Abstract
Background
There is a critical need for a rapid and sensitive pathogen detection method for septic patients. This study aimed to investigate the diagnostic efficacy of Digital droplet polymerase chain reaction (ddPCR) in identifying pathogens among suspected septic patients.
Methods
We conducted a prospective pilot diagnostic study to clinically validate the multiplex ddPCR panel in diagnosing suspected septic patients. A total of 100 sepsis episodes of 89 patients were included in the study.
Results
In comparison to blood culture, the ddPCR panel exhibited an overall sensitivity of 75.0% and a specificity of 69.7%, ddPCR yielded an additional detection rate of 17.0% for sepsis cases overall, with a turnaround time of 2.5 h. The sensitivity of ddPCR in the empirical antibiotic treatment and the non-empirical antibiotic treatment group were 78.6% versus 80.0% (p > 0.05). Antimicrobial resistance genes were identified in a total of 13 samples. Whenever ddPCR detected the genes beta-lactamase-Klebsiella pneumoniae carbapenemase (blaKPC) or beta-lactamase-New Delhi metallo (blaNDM), these findings corresponded to the cultivation of carbapenem-resistant gram-negative bacteria. Dynamic ddPCR monitoring revealed a consistent alignment between the quantitative ddPCR results and the trends observed in C-reactive protein and procalcitonin levels.
Conclusions
Compared to blood culture, ddPCR exhibited higher sensitivity for pathogen diagnosis in suspected septic patients, and it provided pathogen and drug resistance information in a shorter time. The quantitative results of ddPCR generally aligned with the trends seen in C-reactive protein and procalcitonin levels, indicating that ddPCR can serve as a dynamic monitoring tool for pathogen load in septic patients.
Introduction
Sepsis/septic shock are one of the leading causes of mortality in critically ill patients, research has shown that patients who receive antibiotic therapy within the first hour of diagnosis have a survival rate close to 80%, every hour of delay in treatment results in a 7.6% decrease in survival, and if treatment is delayed for more than six hours, the mortality rate reaches 58% [Citation1]. Early adequate antibiotic therapy is a key to improve patient outcomes, the successful antimicrobial treatment depends first on the rapid and accurate diagnosis of pathogenic microbes [Citation2]. Community‑acquired sepsis in immunocompetent adults usually involve drug‑susceptible bacteria, while healthcare‑associated sepsis are frequently due to multidrug‑resistant strains. Therefore, obtaining the identification of the causative microorganism and its resistance information as early as possible has become a key factor in the early and rational use of antimicrobial drugs. Over the past few decades, culture methods have been the gold standard for pathogen detection, although their time-consuming nature and low sensitivity do not meet the clinical requirements of fast and accurate diagnosis [Citation3]. Therefore, there is an urgent need for a rapid and highly sensitive detection method to improve the timeliness and detection rate of pathogenic microorganisms causing sepsis.
Droplet digital PCR (ddPCR) technology is the third-generation nucleic acid detection technique, following the first-generation conventional PCR and the second-generation quantitative PCR. It is characterised by ultra-high sensitivity, absolute quantification, independence from standard curves, and high stability [Citation4]. It is particularly suitable for detecting extremely low amounts of nucleic acid molecules and shows great potential in pathogenic microorganism identification, liquid biopsy for tumours, non-invasive prenatal screening, and other applications [Citation5, Citation6]. In the field of infectious diseases, utilising the ultra-high sensitivity and absolute quantification capabilities of ddPCR, rapid detection of pathogenic microorganism DNA in peripheral blood can be achieved. This enables the swift diagnosis of pathogens, potentially reducing the reporting time from several days to just a few hours when compared to conventional culture methods [Citation7]. However, there is currently insufficient evidence and research on the application and effectiveness of ddPCR in suspected septic patients in the intensive care unit.
This study aims to investigate the diagnostic efficacy of ddPCR in identifying pathogens among suspected septic patients in the intensive care unit. The goal is to find a method that can rapidly diagnose the causative microorganisms and resistance information of septic patients in the intensive care unit, thereby improving the prognosis.
Methods
Ethical approval
This study was conducted in compliance with the Declaration of Helsinki and approved by the ethics committee of the institutional review board of our hospital (Reference number: IIT20230015B).
Study population
This study was a prospective pilot diagnostic study to clinically validate the multiplex ddPCR panel in diagnosing suspected septic patients. This work was performed in the intensive care unit of our hospital from December8, 2022, to June19, 2023. The participants were all older than 18 years, inclusion criteria include: clinical suspicion of sepsis according to The Surviving Sepsis Campaign Bundle [Citation8] and meeting the following criteria: a) meeting the diagnostic criteria for sepsis: Sequential organ failure assessment (SOFA) score increment ≥ 2 points or quick SOFA (qSOFA) ≥ 2 points; b) presence of any of the following clinical features:fever (> 38.3 °C) or hypothermia (< 36.0 °C); White blood cell count> 12 × 10^9/L or < 4 × 10^9/L;, Procalcitonin > 2 times the upper limit of normal, that were hard to explain with non-infectious factors. Exclusion Criteria: a) women who are pregnant or breastfeeding; b) patients with confirmed sepsis who already had a clear pathogen infection before undergoing ddPCR testing. The study protocol allowed the inclusion of multiple episodes of suspected sepsis occurring in one patient, during the second instance of suspected sepsis within the same patient’s hospitalisation, two clinical doctors are required to comprehensively assess the previous suspected sepsis for recovery through laboratory examinations and clinical manifestations. During the study period, following inclusion and exclusion criteria, a total of 100 sepsis episodes from 89 patients were enrolled for analysis.
