS Plus registry 2002-2012. Heart. 2014;100(4):288–294.
- Le Gall JR, Lemeshow S, Saulnier F. A new simplified acute physiology score (SAPS II) based on a European/North American multicenter study. Jama. 1993;270(24):2957–2963.
- Capuzzo M, Valpondi V, Sgarbi A, et al. Validation of severity scoring systems SAPS II and APACHE II in a single-center population. Intensive Care Med. 2000;26(12):1779–1785.
- Beck DH, Smith GB, Pappachan JV, et al. External validation of the SAPS II, APACHE II and APACHE III prognostic models in South England: a multicentre study. Intensive Care Med. 2003;29(2):249–256.
- Helsedirektoratet. Antibiotika i sykehus Oslo: Helsedirektoratet; [updated 2021 May 27; cited 2021 Oct 11]. Available from: https://www.helsedirektoratet.no/retningslinjer/antibiotika-i-sykehus#referere.
- NordicAST. Nordic Comittee on Antimicrobial Susceptibility Testing. [cited 2022 July 04]. Available from: https://view.officeapps.live.com/op/view.aspx?src=http%3A%2F%2Fs3-eu-west-1.amazonaws.com%2Fhl-intranet%2Ffiles%2Fb093b042c80456a448f7e88a11f2e864a33c9202%2Fv_9_0_brytningspunkttabell_nordicast_no_final190325_locked.xlsx&wdOrigin=BROWSELINK.
- Möller V, Östholm-Balkhed Å, Berild D, et al. Antibiotic resistance among major pathogens compared to hospital treatment guidelines and antibiotic use in nordic hospitals 2010-2018. Infect Dis. 2021;53(8):607–618.
- Barlam TF, Cosgrove SE, Abbo LM, et al. Implementing an antibiotic stewardship program: guidelines by the infectious diseases society of america and the society for healthcare epidemiology of america. Clin Infect Dis. 2016;62(10):e51–e77.
- Schuts EC, Hulscher M, Mouton JW, et al. Current evidence on hospital antimicrobial stewardship objectives: a systematic review and meta-analysis. Lancet Infect Dis. 2016;16(7):847–856.
- NordicAST. Nordic comittee on antimicrobial susceptibility testing. Available from: https://view.officeapps.live.com/op/view.aspx?src=http%3A%2F%2Fs3-eu-west-1.amazonaws.com%2Fhl-intranet%2Ffiles%2F4474e596b67691fa4ee06ff89f4d3c98f7c568d3%2F200207_v_10_0_nordicast_no_protected.xlsx&wdOrigin=BROWSELINK.
- Evans L, Rhodes A, Alhazzani W, et al. Surviving sepsis campaign: international guidelines for management of sepsis and septic shock 2021. Intensive Care Med. 2021;47(11):1181–1247.
- Sjövall F, Perner A, Hylander Møller M. Empirical mono- versus combination antibiotic therapy in adult intensive care patients with severe sepsis – a systematic review with meta-analysis and trial sequential analysis. J Infect. 2017;74(4):331–344.
- Ju M, Huang Y, Xu X, et al. Predictors of mortality in adult patients with methicillin-resistant Staphylococcus aureus bloodstream infection: a meta-analysis and systematic review. Ann Palliat Med. 2021;10(8):8617–8627.
- Corcione S, Mornese Pinna S, Lupia T, et al. Antibiotic De-escalation experience in the setting of emergency department: a retrospective, observational study. J Clin Med. 2021;10(15):3285.
- Lin TL, Chang PH, Chen IL, et al. Risk factors and mortality associated with multidrug-resistant gram-negative bacterial infection in adult patients with abdominal surgery. J Hosp Infect. 2021;119:P22–P32.
- Baltas I, Stockdale T, Tausan M, et al. Impact of antibiotic timing on mortality from gram-negative bacteraemia in an english district general hospital: the importance of getting it right every time. J Antimicrob Chemother. 2021;76(3):813–819.
- Gradel KO, Jensen US, Schønheyder HC, et al. Impact of appropriate empirical antibiotic treatment on recurrence and mortality in patients with bacteraemia: a population-based cohort study. BMC Infect Dis. 2017;17(1):122.
- Tang Y, Wu X, Cheng Q, et al. Inappropriate initial antimicrobial therapy for hematological malignancies patients with gram-negative bloodstream infections. Infection. 2020;48(1):109–116.
- Yamaga S, Shime N. Association between appropriate empiric antimicrobial therapy and mortality from bloodstream infections in the intensive care unit. J Infect Chemother. 2018;24(4):267–271.
- Suppli M, Aabenhus R, Harboe ZB, et al. Mortality in enterococcal bloodstream infections increases with inappropriate antimicrobial therapy. Clin Microbiol Infect. 2011;17(7):1078–1083.
- Kollef MH, Shorr AF, Bassetti M, et al. Timing of antibiotic therapy in the ICU. Crit Care. 2021;25(1):360.