Abstract
Geographic isolation has been proposed as the factor driving subspecific diversity of the Ecuadorian hillstar (Oreotrochilus chimborazo), a highland species restricted to the naturally fragmented paramos of Ecuador and southern Colombia. Current taxonomy recognizes three subspecies: O. c. chimborazo (from the Chimborazo volcano), O. c. soderstromi (from the Quilotoa volcano), and O. c. jamesonii (along the paramos of Ecuador and southern Colombia). To understand the origin of this morphological diversity, we explored the genetic variation along the species range based on analyses of two mitochondrial genes (ND2 and ND4), and one nuclear intron (MUSK). Subspecies O. c. soderstromi was not included in the analysis, as it was not registered at or around its type locality, the Quilotoa volcano. Instead, only O. c. jamesonii was encountered in that area. We found no evidence of genetic structure corresponding to subspecies or physiographic units, aside from some inconclusive evidence in putatively isolated populations. Ecological niche modeling predicted continuous and homogeneous environmental space between the two volcanos, and field expeditions showed evidence of a potential contact zone between O. c. jamesonii and O. c. chimborazo. Also, our data suggest that the only specimen described as O. c. soderstromi may have been an intergrade. We discuss our results in the light of possible range shifts in the past, resulting from climatic fluctuations around the Pleistocene–Holocene transition.
El aislamiento geográfico ha sido propuesto como el factor más importante en la generación de diversidad subespecífica en la estrellita ecuatoriana (Oreotrochilus chimborazo), un ave de los altos Andes restringida a los páramos naturalmente fragmentados de Ecuador y el sur de Colombia. La taxonomía actual reconoce tres subespecies: O. c. chimborazo (en el volcán Chimborazo), O. c. soderstromi (en el volcán Quilotoa) y O. c. jamesonii (a lo largo de los páramos de Ecuador y el sur de Colombia). Con el fin de comprender el origen de esta diversidad morfológica, exploramos la variación genética a lo largo del rango de la especie con base en los análisis de dos genes mitocondriales (ND2 y ND4) y un intrón nuclear (MUSK). La subespecie O. c. soderstromi no fue incluida en el análisis, porque no se registraron individuos de este taxón en su localidad tipo, el volcán Quilotoa, donde solo confirmamos la presencia de O. c. jamesonii. No encontramos evidencia de estructura genética correspondiente a las subespecies o a unidades fisiográficas de páramo, a excepción de una posible estructuración genética probablemente debida a poblaciones presumiblemente aisladas. Análisis de modelamiento de nicho ecológico predijeron que el espacio ambiental entre los dos volcanes es continuo y homogéneo, mientras que los resultados de expediciones de campo sugieren la existencia de una zona de contacto entre O. c. jamesonii y O. c. chimborazo. Además, nuestros datos apoyan la idea de que el único espécimen descrito como O. c. soderstromi puede ser un híbrido. Discutimos nuestros resultados en el contexto de posibles cambios de rango de distribución en el pasado, como resultado de las fluctuaciones climáticas que se dieron en el periodo de transición entre el Pleistoceno y el Holoceno.
Introduction
Tropical mountain ranges have probably hosted more events of bird diversification in geologically recent times than any other geographic area of comparable extension.[Citation1] By establishing elevational gradients of temperature and precipitation, these landscapes promote a diversity of ecosystems ranging from tropical forests and savannahs, near the base, to tundra-like grasslands, near the top, with each ecosystem having its share of specialized birds. To add another layer of complexity to this process of diversification, the rugged topography of mountains promotes the generation of dispersal barriers, leading to divergence by geographic isolation. In the Andes, for example, deep, dry valleys act as dispersal barriers for birds in the cloud forest, and often, closely related and ecologically similar species replace each other on either side of the barrier.[Citation2–4] High-elevation ecosystems tend to be more restricted in area, and thus their inhabitants are more likely to diverge in response to habitat fragmentation.[Citation5,6] In extreme cases, habitats are restricted to the slopes of outstanding mountain peaks, as probably happens with the habitat of some populations of the Ecuadorian hillstar (Oreotrochilus chimborazo), a hummingbird with one of the highest elevational ranges in the world [Citation7] that seems to be undergoing rapid evolutionary divergence.
Ecuadorian hillstars have a geographic range restricted to the paramos of Ecuador and extreme southern Colombia.[Citation8,9] Within this small range, three subspecies have been described: O. c. chimborazo, O. c. jamesonii, and O. c. soderstromi [Citation8,10,11] (Figure (A)). Subspecies O. c. chimborazo and O. c. soderstromi are described in the literature as being endemic to the volcanoes Chimborazo and Quilotoa, [Citation8,9,12] respectively (Figure (A)). The soil on the slopes and surrounding areas of these volcanoes are barren and consists of fine, volcanic sediment.[Citation13] Among the few scattered bushes that thrive under these conditions is Chuquiraga jussieui (Asteraceae), a primary source of nectar for O. Chimborazo, which might limit the distribution of the hummingbird.[Citation11] This case of extreme habitat insularity has caught the attention of notable naturalists such as Alfred Russel Wallace, who wrote in his treatise Island Life that the ranges of these subspecies were among ‘the most wonderfully restricted.’[Citation10]
Figure 1. Geographic range of Oreotrochilus chimborazo. (A) Range as described in the literature.[Citation8,9,11,14,15,17,18] Recent pictures [Citation20] taken in Quilotoa show individuals clearly identifiable as males of O. c. chimborazo and O. c. jamesonii, thereby putting in doubt the geographic isolation between these subspecies. (B) Localities visited during this study. Localities 12, 13, and 14 were those visited around Quilotoa and Chimborazo and in the intervening páramo between both volcanoes. (C) Predicted distribution of O. c. chimborazo based on ecological niche modeling using data from WorldClim [Citation36] and remote sensing. The localities in which O. chimborazo was found between volcanoes Quilotoa and Chimborazo were not used during modeling to test whether occurrence in that region could be predicted using information from the other localities only. MP, Minimum Presence threshold provided by MaxEnt analysis. Localities, numbered according to latitude starting at the northernmost: 1, Mt. El Chiles; 2, El Ángel Ecological Reserve; 3, Mt. Cotacachi; 4, lakes of Mojanda; 5, Mt. Cayambe; 6, Mt. Pichincha; 7, Papallacta Pass; 8, Antisana Ecological Reserve; 9, Mt. Cotopaxi; 10, Mt. Illinizas; 11, Quilotoa crater; 12, Apagua mountain ridge; 13, Mountains around Mula Corral reservoir; 14, Mountains around Simiatug; 15, Cunungyacu (near Mt. Chimborazo); 16, Culebrillas lake; 17, Mountains around Migüir; 18, Lagunillas mountain ridge.