Sample and clinical data collection
Patients with suspicion of sepsis included in the study will undergo routine clinical assessments including complete blood count, inflammatory markers, blood biochemistry and cultures of samples from suspected infection sites including blood, abdominal, respiratory, skin and soft tissue, and other tissue/fluid during their clinical management. At the time of the initial blood culture, a peripheral blood sample (5 mL) will be concurrently inoculated into a free DNA preservation tube used for ddPCR detection. Among patients with positive blood cultures, we performed dynamic monitoring of ddPCR detection on the 3rd and 6th days after the initial sampling. Synchronously record the clinical data of enrolled patients, including patient demographics (age, gender, body mass index (BMI)), comorbidities, immunosuppressant exposure(use of immunosuppressants for more than 1 week prior to sampling), empirical antibiotic therapy (EAT, empirical use of broad-spectrum antibiotics for more than 48 h prior to sampling), presence of septic shock, sequential organ failure assessment score, laboratory tests, 28-day mortality rate, and for patients undergoing dynamic monitoring with ddPCR, simultaneously document the results of white blood cell count, C-reactive protein, and procalcitonin on the day of sample collection.
Sample culture
When a sepsis diagnosis was suspected a set of 2 blood culture samples was drawn from each patient according to The Surviving Sepsis Campaign Bundle [Citation8] within 1 h, with aerobic and anaerobic culture for each sample. The results of the antibiotic susceptibility test were interpreted according to the Clinical and Laboratory Standards Institute guidelines [Citation9]. Strains that showed resistance to imipenem or meropenem were identified as carbapenem-resistant. Polymerase chain reactions were performed to detect carbapenem-encoding resistance genes (beta-lactamase-Klebsiella pneumoniae carbapenemase (blaKPC) or beta-lactamase-New Delhi metallo (blaNDM)) [Citation10]. Methicillin-resistant Staphylococcus sp. was speculated when gene methicillin resistance cassette A (mec-A)-positive.
ddPCR detection
ddPCR analysis was performed using a 5-fluorescent-channel droplet digital PCR system (Pilot Gene Technology [Hangzhou] Co., Ltd., Hangzhou, China). The targeting pathogens from PilotBac, PilotFungi, and PilotAMR assay panels are provided in . Information on the primers and probes used for detecting pathogenic microorganisms through ddPCR showed in the Supplemental material. Peripheral venous blood (5 mL) was collected from each patient into a cell-free DNA storage tube (CWBio, Beijing, China). Blood samples were stored at 4°C in the refrigerator, and all ddPCR tests were conducted on the same day or the following day after receiving the samples. Plasma was immediately isolated after centrifugation at 1,600 × g, and 4 °C for 15 min. DNA was extracted from 2 mL of plasma using the CF1 nucleic acid purification Kit (Pilotgene, Hangzhou, China) and the Auto-Pure 10B nucleic acid purification system (Allsheng Instruments, Hangzhou, China) following the manufacturer’s protocol. DNA was eluted in 100 µL of elution buffer and used for ddPCR assay. The specific ddPCR sample preparation and operational procedures can refer to previous publication [Citation11]. Further, ddPCR testing procedures were performed for about 2.5 h according to the manufacturer’s protocol (). The copies of each targeted pathogen or gene were reported by ddPCR results.
Figure 1. The work process of ddPCR test. ddPCR testing procedures were performed for about 2.5 h according to the manufacturer’s protocol.
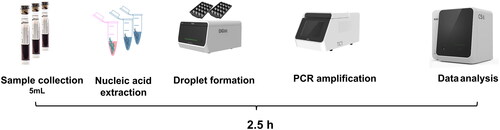
Table 1. Pathogens and antimicrobial resistance (AMR) genes included in the ddPCR panel.