![Figure 1. Geographic range of Oreotrochilus chimborazo. (A) Range as described in the literature.[Citation8,9,11,14,15,17,18] Recent pictures [Citation20] taken in Quilotoa show individuals clearly identifiable as males of O. c. chimborazo and O. c. jamesonii, thereby putting in doubt the geographic isolation between these subspecies. (B) Localities visited during this study. Localities 12, 13, and 14 were those visited around Quilotoa and Chimborazo and in the intervening páramo between both volcanoes. (C) Predicted distribution of O. c. chimborazo based on ecological niche modeling using data from WorldClim [Citation36] and remote sensing. The localities in which O. chimborazo was found between volcanoes Quilotoa and Chimborazo were not used during modeling to test whether occurrence in that region could be predicted using information from the other localities only. MP, Minimum Presence threshold provided by MaxEnt analysis. Localities, numbered according to latitude starting at the northernmost: 1, Mt. El Chiles; 2, El Ángel Ecological Reserve; 3, Mt. Cotacachi; 4, lakes of Mojanda; 5, Mt. Cayambe; 6, Mt. Pichincha; 7, Papallacta Pass; 8, Antisana Ecological Reserve; 9, Mt. Cotopaxi; 10, Mt. Illinizas; 11, Quilotoa crater; 12, Apagua mountain ridge; 13, Mountains around Mula Corral reservoir; 14, Mountains around Simiatug; 15, Cunungyacu (near Mt. Chimborazo); 16, Culebrillas lake; 17, Mountains around Migüir; 18, Lagunillas mountain ridge.](/cms/asset/59890d36-80cb-4e8b-b551-338a0f2986e2/tneo_a_1155280_f0001_oc.gif)
Since the publication of Island Life, however, important findings about the distribution of O. chimborazo have challenged the assumption that these hummingbirds are restricted to volcanic peaks. The subspecies with the most widespread distribution, O. c. jamesonii, is now known to also occur in grassland paramo, outside the slopes of volcanoes but still in places where Chuquiraga grows (Figure (A)).[Citation11,14–18] Dependence on volcanic slopes is still being proposed as the factor driving divergence between hillstars living in Quilotoa and Chimborazo.[Citation8,9] Nevertheless, grassland paramo stretches between both volcanoes,[Citation19] at elevations above the minimum that at which O. c. jamesonii has been recorded elsewhere in Ecuador. Therefore, unless the subspecies at Quilotoa and Chimborazo were restricted to volcanoes, and thus differ ecologically from O. c. jamesonii, no reason exists to claim that birds belonging to such subspecies are isolated. In fact, the possibility of contact between subspecies of O. chimborazo has been suggested even in the description of subspecies O. c. soderstromi,[Citation12] upon the realization that morphological characteristics that define O. c. soderstromi are consistent with what would be expected of an intergrade between the other two subspecies.[Citation8,9,12]
All three subspecies are recognized on the basis of a single character, the color of the gorget in males: in O. c. jamesonii, it is violet-blue, the same color as that of the rest of the head; in O. c. chimborazo, it is bright green; and in O. c. soderstromi, the gorget is violet-blue with a few interspersed green feathers [Citation8,9,12] (Figure (A)). The intermediate plumage and geographic distribution of O. c. soderstromi thus fits with the description of an intergrade, but such hypothesis did not surface because it was assumed that hillstars from Quilotoa were isolated from hillstars elsewhere.[Citation8,9] Moreover, recent photographic evidence shows males of O. c. jamesonii and O. c. chimborazo in Quilotoa,[Citation20] providing some preliminary evidence that both subspecies occur in the area.
In spite of the possibility of limited contact and intergradation, how did these differentiated forms came into being? Divergence under sympatry is among the scenarios least favored by evolutionary models [Citation21,22]; a more likely scenario is divergence in allopatry caused by a geographic barrier that is non-existent in the present. Furthermore, geographic isolation might be affecting genetic structure within O. c. jamesonii. The distribution of this subspecies extends across all grassland paramo in Ecuador (Figure (B)), but is intersected by deep dry valleys. Some of these valleys, such as those of the Mira and Chanchán rivers, have been proposed to be barriers of dispersal for birds.[Citation9] To understand how divergence by isolation may occur in relatively short evolutionary times within Oreotrochilus in particular and paramo birds in general, it is worth testing whether geographic isolation might be affecting genetic structure within O. c. jamesonii.
Phylogeography, as the study of the relation between geography and phylogenetics is often named, may provide important insights on the evolutionary history of O. chimborazo. In past studies, phylogeography has been useful in confirming the role of dry Andean valleys as dispersal barriers,[Citation2,3,23,24] and has allowed researchers to uncover cryptic diversity [Citation24–29] and find correlations between sequences of speciation events and emergence of potential dispersal barriers.[Citation24] Most phylogeographic studies in the Andes have focused on the inhabitants of the cloud forests, but the paramo also offers promising case studies, such as that of the Ecuadorian hillstar. Thus, in this study, we used phylogeographic methods to test: (1) whether subspecies O. c. jamesonii and O. c. chimborazo represent diverging evolutionary lineages, and (2) whether O. c. jamesonii shows genetic structure along its geographic distribution. We also aimed to gain a better understanding of the distribution of O. chimborazo. To meet this goal, we conducted systematic visits to the most important ‘paramo islands’ in Ecuador, in addition to visits to volcanoes Quilotoa and Chimborazo, and three sites in between these two volcanoes, to explore whether subspecies of O. chimborazo are in contact. We expect this study to pioneer phylogeographic research of birds in the paramos of the northern Andes, and to present a model organism for the study of geographic isolation and divergence at its earliest stages.
Materials and methods
Methods
Fieldwork and specimen collection
We visited 17 localities to test for occurrence of O. chimborazo (Figure (B)). We included three localities situated between Quilotoa and Chimborazo, which we hypothesized to have suitable habitat for the species, against some claims from the literature.[Citation8,9] In selecting the 17 localities, we tried to visit at least one locality from each ‘paramo island’ in Ecuador (Figure (B)). A ‘paramo island’ is defined here as a set of regions topographically continuous between 3600 and 4800 m of elevation and separated from other paramos by more than 15 km. This last criterion of separation was chosen because it is the minimum width of valleys that have been hypothesized to be barriers for the dispersal of birds.[Citation30]
Collection of samples for genetic analysis was possible in 11 of the above-mentioned localities. Additionally, we visited one locality in southern Ecuador (Lagunillas mountain range) (Figure (B)) to obtain samples from O. estella, a closely related species to O. chimborazo.[Citation31,32] Specimens of Oreotrochilus were collected around patches of Chuquiraga jussieui in the paramo. Samples of pectoral muscle were used for genetic analysis (stored in 95% ethanol). Voucher specimens for most individuals are housed in the zoology museums of Pontificia Universidad Católica del Ecuador (QCAZ) and Universidad Tecnológica Indoamérica (MZUTI) (list of specimens provided in Table ). Pectoral muscle samples were frozen at −20 or −80 °C, and deposited at the same institutions.
Table 1. Samples of Oreotrochilus chimborazo and Oreotrochilus estella collected for this study. Museum abbreviations: QCAZ, Museo de Zoología de la Pontificia Universidad Católica del Ecuador; MZUTI, Museo de Zoología de la Universidad Tecnológica Indoamérica; UTI, Universidad Tecnológica Indoamérica (only for specimens for which no voucher is available). Sex codes: F, female; M, male; U, undetermined. Gene accession numbers are given in each gene column.
Species distribution analysis
We built an ecological niche model (ENM) for O. chimborazo and projected it back on to geography using MaxEnt.[Citation33,34] Occurrences used to build the model were localities visited during fieldwork and additional localities retrieved from the literature [Citation8,9,14,18] (Figure (C)). The database eBird [Citation35] was consulted to find additional localities, but we found none that were not already covered by the literature and our field work. In calibrating the ENM, we excluded the localities situated between Quilotoa and Chimborazo to test whether environmental information retrieved from other localities was sufficient to predict occurrence of these birds between the two volcanoes. Predicting occurrence in the left-out localities would provide evidence that the hillstars that we found in those localities, rather than being vagrant individuals, belong to populations thriving under suitable environmental conditions. To avoid spatial autocorrelation, we included only localities situated more than 5 km apart, a distance large enough to overpass small mountain ridges that may set boundaries to local climate. Upon applying this filter, 15 occurrences remained for the analysis (Figure (C)).
The environmental data-set used to build the ENM consisted of raster grids containing data from WorldClim [Citation36] on temperature and precipitation. The geographic area covered by the grids was defined by a continuous area that encompassed a 120-km buffer around presence localities: an area large enough to cover the known distribution of the species and to provide enough contrast between environmental conditions characterizing presence localities and background areas. We did not use a larger area representing a biogeographic zone,[see [Citation37]] because wide topographic depressions at the north and south of the hummingbird’s known distribution preclude the presence of immediately accessible areas to the species.[Citation8]
The resolution of the grids was of 1 km per pixel. Temperature was used in the ENM because of its strong correlation to elevation, and because using temperature, in contrast to using elevation, allows constructing a biologically meaningful description of potential occurrence. Precipitation was used because it was hypothesized to explain the occurrence of arid environments, which may be preferred by the hillstars.[Citation11] WorldClim provided 19 variables derived from temperature and precipitation, but based on results from a preliminary run of MaxEnt we selected only six of those variables, which were those that had a relative contribution of at least 1% to the ENM in preliminary runs.
In addition to WorldClim data, six data-sets from remote satellite sensors were used to build the ENM: NDVI, the normalized vegetation index from NOAA-AVHRR [Citation38]; LAI1, LAI2, and LAI3, which are different measurements of leaf area index derived from the remote sensor MODIS [Citation39]; and two layers from the scatterometer QSCAT (http://www.scp.byu.edu/) containing data on microwave reflectance of land surface, which is informative on aridity of soils.[Citation40] These MODIS and QSCAT data were the same as the ones used in a previous study [Citation40] to model the potential distribution of South American birds. Measurements derived from MODIS corresponded to averages of monthly data taken between 2000 and 2004. Measurements derived from QSCAT corresponded to the average and standard deviation of three-day composite images for the year 2001.
We used MaxEnt 3.3.3 k to model the species distribution, with the following settings: convergence threshold set to 1 × 10−5, maximum number of background points to 104, maximum iterations to 500, and the regularization parameter set to ‘auto.’ Equal training and minimum presence thresholds were used to filter the ENM. A map of potential occurrence of O. chimborazo was created by projecting the filtered ENM back on to geography. Support for the ENM was tested using a Pearson cross-validation test, which is recommended for ENMs built with fewer than 25 known occurrences.[Citation41] (see [Citation42] for a justification of not using AUC curve).
Sample preparation for genetic analysis
DNA was extracted following a protocol based on guanidinine thiocyanate lysis and isopropanol precipitation.[Citation43] Three regions were amplified: subunits 2 and 4 of the mitochondrial DNA (mtDNA) gene Nicotinamide Dehydrogenase (ND2 and ND4, respectively), and the third intron of the Muscle-specific Kinase gene (MUSK). The amplification product for ND4 also included flanking tRNA-coding regions. Amplification primers were used as follows: for ND2, primers 5219 and 6313 [Citation44]; for ND4, primers ND4 and LEU [Citation45]; and, for MUSK, primers MUSK I3F and MUSK I3R.[Citation46] A standard protocol was followed for amplification of ND2, a step-down protocol for that of ND4, and a touch-down protocol for that of MUSK (details available upon request). Unincorporated primers and dNTPs were degraded with Exo-Sap-IT® (Affimetrix). PCR products and PCR primers were processed in Macrogen Inc. (South Korea) for sequencing using BigDye® Terminator chemistry (Applied Biosystems). In Macrogen Inc., sequencing reaction products were purified through precipitation with ethanol and resolved on an ABI 3730XL (Applied Biosystems) genetic analyzer. Back in our lab, sequence chromatograms were edited using Sequencher 4.1 (Gene Codes Corporation 2007) to correct for discrepancies between the forward and reverse chains. The forward and reverse chains were aligned automatically using CLUSTAL X.[Citation47]
Phylogenetic analyses and hypothesis testing
Genetic variability was summarized using haplotype (h) and nucleotide (π) diversity indicators in DNAsp.[Citation48] To visualize genetic variability in geographic space, a haplotype network was built in TCS 2.1 [Citation49] under maximum parsimony and 95% of connectivity (gaps coded as missing data). Haplotype frequency pie charts for mtDNA data were drawn over corresponding localities. Phylogenetic trees were inferred from a data-set consisting of the sequences generated here, plus outgroup sequences retrieved from GenBank. The outgroup included other species of Oreotrochilus (accession numbers: O. estella, AY830507.1,[Citation50] EU042327.1 [Citation32]; O. melanogaster, GU166854.1,[Citation51], GU166866.1 [Citation51]), and Ramphomicron microrhynchum (accession number: R. microrhynchum, EU042266.1,[Citation32] EU042587.1 [Citation32]).
Phylogenetic inference was obtained by maximum likelihood (ML) and Bayesian inference (BI) using the software GARLI 2.01 [Citation52] and Phycas,[Citation53] respectively. The partitioning scheme and models of sequence evolution used in both ML and BI were selected using PartitionFinder [Citation54] under the Bayesian Information Criterion. The best-fit partitioning scheme (BIC = 7290.7421) consisted of the following subset partitions: (1) codon 2 of ND2 + codon 1 of ND4, (2) codon 3 of ND2 + codon 2 of ND4, and (3) codon 1 of ND2 + codon 3 of ND4 + tRNAs flanking ND4. The best-fit models of molecular evolution for these subsets were, respectively: (1) F81,[Citation55] (2) K81uf,[Citation56] and (3) HKY [Citation57] + I.
In ML and BI, data-sets of mtDNA (ND2 + ND4) and of MUSK were first analyzed separately and then combined. Support for the ML trees was estimated from 100 bootstrap repetitions performed in GARLI. The bootstrap trees were then used to build a 50% majority-rule consensus tree in Mesquite.[Citation58] A clade was considered to be supported if it was found in 70% of the bootstrap trees. BI proceeded with priors set to uninformative values, except for the inclusion of a polytomy prior, which is recommended to avoid the ‘Bayesian star tree paradox’ problem.[Citation53] For each data-set, a Markov chain Monte Carlo (MCMC) was run for 10 thousand generations, with sampling occurring once every 100 generations. The first 1000 trees were discarded as burn-in. Stationarity of the MCMC chain was confirmed by visual inspection (plotting functions in R [Citation59]) of the marginal likelihood at each sampled generation. The remaining trees were summarized into a 50% majority rule consensus tree in Mesquite.[Citation58] A clade was considered to be supported if it was found within 95% of the trees drawn from the posterior distribution.