Definitions and interpretation of culture and ddPCR results
Results of ddPCR and culture were independently verified by two intensive care unit physicians. Blood culture positive (BC+) is defined as a positive result in blood culture, and it is confirmed by clinical assessment to exclude the possibility of contamination. ddPCR positive (ddPCR+) is defined as the detection of at least one pathogenic microorganism in ddPCR testing. To resolve discrepancies, discordant ddPCR+/BC − results were classified as probable sepsis, possible sepsis cases similar to previous studies [Citation11, Citation12]. The following definitions were used for each classification, (i) probable sepsis: ddPCR result was concordant with a microbiological test performed within 7 days of sample collection from other extra-blood site, ‘Day 0’ is defined as the day of the ddPCR blood sample collection; (ii) possible: without microbiological data but ddPCR result had potential for pathogenicity based on clinical presentation and laboratory findings. When a possible sepsis result occurs, since there was no microbial evidence from cultivation in this set of samples, in order to investigate whether these samples are indeed sepsis samples, we conducted exploratory efforts by submitting the samples for first-generation sequencing also known as Sanger sequencing to observe their concordance with ddPCR. ddPCR additional detection rate = (probable sepsis – BC+/ddPCR-)/overall-samples.
In our collection of clinical samples suspected of sepsis, there was a portion empirical antibiotic therapy was initiated within ≤48 h. Another portion empirical antibiotic therapy was administered for >48 h. To assess the impact of antibiotic treatment on the diagnostic performance of ddPCR, we divided the patients into empirical antibiotic therapy (EAT) and non-empirical antibiotic therapy (Non-EAT) group.
To further analyse the clinical application value of ddPCR in rapid pathogen detection, we defined the time from sample receipt to result reporting as the turnaround time.
First-generation sequencing (Sanger sequencing)
To verify the results of ddPCR test, DNA fragment of target pathogen was amplified using nested primers and validated with Sanger sequencing. It involves designing two primer pairs, one for the outer fragment and one for the inner fragment. The target gene for primer design of each pathogen is the same as the one used for ddPCR detection. The length of outer fragment is about 200 ∼ 300bp, and the inner fragment 120 ∼ 180bp. The inner fragment was used for two independent Sanger sequencing reactions, one with forward inner primer and the other with reverse inner primer. Both rounds of nested PCR were performed in a 20 μl reaction system. The reaction contained 0.5 μl of 10 μM forward and reverse primers, 4 μl of 5 × PCR mix, 5 μl of sample DNA for the first round PCR and 1 μl of first round PCR product for the second round as PCR template. Thermal cycle parameters included 95 °C for 5 min, followed by 35 cycles of 95 °C for 20 s, 58 °C for 30 s, 72 °C for 30 s, and finally extended for 5 min at 72 °C. PCR products were analysed in 1.5% agarose gel electrophoresis and cleaned up with PCR purification kit. Sanger sequencing was performed with the forward and reverse primers of second round PCR on an ABI 3730XL DNA Analyser according to the manufacturer’s instructions.
Statistics analysis
For continuous variables that followed the Kolmogorov-Smirnov test, we described them using means, while medians were used when they did not conform to the test. Chi-square tests were employed to assess independent binomial variables, with a significance level set at 0.05. To compare differences across ddPCR subgroups, we analysed the results using the Mann-Whitney test. All statistical analyses and figure generation were performed using SPSS 20.0 (IBM Corp., Armonk, NY, USA), Prism 8.4.0 software (GraphPad Software, San Diego, CA, USA), and RStudio 4.0.3 software (RStudio Software, Boston, MA, USA).
Results
Enrollment of patients and clinical characteristics
A total of 100 sepsis episodes of 89 patients were included in the study. The clinical characteristics of the eligible patients are presented in . A total of 24 samples exhibited positive blood culture (BC+) results. Out of the 24 BC + strains, three were not included in the ddPCR microbial panel, including Burkholderiaenocepacia, Pantoeaagglomerans and Staphylococcus warneri. Among the remaining 21 BC + strains, 18 were detected by ddPCR. Two Serratia marcescens and one Proteus mirabilis were missed by ddPCR.
Table 2. Clinical characteristics of enrolled patients.
Among 76 negative blood culture(BC-) cases, ddPCR did not detect any strains (ddPCR-) in 29 cases, while it detected strains (ddPCR+) in 47 cases. In these 47 BC-/ddPCR + samples, there were 23 cases of probable sepsis and 24 cases of possible sepsis. About the 23 cases of probable sepsis, the pathogens and extra-blood culture site were described in . About the 24 cases of possible sepsis, we re-submitted the samples for first-generation sequencing. The first-generation sequencing results revealed that 13 samples were consistent with the ddPCR test results, while 11 samples were not ().
Figure 2. Flow chart for patient enrolment and results analysis. (i) Probable sepsis: ddPCR result was concordant with a microbiological test performed within 7 days of sample collection from other extra-blood site, ‘Day 0’ is defined as the day of the ddPCR blood sample collection; (ii) Possible sepsis: without microbiological data but ddPCR result had potential for pathogenicity based on clinical presentation and laboratory findings.
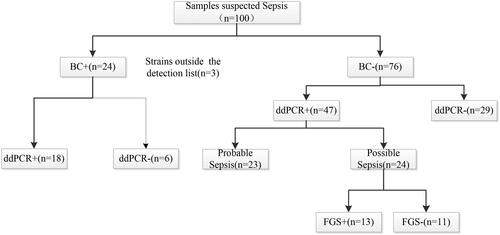
Table 3. Descriptions of the pathogens and extra-blood culture site in probable sepsis patients.