To explore whether our data support the hypothesis of genetic differentiation between individuals identified as O. c. chimborazo and O. c. jamesonii, we applied a hypothesis test based on the results of the Bayesian analysis. In PAUP, we took the post burning-in trees from the posterior probability distribution and filtered all trees that portrayed: (1) both O. c. chimborazo and O. c. jamesonii as monophyletic (not necessarily as sister taxa), or (2) any of them as monophyletic. The total of trees retained by the filter divided by the total number of post burn-in trees indicated the posterior probability that any of these hypotheses is correct (conditional on the model, data, prior probabilities, and convergence of the MCMC). [Citation62]
Molecular analysis of variance
We used an molecular analysis of variance (AMOVA) [Citation60] to test for genetic structure among subspecies and populations living across paramo islands. The analysis was conducted using Arlequin 3.5.[Citation61] In the AMOVA hierarchy, groups were the subspecies O. c. chimborazo and O. c. jamesonii and demes were paramo islands. Because O. c. chimborazo is found in only one island, Quilotoa-Chimborazo, the test for genetic differences among islands within subspecies is effectively a test of differences among islands within O. c. jamesonii. Probability values were estimated from permutation analyses, as in standard AMOVA.
Results
New records
We recorded O. chimborazo in six localities where it was previously unreported (Figure (B), see arrows). At each of these localities, the hillstars were found visiting Chuquiraga jussieui bushes. Three of these localities were situated between Quilotoa and Chimborazo, thereby confirming that hillstars occur in the paramo extending between both volcanoes. Males in each of the three localities were identified as either O. c. jamesonii or O. c. chimborazo; however, we never registered both subspecies in the same site. In Apagua, the northernmost of the three localities, 10 adult males (observed) and the 5 males (captured) belonged to O. c. jamesonii. In the mountains around Mula Corral, intermediate in latitude between the other two, we identified five males as O. c. jamesonii. In the mountains around Simiatug, the southernmost locality (closer to Chimborazo volcano), the only adult male for which we had good views of its gorget belonged to O. c. chimborazo. In Mula Corral strong winds precluded the collection of specimens. In Simiatug, only a juvenile male was captured, which could not be identified to subspecies because of its age.
We could not confirm the presence of either O. c. chimborazo or O. c. jamesonii in Quilotoa. We captured a female Ecuadorian hillstar in that site, but as discussed previously, females cannot be identified to either subspecies. Chuquiraga was abundant at the site, but the bushes were mostly visited by other species of hummingbirds (e.g. Lesbia victoriae) which tend to live at lower elevations than O. chimborazo. Quilotoa, however, was visited for fewer than 2 days, and given previous reports of the occurrence of both subspecies,[Citation20] the site clearly deserves longer visits in future studies.
Ecological niche modeling
Percentages of contribution of each environmental variable to the ENM were as follows: mean temperature of the coldest quarter, 50.8%; mean temperature of the warmest quarter, 22.5%; mean temperature of the driest quarter, 16.7%; minimum temperature of the coldest month, 3.1%; precipitation of the warmest quarter, 2.2%; NDVI7, 1.8%; maximum temperature of the warmest month, 1.5%; QSCAT standard deviation, h sensor, 0.7%; QSCAT arithmetic mean, h sensor, 0.5%; and Leaf Area Index 3, 0.2%.
The potential distribution of O. chimborazo, as predicted by the ENM, covers most areas between 3600 and 4600 m of elevation within the geographic extent of the model (Figure (C)). The potential distribution of the hummingbird clearly extends beyond arid volcanic slopes. The distribution is discontinuous because of the presence of deep valleys that break topographical continuity above 3600 m (Figure (C)). This model was strongly supported by the Pearson’s small sample test (D = 10.760, p < 0.001). The ENM also predicted suitable habitat between Quilotoa and Chimborazo, suggesting that environmental conditions in that area are not different from conditions in the rest of the range of the species. In fact, within this geographic region of suitable conditions, are the three intermediate localities between Quilotoa and Chimborazo where we recorded Ecuadorian hillstars were located (Figure (B)).
Specimen attributes
In total, we collected 51 samples of Oreotrochilus chimborazo and two of O. estella stolzmanni (Table ). Of the 51 specimens of O. chimborazo, 15 belonged to subspecies O. c. chimborazo, 34 to O. c. jamesonii, one was an unidentified female from Quilotoa, and another an unidentified male juvenile from Simiatug. All males observed and collected at each locality belonged to only one of the subspecies; females were therefore assumed to belong to the subspecies to which the males from the same locality belonged.
Sequence attributes
With the exception of the juvenile male from Simiatug, DNA sequences were retrieved for all other specimens. In those birds, sequencing was generally successful, with the exception of the first nucleotide of ND2 in 42 specimens and the entire MUSK sequence in 4 cases. Variable/parsimony-informative sites within fragments were distributed as follows: 4/5 for ND2; 4/3 for ND4; and 0/0 for MUSK (data for two variable sites in MUSK were missing for some sequences and thus those sites were excluded from the DNAsp analysis). No indels were found. With inclusion of outgroup sequences, these parameters changed as follows: 113/26 for ND2; 97/21 for ND4; and 2/1 for MUSK (no MUSK sequences were retrieved for O. estella estella, O. melanogaster, or R. microrhynchum). Haplotypic and nucleotide diversity were low across the species (ND2 + ND4, h/π ± SD: h = 0.660 ± 0.067, π = 0.0064 ± 0.0034). All sequences are available from Genbank (accession numbers available in Table ).
Phylogenetic trees and hypothesis testing
MUSK sequences were uninformative regarding tree topology; the BI and ML trees resulting from using this gene were completely unresolved within O. chimborazo (data not shown). The mtDNA and total combined data analyses produced parallel topologies. For the rest of the paper, then, we will refer only to the trees inferred using mtDNA.
Topologies of the majority-rule consensus ML and BI trees were similar. The BI tree, however, resolved additional clades, albeit with low support. In both majority-rule consensus trees O. chimborazo was monophyletic (mtDNA data-set trees: Pp = 1.00; bootstrap ML = 95%). The clade most closely related to O. chimborazo comprised O. melanogaster and the three individuals of subspecies O. estella stolzmanni that were collected at Lagunillas (southern Ecuador) (Figure (A)). The other individual of O. estella, the one for which DNA sequences were retrieved from GenBank, was sister to all other individuals of Oreotrochilus (Figure (A)), which mirrors recent claims that O. estella is not a monophyletic group.[Citation31] The clade of O. chimborazo was an almost completely unresolved polytomy. It was not possible to resolve subspecies (Figure (A)); excluding females (data not shown) did not improve the resolution of the trees. No clade in the Bayesian tree showed geographical structure. In the Maximum Likelihood tree, the birds from El Cajas were grouped in their own clade, albeit with no statistical support (bootstrap value below 70; data not shown). Finally, the test of hypothesis in the Bayesian framework revealed that no tree from the posterior probability distribution showed O. c. chimborazo or O. c. jamesonii as monophyletic.
Figure 2. Bayesian analysis tree (50% majority-rule consensus) of the mitochondrial data-set (ND2 and ND4; 1730 bp). (A) Phylogenetic relationships of Oreotrochilus chimborazo and closely related taxa. The clade inside the grey box, (O. melanogaster + O. estella (Lagunillas)) + O. chimborazo, is expanded in (B) to show all sampled individuals within O. chimborazo. Values of clade support are shown close to the clade root: Posterior Probabilities (Bayesian Infenrence)/bootstrap values (maximum likelihood.
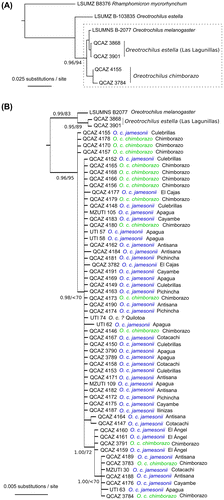
Haplotype and genetic differentiation
Among the 50 individuals analyzed, 10 mtDNA haplotypes were found. The haplotype network was almost star-like, with the same haplotype being present in most individuals of both O. c. jamesonii and O. c. chimborazo (Figure (A)). Most haplotypes were distanced from their closest haplotype by just one evolutionary step. Only two haplotypes showed some degree of geographic aggregation, one at El Cajas Plateau and the other at El Ángel. Individuals from these regions had private alleles (Figure (B)), removed from the common haplotype by one and two steps, respectively (Figure (A)). However, the low sample size from El Ángel or El Cajas precludes estimating the prevalence of these haplotypes within their respective populations.
Figure 3. Data from Haplotype network based on the combined mitochondrial regions ND2 and ND4. (A) Transition network between haplotypes according to maximum parsimony. Haplotypes are named with a unique letter code. Following the haplotype name, the number of individuals bearing the haplotype is given. Box sizes are proportional to number of individuals bearing the haplotypes; (B) Frequency pies for the haplotypes sampled at each locality. The area of the pie is proportional to the sample size at each locality. A map of surface area above 3600 m in elevation is provided for showing the distribution of haplotypes within the Ecuadorian Andes.
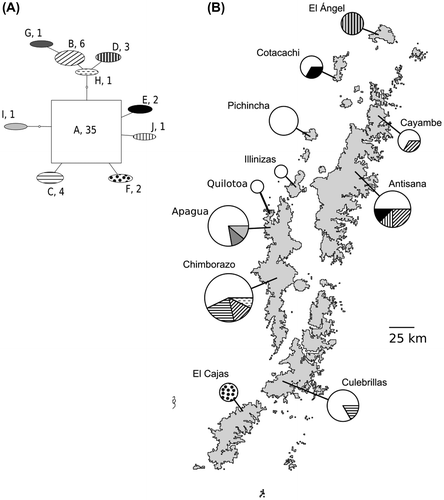
Using AMOVA we did not detect genetic differences between subspecies (FCT=-0.177, p = 0.70 ± 1.54E-2). We did detect genetic differences among paramo islands within subspecies (FSC=0.255, p = 0.004 ± 1.85E-3) and among paramo islands within the whole species (FST=0.123, p = 0.003 ± 1.64E-3). Because O. c. chimborazo is found in only one island, Quilotoa-Chimborazo, differences among islands within subspecies are driven by variation among populations of O. c. jamesonii.
Discussion
The subspecific diversity of the Ecuadorian hillstar has often been cited as a remarkable example of how restricted ecological requirements can combine with habitat insularity to generate evolutionary divergence.[Citation8–11,14] According to the literature, the species comprises three geographically isolated subspecies, two of them restricted to the volcanoes Chimborazo and Quilotoa.[Citation8,9,12] Careful review of the literature, however, suggests that populations of Chimborazo and Quilotoa may not be isolated from each other. Elsewhere in Ecuador, O. chimborazo has been recorded at localities that are not particularly close to volcanoes,[Citation11,14,17,18] in grassland paramo, which is the habitat that occurs between Quilotoa and Chimborazo.[Citation19] Recently, photographs taken in Quilotoa presented preliminary evidence that populations of both subspecies occur in the area.[Citation20] In this study, we confirmed that Ecuadorian hillstars occur between Quilotoa and Chimborazo. We found subspecies O. c. jamesonii 15-km south of Quilotoa and, further south, we found individuals of O. c. jamesonii and O. c. chimborazo living at most 15 km apart from each other (Figure (B)). Our ENM (Figure (C)) predicted occurrence of O. chimborazo in this region, despite not using the localities between Quilotoa and Chimborazo to build the model. Taken together, our results strongly suggest that the populations of both subspecies are in contact.
Our results also suggest that subspecies O. c. soderstromi is probably an intergrade between the other two subspecies. A visual inspection of the holotype (NRM 569802, Naturhistoriskariksmuseet, Royal Museum of Natural History, Sweden) reveals characteristics that are intermediate between O. c. chimborazo and O. c. jamesonii; namely, a purple gorget interspersed with scattered green feathers. Even in the original description of the holotype,[Citation12] the intergrade hypothesis was already stated, but it was dismissed on the basis of rather weak claims. It was mentioned that hummingbirds of O. c. soderstromi, have ‘a known local name’ and that they are ecologically different from other Ecuadorian hillstars because O. c. soderstromi supposedly occured at lower elevations.[Citation12] Ecological differences are unlikely because O. c. jamesonii has been recorded within the same elevational range at which O. c. soderstromi was recorded in Quilotoa.[Citation8] However, to be fair to the authors of the account, those records of O. c. jamesonii were obtained several years after the description of O. c. soedrestromi was published. The validity of O. c. soerstromi has also been defended on the assumption that hillstars in Quilotoa are geographically isolated from other hillstars.[Citation8,9] However, as we have discussed, considerable evidence supports the contrary. Arguments in favor of O. c. soderstromi as a valid subspecies are therefore unconvincing; consequently, we suggest that the demotion of this taxon is in place.
According to our ENM, the potential distribution of O. chimborazo is widespread along the Ecuadorian paramo, with no higher probability of occurrence in drier regions. In the literature, some places that are predicted by our ENM have been stated to be too humid for O. chimborazo. Such places include, for example, Llanganates National Park [Citation8] and Cayambe paramo.[Citation11] However, there is a relatively recently published record of the hillstar in Llanganates,[Citation18] and this occurrence is predicted by our ENM. Though this locality was used to build the ENM, removal of the locality during Pearson validation tests did not affect the outcome of the ENM with regards to predicting occurrence in this area (data available upon request). In Cayambe, we confirmed the occurrence of O. chimborazo and collected voucher specimens; also, this locality is in a region of high likelihood of occurrence according to our ENM (Figure (C)).
Although O. chimborazo may occur throughout the Ecuadorian paramos, we do not reject a possible preference for arid paramos. During our fieldwork, O. chimborazo seemed to be more abundant at sites with barren, sandy soil, where Chuquiraga was among the few yet abundant plants growing there. Cunungyacu, our locality closest to Mt. Chimborazo, was one of those sites. Hillstars were so abundant at that locality that we managed to capture 15 individuals in just two days of field work. In other sites, it would take us about three days to catch up to five individuals. Strong preference for arid environments may effectively isolate populations, which may have resulted in at least one morphological character (i.e. gorget color) being different between populations of the hillstar. However, studies aimed to characterize local abundances, specific habitat preferences, and patterns of individual movement between sites are required to test this hypothesis.
Arid conditions in the paramo may be promoted by anthropogenic pressure. Burning is a common and widespread regime in the Ecuadorian paramos that leads to desertification and erosion.[Citation63] In the past, grassland paramos, generally associated with drier conditions than those of other paramos, may have not been as extensive as they are now. Indeed, ecological studies suggest that forests in the Ecuadorian Andes may have been more extensive than they are today, before burning and grazing practices begun,[Citation64,65] and may have reached elevations close to 4100 m.[Citation65] If this pattern holds true, paramo degradation may be masking the original environmental factors that originally limited the distribution of the hummingbird.