Performance of the ddPCR testing and blood culture
The blood culture positivity rate stood at 24.0% (24/100), ddPCR positivity rate stood at 65.0% (65/100). Excluding the three BC + samples not encompassed by the ddPCR detection panel, the remaining 21 BC + samples revealed a distribution of detected pathogens as illustrated in . Among the 21 BC + samples, Klebsiella pneumoniae (n = 5) ranked highest in prevalence, followed by Serratia marcescens (n = 3), Proteus mirabilis (n = 3), and Enterococcus faecalis (n = 3). In the 65 ddPCR + samples, a total of 112 strains were identified, with the distribution of detected pathogens showcased in . The highest detection count was attributed to Klebsiella pneumoniae (n = 24), followed by Escherichia coli (n = 16) and Enterococcus faecalis (n = 13).
Evaluation of the diagnostic performance of ddPCR compare to blood culture
Among BC-/ddPCR + samples, 23 cases were identified as probable sepsis samples. Further subgroup analysis revealed that within the G + BC-/ddPCR + subgroup, 21 probable sepsis samples were associated with Gram-positive (G+) cocci, while the G-BC-/ddPCR + subgroup comprised 32 probable sepsis samples related to Gram-negative (G-) bacilli. Upon comprehensive assessment of ddPCR's diagnostic performance, it was found that compared to blood culture, ddPCR exhibited an overall sensitivity of 75.0% (95% CI, 52.9,89.4) and a specificity of 69.7% (95% CI, 58.0,79.5) for sepsis diagnosis. For sepsis cases related to Gram-positive cocci, ddPCR demonstrated a sensitivity of 100% (95% CI, 46.3,100.0) and a specificity of 88.4% (95% CI, 79.8, 93.8). In the context of sepsis related to Gram-negative bacilli, ddPCR exhibited a sensitivity of 66.7% (95% CI, 41.2, 85.6) and a specificity of 75.6% (95% CI, 64.7,84.1). Notably, ddPCR displayed a relatively higher detection rate compared to blood culture (41% [41/100] versus 24% [24/100], χ2=6.587; p = 0.01), resulting in an additional detection rate of 17% for sepsis cases overall ().
Table 4. The diagnostic performance of ddPCR compare to BC in suspected sepsis patients.
Furthermore, we compared the turnaround time of ddPCR and blood culture detection. Due to the established and streamlined process of ddPCR, the entire testing procedure typically takes only 2–3 h to complete. We recorded the time it took for 24 blood culture-positive samples to be processed from sample receipt in the microbiology department to obtaining susceptibility results, the median time was 47.5 h (IQR, 42, 62.25 h). While the specific turnaround time for ddPCR cannot be precisely determined, due to ddPCR testing procedures were performed for about 2.5 h according to the manufacturer’s protocol, we can observe that the median turnaround time of blood culture is longer than that of ddPCR.
Impact of empirical antibiotic therapy on the diagnostic performance of ddPCR
We categorised the samples based on whether patients received empirical antibiotic therapy, as illustrated in , and compared the impact of empirical antibiotic therapy on the diagnostic performance of ddPCR. In the empirical antibiotic therapy group, ddPCR exhibited a detection rate of 46.2% (24/52) for sepsis, which was not statistically different from the rate of 37.5% (18/48) observed in the non-empirical antibiotic therapy group (χ2 = 0.767; p = 0.381). We further comparing the sensitivity and specificity of ddPCR between the two groups, it was observed that in the empirical antibiotic therapy group, ddPCR exhibited a sensitivity of 78.6% (95%CI, 48.8,94.3) and a specificity of 65.8% (95%CI, 48.6,79.9) for diagnosing sepsis compared with blood culture. In the non-empirical antibiotic therapy group, ddPCR showed a sensitivity of 80.0% (95%CI, 50.7,99.4) and a specificity of 73.7% (95%CI, 56.6,86.0) (). No significant influence of empirical antibiotic therapy on the sensitivity and specificity of ddPCR diagnosis was observed (78.6% versus 80.0% and 65.8% versus 73.7%, respectively; p > 0.05).
Figure 4. Flow chart for patient based on whether empirical antimicrobial treatment was administered. EAT group: empirical antimicrobial treatment was administered for >48 hours; Non-EAT group: empirical antimicrobial treatment was administered for ≤48 hours; FGS: first generation sequencing.
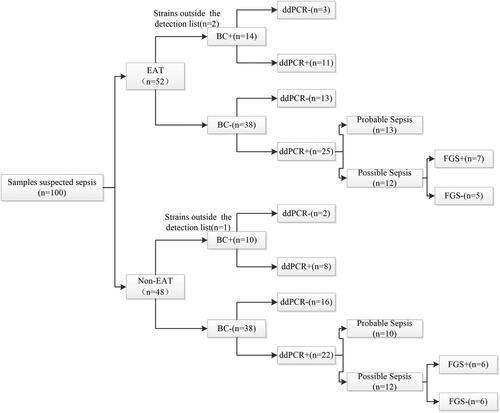
Table 5. The impact of empirical antimicrobial treatment on the sensitivity and specificity of ddPCR in diagnosing sepsis.