Every site where O. chimborazo was found showed some evidence of degradation, whether in the way of regular fires or cattle grazing (personal observations). By benefiting paramos over original forest, habitat disturbance may have extended the distribution of Chuquiraga and consequently that of the Ecuadorian hillstar.[Citation11,15] A restricted geographic range in the recent past and expansion of such range is consistent with the star-like haplotype network (Figure ) and low genetic diversity within O. chimborazo. Interestingly, the area where the presumed contact zone is located has a long record of human activity.[Citation66] Before human intervention, both subspecies may have been isolated to islands of arid paramo, separated by a barrier of undisturbed humid habitat where neither Chuquiraga nor Oreotrochilus may have occurred. In our visits to the field we found, amid the grassland paramo, patches of Polylepis forest (in Mulá Corral creek) restricted to hilltops along very steep slopes where the degree of human intervention is low, suggesting that the area was in a good climatic zone for forest. On the other hand, slash and burn agriculture has also been hypothesized to threaten Chuquiraga and, therefore, O. chimborazo.[Citation14] Accordingly, in some mountains we did not find large patches of Chuquiraga below 4100 m, presumably because of intense degradation of the paramo.
Moderate anthropogenic pressure in the recent past is not the sole possibility that may explain secondary contact between subspecies O. c. chimborazo and O. c. jamesonii. Natural fluctuations in climate, possibly during the early Holocene,[Citation65] may have restricted the range of suitable habitats for O. chimborazo in the recent past. For example, after glaciers retreated from the Last Glacial Maximum, global temperature increased to levels slightly higher than those of today, which has been suggested to have favored the growth of forests and displaced paramos to higher elevations.[Citation65,67,68] Paramos, therefore, may have been more insular, presenting more opportunities for divergence promoted by geographic isolation.
Regardless of the factors driving morphological divergence, we hypothesized that O. c. chimborazo and O. c. jamesonii constitute distinct genetic lineages. Our analyses, however, did not support our hypothesis: (1) we did not recover the two subspecies in the phylogeny, (2) the probability of monophyly of either subspecies was close to zero (according to the Bayesian hypothesis test), and (3) we failed to reject the null hypothesis that O. c. chimborazo and O. c. jamesonii are genetically similar (see AMOVA). Our results, however, cannot be used to conclude that genetic divergence is inexistent among subspecies of O. chimborazo. Their distinct morphology and geographic ranges suggest some degree of genetic divergence that arose at some point in their evolutionary history. Such divergence, however, may have been too recent to be reflected in mtDNA. In fact, if current geographic isolation has played any role in the separation between subspecies, it may not have started to act until very recently, because before 13,000 years ago, during the last glaciation, the paramo probably occupied a lower, more continuous zone.[Citation65,69,70] In addition, slow molecular evolution, which has been suggested to exist in high-elevation hummingbirds,[Citation71] may have hindered accumulation of informative mtDNA substitutions in this relatively short time.
Is not uncommon for mtDNA to be similar between populations classified as different subspecies.[Citation72,73] Fast accumulation of plumage characters could be promoted, for example, by sexual selection [Citation22] when novel characters increase the attractiveness of males. The diversity of plumages among males of the different species of Oreotrochilus [Citation7] is in sharp contrast with the similar plumages among females, suggesting a role for sexual selection in shaping the evolution of male plumage in this genus. Distinct plumage characters between the subspecies of O. chimborazo may hint at incipient divergence resulting from geographic isolation in the recent past; the populations may have been isolated for enough time for one population to start diverging on plumage characters under sexual selection, but not enough time to leave traces of the divergence in mtDNA. In cases like these, the use of faster evolving markers, such as those based on microsatellites [Citation74] or SNPs, is recommended.
Finally, because the range of O. c. jamesonii is highly fragmented, we hypothesized that the population genetics of this subspecies would show geographic structure. Using AMOVA, we found significant differences between hummingbirds living in different paramo islands. However, results from phylogenetic trees and haplotype networks were not supportive for such differences (Figure (A)); only two populations seemed to have genetic structure in the haplotype network, those from El Cajas plateau and from El Ángel (Figure (A) and (B)), but this separation is not conclusive. Still, these paramos are notorious for the presence of several endemic species of plants,[Citation19] insects, [Citation74] and distinct forms of other Andean birds.[Citation30] Such endemism might be related to the age of the valleys that separate these paramos from other paramos, which are ~2 million years older than most other valleys in the Ecuadorian Andes.[Citation75] Thus, we think that it would be worthwhile using larger sample sizes to test if hillstars from El Cajas and El Ángel belong to diverging lineages.
Disclosure statement
No potential conflict of interest was reported by the authors.
Acknowledgments
Field work was possible thanks to the help of several biology students from PUCE and staff from QCAZ, including D. Bahamonde, H. F. Cadena, J. Castillo, M. Ninazunta, A. Charpentier, C. Rodríguez, M. Santacruz, and J. Sindram. Ana Charpentier and M. J. Erazo provided valuable information on localites where hillstars occur; M. E. Ordóñez and C. Barnes provided advice on molecular biology techniques; C. C. Witt recommended the molecular primers for this study; D. Arzuza assisted with the preparation of specimens and data management of collections; P. Menéndez provided access to data from remote sensing systems; U. Johansson kindly provided pictures from the O. c. soderstromi specimen housed at NRM. Juan F. Freile, N. Krabbe, R. S. Ridgely and R. Ahlman shared personal observations about the hillstars; J. F. Freile and J. M. Guayasamin provided feedback at multiple stages during this project, and J. M. Guayasamin, T. de Vries, and C. Barnes provided valuable comments on the manuscript. Research permits were issued by Ministerio del Ambiente in Ecuador (008–09 ICFAU-DNB/MA; 001–10 IC-FAU-DNB/MA; MAE-DNB-CM-2015–0017). Associate Editor: Townsend Peterson.
Funding
This work was supported by Secretaría Nacional de Ciencia y Tecnología of Ecuador SENACYT [grant number PI-C08–0000470] and Universidad Tecnológica Indoamérica (Convocatoria a proyectos 2011).
References
- Fjeldså J, Bowie RCK, Rahbek C. The role of mountain ranges in the diversification of birds. Annu. Rev. Ecol. Evol. Syst. 2012;43:249–265.10.1146/annurev-ecolsys-102710-145113
- Cadena CD, Klicka J, Ricklefs RE. Evolutionary differentiation in the Neotropical montane region: molecular phylogenetics and phylogeography of Buarremon brush-finches (Aves, Emberizidae). Mol. Phylogenet. Evol. 2007;44:993–1016.10.1016/j.ympev.2006.12.012
- Bonaccorso E. Historical biogeography and speciation in the Neotropical highlands: molecular phylogenetics of the jay genus Cyanolyca. Mol. Phylogenet. Evol. 2009;50:618–632.10.1016/j.ympev.2008.12.012
- Winger BM, Bates JM. The tempo of trait divergence in geographic isolation: avian speciation across the Marañon valley of Peru: tempo of avian speciation across the Marañon. Evolution. 2015;69:772–787.10.1111/evo.2015.69.issue-3
- Graves GR. Linearity of geographic range and its possible effect on the population structure of Andean birds. The Auk. 1988;105:47–52.
- Weir JT. Implications of genetic differentiation in Neotropical montane forest birds. Ann. Mo. Bot. Gard. 2009;96:410–433.10.3417/2008011
- Schuchmann K, Del Hoyo J. Family Trochilidae (Hummingbirds). Handb. Birds World. 1999;5:468–680.
- Ridgely R, Greenfield P. The birds of ecuador: status, distribution, and taxonomy, Vol. 1. Ithaca (NY): Cornell University Press; 2001.
- Fjeldså J, Krabbe N. Birds of the High Andes. Svendborg: Zoological Museum, University of Copenhagen and Apollo Books; 1990.