Due to ddPCR's capability for quantifying the copy numbers of target pathogen genes, we further examined whether empirical antibiotic therapy affected the quantitative results of ddPCR in the 19 BC+/ddPCR + samples. Among these, 11 belonged to the empirical antibiotic therapy group with a median pathogen gene copy number of 15,140 copies/ml (IQR, 1,919, 214,122), while the non-empirical antibiotic therapy group consisted of 8 samples with a median pathogen gene copy number of 20,955 copies/ml (IQR, 6195.75,64,825.5). Although the median copy number was higher in the non-empirical antibiotic therapy group than the empirical antibiotic therapy group, no statistically significant difference in pathogen gene copy numbers was observed between the two groups (Z = −0.165, p = 0.869) ().
Evaluation of antimicrobial resistance gene detection by ddPCR
In our study, we used the AMR panel to detect three most common antimicrobial resistance genes in clinical practice including blaKPC, mecA and blaNDM. In a total of 13 samples, we detected antimicrobial resistance genes as shown in the . Among these, 6 samples were detected the blaKPC gene, and 1 sample was detected the blaNDM gene. Further analysis revealed that whenever the ddPCR detected the carbapenemase-resistant genes blaKPC or blaNDM, they corresponded to the cultivation of carbapenem-resistant gram-negative bacteriain either the patient’s blood or extra-blood samples. The mecA gene was detected in 7 samples. In No 6,53 and 94 samples, the resistance gene matched the results of the susceptibility test. In No 40 sample, only the mecA gene was detected, and no bacterial species were identified in ddPCR.
Table 6. AMR genes detected by ddPCR and the related pathogens detected by blood culture and all microbiological testing.
The value of ddPCR in the dynamic surveillance of sepsis
In order to understand the value of ddPCR's quantitative detection capability in the dynamic monitoring of sepsis, we conducted dynamic ddPCR monitoring for six patients (No. 1, 27, 31, 32, 74, 84) who had positive blood cultures. Patients who initially tested blood culture and ddPCR positive underwent repeat blood monitoring on the 3rd and 6th days. We compared the detected pathogen copy numbers with clinically relevant infection indicators, including white blood cell count, C-reactive protein, and procalcitonin. It can be observed that the quantitative results of ddPCR generally align with the trends seen in C-reactive protein and procalcitonin levels. However, there were variations in the trends when compared to changes in white blood cell count ().
Discussion
ddPCR as a next-generation culture-independent molecular diagnostic technique, utilises real-time polymerase chain reaction or microarray-based methods to detect circulating cell-free DNA (cfDNA) in body fluids like blood. It has been developed as a novel approach for the precise detection and absolute quantification of nucleic acids without the necessity of generating a calibration curve [Citation13]. cfDNA molecules originate from dying cells and from colonising or invading microorganisms, which release DNA fragments into the blood as they break down, and their detection has emerged as a powerful non-invasive diagnostic tool in the fields of prenatal screening, transplantation, and oncology [Citation14, Citation15]. An increasing number of studies have also indicated that microbial cfDNA detection may enable the reliable identification of various infections [Citation16, Citation17]. Compared to other PCR-based pathogen diagnostic methods, ddPCR exhibits higher sensitivity and specificity. In our study, it achieved a sensitivity of 75.0% and a specificity of 69.7%. In contrast, earlier methods such as SeptiFast (Roche), Magicplex (Seegene), and TaqMan array card assay (Academy of Military Medical Science, Beijing, China) showed promise in rapidly identifying pathogens and guiding early targeted antibiotic therapy, but their low sensitivities, ranging from 29% to 79.4%, limit their clinical application [Citation18]. In the study conducted by Wu et al., ddPCR was applied to detect pathogens in suspected bloodstream infection patients, they found that ddPCR exhibited a sensitivity of 72.5% and specificity of 63.1% compared to blood culture [Citation11]. Similarly, Yannick et al. reported that ddPCR had a sensitivity of 80% and specificity of 87% for diagnosing suspected bloodstream infections [Citation19]. These findings, consistent with our study, collectively demonstrate the high sensitivity and specificity of ddPCR for pathogen diagnosis.