- Wallace AR. Island life : or, the phenomena and causes of insular faunas and floras, including a revision and attempted solution of the problem of geological climates [Internet]. 3rd and rev. ed. London : Macmillan; 1911. Available from: http://www.biodiversitylibrary.org/bibliography/52127.
- Ortiz-Crespo FI, Bleiweiss R. The northern limit of the hummingbird genus Oreotrochilus in South America. The Auk. 1982;2:376–378.
- Lönnberg E, Rendahl H. A contribution to the ornithology of Ecuador. Ark. För Zool. Stockh. 1922;14:1–87.
- Sauer W. Geología del Ecuador [Geology of Ecuador]. Quito: Ministerio de Educación; 1965.
- Woods S, Ortiz-Crespo F, Ramsay PM. Presence of giant hummingbird Patagona gigas and Ecuadorian Hillstar Oreotrochilus chimborazo jamesoni at the Ecuador-Colombia border. Cotinga. 1998;10:37–40.
- Smith GTC. A high altitude hummingbird on the Volcano Cotopaxi. Ibis. 1969;111:17–22.
- Solano-Ugalde A. High in the Andes: colonial nesting of Ecuadorian Hillstar (Oreotrochilus chimborazo: trochilidae) under a bridge. Ornitol. Colomb. 2008;6:86–88.
- Vuilleumier F. On the occurrence and identity of Oreotrochilus Chimborazo at the Guamani Pass. Ecuador. Ibis. 1976;118:425–425.
- Benítez V, Sánchez D, Larrea M. Evaluación ecológica rápida de la avifauna en el Parque Nacional Llanganates: un reporte de las evaluaciones ecológicas y socioeconómicas rápidas [Rapid environmental assessment of the avifauna of Llanganates National Park]. Quito: EcoCiencia Ministerio del Ambiente, Herbario Nacional de Ecuador, Museo de Ecuatoriano de Ciencias Naturales, Instituto Internacional de Reconstrucción Rural; 2000. p. 67–107.
- Beltrán K, Salgado S, Cuesta F, et al. Distribución espacial, sistemas ecológicos y caracterización florística de los páramos en el Ecuador [Spatial distribution, ecological systems and floristic characterization of the paramos of Ecuador]. Quito: EcoCiencia; 2009
- Ahlman R. Ecuadorian Hillstar by Roger Ahlman [Internet]. PBase- Roger Ahlman- Ecuadorian Hillstar; 2011 [cited 2015 Jun 7]. Available from: http://www.pbase.com/ahlman/image/134844820
- Gavrilets S. Fitness landscapes and the origin of species (MPB-41). Princeton: University Press Princeton; 2004.
- Price T, 1953-. Speciation in birds. 2008 [cited 2014 Dec 23]. Available from: http://agris.fao.org/agris-search/search.do?recordID=US201300125044.
- Chesser RT. Evolution in the high Andes: the phylogenetics of Muscisaxicola ground-tyrants. Mol. Phylogenet. Evol. 2000;15:369–380.10.1006/mpev.1999.0774
- Chaves JA, Weir JT, Smith TB. Diversification in Adelomyia hummingbirds follows Andean uplift. Mol. Ecol. 2011;20:4564–4576.10.1111/j.1365-294X.2011.05304.x
- Sanín C, Cadena CD, Maley JM, et al. Paraphyly of Cinclodes fuscus (Aves: Passeriformes: Furnariidae): implications for taxonomy and biogeography. Mol. Phylogenet. Evol. 2009;53:547–555.10.1016/j.ympev.2009.06.022
- Rheindt FE, Cuervo AM, Brumfield RT. Rampant polyphyly indicates cryptic diversity in a clade of Neotropical flycatchers (Aves: Tyrannidae). Biol. J. Linn. Soc. 2013;108:889–900.10.1111/bij.2013.108.issue-4
- Mata H, Fontana CS, Maurício GN, et al. Molecular phylogeny and biogeography of the eastern Tapaculos (Aves: Rhinocryptidae: Scytalopus, Eleoscytalopus): cryptic diversification in Brazilian Atlantic forest. Mol. Phylogenet. Evol. 2009;53:450–462.10.1016/j.ympev.2009.07.017
- Fernandes AM, Gonzalez J, Wink M, et al. Multilocus phylogeography of the wedge-billed woodcreeper Glyphorynchus spirurus (Aves, Furnariidae) in lowland Amazonia: widespread cryptic diversity and paraphyly reveal a complex diversification pattern. Mol. Phylogenet. Evol. 2013;66:270–282.10.1016/j.ympev.2012.09.033
- Robbins MB, Stiles FG. A new species of Pygmy-Owl (Strigidae: Glaucidium) from the Pacific Slope of the Northern Andes. The Auk. 1999;116:305–315.10.2307/4089365
- Krabbe N. Arid valleys as dispersal barriers to high-Andean forest birds in Ecuador. Cotinga. 2008;29:28–30.
- McGuire JA, Witt CC, Remsen JV, et al. Molecular phylogenetics and the diversification of hummingbirds. Curr. Biol. 2014;24:910–916.10.1016/j.cub.2014.03.016
- McGuire JA, Witt CC, Altshuler DL, et al. Phylogenetic systematics and biogeography of hummingbirds: bayesian and maximum likelihood analyses of partitioned data and selection of an appropriate partitioning strategy. Syst. Biol. 2007;56:837–856.10.1080/10635150701656360
- Elith J, Phillips SJ, Hastie T, et al. A statistical explanation of MaxEnt for ecologists. Divers. Distrib. 2011;17:43–57.10.1111/ddi.2010.17.issue-1
- Phillips SJ, Dudík M. Modeling of species distributions with Maxent: new extensions and a comprehensive evaluation. Ecography. 2008;31:161–175.10.1111/j.0906-7590.2008.5203.x
- eBird. eBird: an online database of bird distribution and abundance [Internet]. eBird; 2015. Available from: http://www.ebird.org
- Hijmans RJ, Cameron SE, Parra JL, et al. Very high resolution interpolated climate surfaces for global land areas. Int. J. Climatol. 2005;25:1965–1978.10.1002/(ISSN)1097-0088
- Barve N, Barve V, Jiménez-Valverde A, et al. The crucial role of the accessible area in ecological niche modeling and species distribution modeling. Ecol. Model. 2011;222:1810–1819.10.1016/j.ecolmodel.2011.02.011
- Lillesand TM, Kiefer RW, Chipman JW. Remote sensing and image interpretation. New York (NY): Wiley; 2004.
- Myneni R, Hoffman S, Knyazikhin Y, et al. Global products of vegetation leaf area and fraction absorbed PAR from year one of MODIS data. Remote Sens. Environ. 2002;83:214–231.10.1016/S0034-4257(02)00074-3
- Buermann W, Saatchi S, Smith TB, et al. Predicting species distributions across the Amazonian and Andean regions using remote sensing data. J. Biogeogr. 2008;35:1160–1176.10.1111/jbi.2008.35.issue-7
- Pearson RG, Raxworthy CJ, Nakamura M. Predicting species distributions from small numbers of occurrence records: a test case using cryptic geckos in Madagascar. J. Biogeogr. 2007;34:102–117.