In our study, among the six samples classified as BC+/ddPCR-, three exhibited positive blood cultures for pathogens not included in the detection panel, namely Burkholderiaenocepacia, Pantoeaagglomerans, and Staphylococcus warneri. However, ddPCR failed to detect two instances of Serratia marcescens and one instance of Proteus mirabilis, all within the detection panel. Upon scrutinising our study results, we noted that the observed discrepancies may be related to the time interval between the collection of blood culture samples and ddPCR samples. All three patients were admitted to the intensive care unit after blood culture sampling in the emergency department, with ddPCR samples collected after their admission to the intensive care unit. It is possible that transient bacteraemia in patients at different blood sampling time points may have contributed to the observed differences in results. Furthermore, we acknowledge the possibility of potential issues concerning the sensitivity of the ddPCR detection system itself. Regarding the BC-/ddPCR + subset of samples, most earlier studies extrapolated their clinical diagnoses on clinical presentations and the results of cultures from samples other than blood [Citation11, Citation12]. In this subset of samples clinically diagnosed as possible sepsis, where no pathogens were cultured in blood or other fluid samples, there is currently no consensus on the interpretation of results. This uncertainty may be attributed to several factors, including the presence of nonviable, nonproliferating, or transient bacteraemia, intracellular organisms within circulating phagocytic cells, inhibition of bacterial growth by antibiotics, or possible contamination [Citation12]. To provide further insights into the results of this subset, we conducted exploratory attempts by subjecting these samples to sanger sequencing. The results revealed that among the 24 blood samples clinically diagnosed as possible sepsis, 13 were consistent with the ddPCR results. The inconsistency may be due to the failure of first-generation sequencing, as it is not as sensitive as ddPCR, even though nested PCR was used to enhance the sensitivity of first-generation sequencing in our study. In this subset of sepsis samples lacking cultivation evidence, there may be cases where antibiotics inhibited bacterial growth, leading to negative cultures, while there was indeed a pathogen infection. This implies that ddPCR may have a higher actual sensitivity and specificity for sepsis diagnosis than previously believed.
In addition to its higher sensitivity and specificity, ddPCR, as a molecular diagnostic tool, significantly shortens the time required to obtain pathogen information. In our study, the median turnaround time for obtaining pathogen and drug resistance information from all blood culture-positive samples was 47.5 h. In contrast, ddPCR, which is already a standardised process, requires only 2.5 h according to the protocol. Earlier reports of ddPCR turnaround times ranged from 2.5 to 11 h [Citation11, Citation20, Citation21], all significantly faster than traditional culture times. This demonstrates that the ddPCR outperforms blood culture in terms of specificity, sensitivity, and turnaround time. It represents a promising approach for the early and accurate diagnosis of sepsis or bloodstream infections.
As we known that empiric antibiotic therapy can reduce the positivity rate of blood culture in sepsis. Scheer et al. conducted a study and found that blood culture positivity was 50.6% among patients with sepsis who did not receive antibiotics, but it was only 27.7% in those who were already receiving antibiotics [Citation22]. However, the impact of antibiotic therapy on the diagnostic efficiency of ddPCR is not well understood. In our study, we categorised the collected sepsis samples into two groups: those who received empiric antibiotic therapy and those who did not. The results showed that there was no statistically significant difference in the detection rate, sensitivity, and specificity of ddPCR between the two groups. This suggests that, compared to culture methods, the diagnostic efficiency of ddPCR may be less affected by antibiotic therapy. The study conducted by Lin et al., found that antibiotic therapy did not affect the detection rate of ddPCR but did lower its sensitivity and specificity [Citation21]. This finding differs slightly from the results of our study. It’s possible that variations in patient populations, antibiotic regimens, or other factors may contribute to these differences. To gain a more comprehensive understanding of the impact of antibiotic therapy on ddPCR's diagnostic performance, further research with larger sample sizes and multi-centre studies may be necessary. This would help confirm whether there are specific factors that influence ddPCR's performance in the presence of antibiotic treatment and provide more robust insights into its clinical utility in different scenarios. ddPCR not only enables rapid and sensitive detection of the pathogens responsible for sepsis but also has the potential to identify bacterial resistance genes to some extent. In our study, we utilised probes and targets for common resistance genes like blaKPC, mecA, and blaNDM, based on resistance trends among clinical isolates in China [Citation23]. In all cases where carbapenem resistance genes were detected, carbapenem-resistant Gram-negative bacteria were also identified in blood or extra-blood site samples, this suggests that ddPCR can provide clinicians with early information about pathogen resistance to some extent. However, it’s important to note that bacterial resistance mechanisms are diverse, and enzymatic mechanisms represent only one type [Citation24], therefore, ddPCR cannot completely replace traditional antimicrobial susceptibility testing but can serve as a complementary tool. In the 40th sample, only the mecA gene was detected, and no bacterial species were identified in ddPCR. This discrepancy may be due to the presence of methicillin-resistant Staphylococcus species beyond the pathogen detection list of ddPCR, resulting in the detection of only the resistance genes without the identification of the specific bacterial species.
Lastly, due to ddPCR allows for quantitative measurement of pathogen copy numbers, previous research has indicated that quantifying microbial burden using ddPCR can assess the severity and prognosis of sepsis [Citation25, Citation26]. In our study, we primarily explored the clinical significance of pathogen quantification in the dynamic monitoring of sepsis. By dynamically monitoring ddPCR results in six sepsis patients with positive blood cultures, we observed that the quantitative results of ddPCR generally align with the trends seen in C-reactive protein and procalcitonin levels. This suggests that ddPCR may be used for dynamic monitoring of pathogen load, like conventional inflammatory infection indicators, to reflect changes in infection status. The benefit of monitoring clinical progression determining DNA load was also demonstrated by Bialasiewicz et al. in that study, the authors assessed the effectiveness of antibiotic therapy by quantifying bacterial DNA load at day zero and five, showing a great decrease in DNA load over time [Citation27]. However, further validation is needed to confirm the role of ddPCR in quantifying pathogen load in clinical practice, including its potential for stratifying severity, assessing prognosis, and guiding treatment decisions.
This study is subject to certain limitations. Firstly, due to the inherent limitations of ddPCR, it can only detect the pathogens included in the ddPCR panels. Pathogens beyond the scope of detection cannot be identified, and it is incapable of distinguishing between viable and non-viable microorganisms, which is a constraint shared by all PCR-based methods. Additionally, although ddPCR can detect resistance genes, it can not completely replace conventional antimicrobial susceptibility testing. Secondly, our sample size was relatively small, consisting of only 100 sepsis events. Despite our efforts to minimise biases in study design and analysis, the limited sample size restricted our ability to conduct in-depth analyses within specific subgroups and to generalise our findings to a broader patient population. Moreover, our study was conducted at a single medical centre, potentially introducing geographic and demographic limitations. Patients from different regions or populations may exhibit distinct characteristics that could impact the external validity of our study. Lastly, concerning the clinical application of ddPCR for quantitative pathogen detection, we only conducted preliminary exploration due to sample size constraints. Further validation is needed to confirm the role of ddPCR in quantifying pathogen load in clinical practice. This includes assessing its potential for stratifying severity, assessing prognosis, and guiding treatment decisions.
In conclusion, compared to blood culture, ddPCR exhibited higher sensitivity for pathogen diagnosis in suspected septic patients, and it provided pathogen and drug resistance information in a short time. The quantitative results of ddPCR generally aligned with the trends seen in C-reactive protein and procalcitonin levels, indicating that ddPCR can serve as a dynamic monitoring tool for pathogen load in septic patients. For a more comprehensive understanding of its clinical value, larger-scale and multicentre studies are needed.
Author contributions
Hua Zhou, Yunsong Yu, Zhenping Wu, Xi Li, Jiang Xia contributed in the design, data collection and analysis, and writing of the manuscript. Zhenping Wu, Yake Yao, Hongliu Cai, Guobin Wang, Wenqiao Yu, Qi Chen, Hui Lou, Zhu Zeng contributed to the data collection, data analysis, diagnosis and treatment of patients. Xi Li, Hao Yu, Jiang Xia conducted experimental operations. Hua Zhou, Yunsong YU, Yake Yao, Xi Li contributed to the data results interpretation and revision of the manuscript.
Supplemental Material
Download MS Word (409.2 KB)Disclosure statement
The authors report there are no competing interests to declare.
Additional information
Funding
References
- Kumar A, Roberts D, Wood KE, et al. Duration of hypotension before initiation of effective antimicrobial therapy is the critical determinant of survival in human septic shock. Crit Care Med. 2006;34(6):1589–1596. doi:10.1097/01.CCM.0000217961.75225.E9.
- Evans L, Rhodes A, Alhazzani W, et al. Surviving sepsis campaign: international guidelines for management of sepsis and septic shock 2021. Intensive Care Med. 2021;47(11):1181–1247. doi:10.1007/s00134-021-06506-y.
- Timsit J-F, Ruppé E, Barbier F, et al. Bloodstream infections in critically ill patients: an expert statement. Intensive Care Med. 2020;46(2):266–284. doi:10.1007/s00134-020-05950-6.
- Kojabad AA, Farzanehpour M, Galeh HEG, et al. Droplet digital PCR of viral DNA/RNA, current progress, challenges, and future perspectives. J Med Virol. 2021;93(7):4182–4197. doi:10.1002/jmv.26846.
- Postel M, Roosen A, Laurent-Puig P, et al. Droplet-based digital PCR and next generation sequencing for monitoring circulating tumor DNA: a cancer diagnostic perspective. Expert Rev Mol Diagn. 2018;18(1):7–17. doi:10.1080/14737159.2018.1400384.
- Poulet G, Massias J, Taly V. Liquid biopsy: general concepts. Acta Cytol. 2019;63(6):449–455. doi:10.1159/000499337.
- Chen B, Jiang Y, Cao X, et al. Droplet digital PCR as an emerging tool in detecting pathogens nucleic acids in infectious diseases. Clin Chim Acta. 2021;517:156–161. doi:10.1016/j.cca.2021.02.008.
- Levy MM, Evans LE, Rhodes A. The surviving sepsis campaign bundle: 2018 update. Crit Care Med. 2018;46(6):997–1000. doi:10.1097/CCM.0000000000003119.
- Mehta NM, Halwick DR, Dodson BL, et al. Potential drug sequestration during extracorporeal membrane oxygenation: results from an ex vivo experiment. Intensive Care Med. 2007;33(6):1018–1024. doi:10.1007/s00134-007-0606-2.
- Poirel L, Walsh TR, Cuvillier V, et al. Multiplex PCR for detection of acquired carbapenemase genes. Diagn Microbiol Infect Dis. 2011;70(1):119–123. doi:10.1016/j.diagmicrobio.2010.12.002.
- Wu J, Tang B, Qiu Y, et al. Clinical validation of a multiplex droplet digital PCR for diagnosing suspected bloodstream infections in ICU practice: a promising diagnostic tool. Crit Care. 2022;26(1):243. doi:10.1186/s13054-022-04116-8.
- Nguyen MH, Clancy CJ, Pasculle AW, et al. Performance of the T2Bacteria panel for diagnosing bloodstream infections: a diagnostic accuracy study. Ann Intern Med. 2019;170(12):845–852. doi:10.7326/M18-2772.
- Huggett JF, Cowen S, Foy CA. Considerations for digital PCR as an accurate molecular diagnostic tool. Clin Chem. 2015;61(1):79–88. doi:10.1373/clinchem.2014.221366.
- Aravanis AM, Lee M, Klausner RD. Next-Generation sequencing of circulating tumor DNA for early cancer detection. CELL. 2017;168(4):571–574. doi:10.1016/j.cell.2017.01.030.
- Bloom RD, Bromberg JS, Poggio ED, et al. Cell-Free DNA and active rejection in kidney allografts. J Am Soc Nephrol. 2017;28(7):2221–2232. doi:10.1681/ASN.2016091034.
- Han D, Li R, Shi J, et al. Liquid biopsy for infectious diseases: a focus on microbial cell-free DNA sequencing. Theranostics. 2020;10(12):5501–5513. doi:10.7150/thno.45554.
- Eichenberger EM, Degner N, Scott ER, et al. Microbial cell-Free DNA identifies the causative pathogen in infective endocarditis and remains detectable longer than conventional blood culture in patients with prior antibiotic therapy. Clin Infect Dis. 2023;76(3):e1492-500–e1500. doi:10.1093/cid/ciac426.
- Zheng Y, Jin J, Shao Z, et al. Development and clinical validation of a droplet digital PCR assay for detecting Acinetobacter baumannii and Klebsiella pneumoniae in patients with suspected bloodstream infections. Microbiologyopen. 2021;10(6):e1247. doi:10.1002/mbo3.1247.
- Wouters Y, Dalloyaux D, Christenhusz A, et al. Droplet digital polymerase chain reaction for rapid broad-spectrum detection of bloodstream infections. Microb Biotechnol. 2020;13(3):657–668. doi:10.1111/1751-7915.13491.
- Hu B, Tao Y, Shao Z, et al. A comparison of blood pathogen detection among droplet digital PCR, metagenomic Next-Generation sequencing, and blood culture in critically ill patients with suspected bloodstream infections. Front Microbiol. 2021;12:641202. doi:10.3389/fmicb.2021.641202.
- Lin K, Zhao Y, Xu B, et al. Clinical diagnostic performance of droplet digital PCR for suspected bloodstream infections. Microbiol Spectr. 2023;11(1):e137822. doi:10.1128/spectrum.01378-22.
- Scheer CS, Fuchs C, Gründling M, et al. Impact of antibiotic administration on blood culture positivity at the beginning of sepsis: a prospective clinical cohort study. Clin Microbiol Infect. 2019;25(3):326–331. doi:10.1016/j.cmi.2018.05.016.
- Hu F-P, Guo Y, Zhu D-M, et al. Resistance trends among clinical isolates in China reported from CHINET surveillance of bacterial resistance, 2005-2014. Clin Microbiol Infect. 2016;22(Suppl 1): S9–S14. doi:10.1016/j.cmi.2016.01.001.
- Tenover FC. Mechanisms of antimicrobial resistance in bacteria. Am J Med. 2006;119(6 Suppl 1):S3–S10. discussion S62-70. doi:10.1016/j.amjmed.2006.03.011.
- Ziegler I, Cajander S, Rasmussen G, et al. High nuc DNA load in whole blood is associated with sepsis, mortality and immune dysregulation in Staphylococcus aureus bacteraemia. Infect Dis (Lond). 2019;51(3):216–226. doi:10.1080/23744235.2018.1562205.
- Ziegler I, Lindström S, Källgren M, et al. 16S rDNA droplet digital PCR for monitoring bacterial DNAemia in bloodstream infections. PLoS One. 2019;14(11):e224656. doi:10.1371/journal.pone.0224656.
- Bialasiewicz S, Duarte TPS, Nguyen SH, et al. Rapid diagnosis of Capnocytophaga canimorsus septic shock in an immunocompetent individual using real-time nanopore sequencing: a case report. BMC Infect Dis. 2019;19(1):660. doi:10.1186/s12879-019-4173-2.