- Peterson AT, Papeş M, Soberón J. Rethinking receiver operating characteristic analysis applications in ecological niche modeling. Ecol. Model. 2008;213:63–72.10.1016/j.ecolmodel.2007.11.008
- Chomczynski P. A reagent for the single-step simultaneous isolation of RNA, DNA and proteins from cell and tissue samples. BioTechniques. 1993;15:536–537.
- Sorenson MD, Ast JC, Dimcheff DE, et al. Primers for a PCR-based approach to mitochondrial genome sequencing in birds and other vertebrates. Mol. Phylogenet. Evol. 1999;12:105–114.10.1006/mpev.1998.0602
- Kimball RT, Braun EL, Barker FK, et al. A well-tested set of primers to amplify regions spread across the avian genome. Mol. Phylogenet. Evol. 2009;50:654–660.10.1016/j.ympev.2008.11.018
- Arèvalo E, Davis SK, Sites JW. Mitochondrial DNA sequence divergence and phylogenetic relationships among eight chromosome races of the Sceloporus grammicus complex (phrynosomatidae) in central Mexico. Syst. Biol. 1994;43:387–418.10.1093/sysbio/43.3.387
- Thompson JD, Gibson TJ, Plewniak F, et al. The CLUSTAL_X windows interface: flexible strategies for multiple sequence alignment aided by quality analysis tools. Nucleic Acids Res. 1997;25:4876–4882.10.1093/nar/25.24.4876
- Librado P, Rozas J. DnaSP v5: a software for comprehensive analysis of DNA polymorphism data. Bioinformatics. 2009;25:1451–1452.10.1093/bioinformatics/btp187
- Clement M, Posada D, Crandall KA. TCS: a computer program to estimate gene genealogies. Mol. Ecol. 2000;9:1657–1659.10.1046/j.1365-294x.2000.01020.x
- Altshuler DL, Dudley R, McGuire JA. Resolution of a paradox: hummingbird flight at high elevation does not come without a cost. Proc. Nat. Acad. Sci. U.S.A. 2004;101:17731–17736.10.1073/pnas.0405260101
- Kirchman JJ, Witt CC, McGuire JA, et al. DNA from a 100-year-old holotype confirms the validity of a potentially extinct hummingbird species. Biol. Lett. 2009;rsbl20090545. Available from: http://rsbl.royalsocietypublishing.org/content/roybiolett/early/2009/09/17/rsbl.2009.0545.full.pdf
- Zwickl DJ. Genetic algorithm approaches for the phylogenetic analysis of large biological sequence datasets under the maximum likelihood criterion [Internet] [Ph. D. dissertation]. The University of Texas at Austin; 2006. Available from: http://garli.googlecode.com
- Lewis PO, Holder MT, Swofford DL. Phycas: software for bayesian phylogenetic analysis. Syst. Biol. 2015;64:525–531.10.1093/sysbio/syu132
- Lanfear R, Calcott B, Ho SYW, et al. Partitionfinder: combined selection of partitioning schemes and substitution models for phylogenetic analyses. Mol. Biol. Evol. 2012;29:1695–1701.10.1093/molbev/mss020
- Felsenstein J. Evolutionary trees from DNA sequences: a maximum likelihood approach. J. Mol. Evol. 1981;17:368–376.10.1007/BF01734359
- Kimura M. Estimation of evolutionary distances between homologous nucleotide sequences. Proc. Nat. Acad. Sci. U.S.A. 1981;78:454–458.10.1073/pnas.78.1.454
- Hasegawa M, Kishino H, Yano T. Dating of the human-ape splitting by a molecular clock of mitochondrial DNA. J. Mol. Evol. 1985;22:160–174.10.1007/BF02101694
- Maddison WP, Maddison DR. Mesquite: a modular system for evolutionary analysis [Internet]. 2015. Available from: http://mesquiteproject.org
- R Core Team. R: a language and environment for statistical computing [Internet]. Vienna: R Foundation for Statistical Computing; 2014. Available from: http://www.R-project.org/
- Excoffier L, Smouse PE, Quattro JM. Analysis of molecular variance inferred from metric distances among DNA haplotypes: application to human mitochondrial DNA restriction data. Genetics. 1992;131:479–491.
- Excoffier L, Laval G, Schneider S. Arlequin (version 3.0): an integrated software package for population genetics data analysis. Evol. Bioinforma. Online. 2007;1:47–50.
- Huelsenbeck JP, Rannala B. Frequentist properties of Bayesian posterior probabilities of phylogenetic trees under simple and complex substitution models. Syst. Biol. 2004;53:904–913.
- Sklenář P, Lægaard S. Rain-shadow in the high Andes of Ecuador evidenced by páramo vegetation. Arct. Antarct. Alp. Res. 2003;35:8–17.10.1657/1523-0430(2003)035[0008:RSITHA]2.0.CO;2
- Laegaard S. Influence of fire in the grass páramo vegetation of Ecuador. In: Balslev H and Luteyn JL, editors. Páramo: An Andean Ecosystem Under Human Influence. London: Academic Press; 1992. p. 151–170.
- Lauer W, Rafiqpoor MD, Theisen I. Physiographie, Vegetation und Syntaxonomie der Flora des Páramo de Papallacta (Ostkordillere Ecuador) [Physiography, vegetation and syntaxonomy of the flora of Paramo de Papallacta (Eastern Cordillera Ecuador)]. Stuttgart: Franz Steiner Verlag Wiesbaden GMBH; 2001.
- Podwojewski P, Poulenard J, Zambrana T, et al. Overgrazing effects on vegetation cover and properties of volcanic ash soil in the páramo of Llangahua and La Esperanza (Tungurahua, Ecuador). Soil Use Manag. 2002;18:45–55.10.1079/SUM2002100
- Lozano P, Cleef AM, Bussmann RW. Phytogeography of the vascular páramo flora of Podocarpus National Park, south Ecuador. Arnaldoa. 2009;16:69–85.
- Van der Hammen T, Cleef A. Development of the high Andean páramo flora and vegetation. In: Vuilleumier F, Monasterio FM, editors. High altitude tropical biogeography. Oxford: Oxford University Press; 1986. p. 153–201.
- Van der Hammen T. The pleistocene changes of vegetation and climate in tropical South America. J. Biogeogr. 1974;1:3–26.10.2307/3038066
- Hooghiemstra H, Wijninga VM, Cleef AM. The paleobotanical record of Colombia: implications for biogeography and biodiversity. Ann. Missouri Bot. Gard. 2006;93:297–325.10.3417/0026-6493(2006)93[297:TPROCI]2.0.CO;2
- Bleiweiss R. Slow rate of molecular evolution in high-elevation hummingbirds. Proc. Nat. Acad. Sci. 1998;95:612–616.10.1073/pnas.95.2.612
- Zink RM. The role of subspecies in obscuring avian biological diversity and misleading conservation policy. Proc. R. Soc. Lond. B Biol. Sci. 2004;271:561–564.10.1098/rspb.2003.2617
- Phillimore AB, Owens IP. Are subspecies useful in evolutionary and conservation biology? Proc. R. Soc. B Biol. Sci. 2006;273:1049–1053.10.1098/rspb.2005.3425
- Petren K, Grant BR, Grant PR. A phylogeny of Darwin’s finches based on microsatellite DNA length variation. Proc. R. Soc. Lond. B Biol. Sci. 1999;266:321–329.10.1098/rspb.1999.0641
- Anderson RS. Los Coleópteros Carabidae del páramo en los Andes del Ecuador: sistemática, ecología y biogeografía [The coleopteran Carabidae of paramo in the Andes of Ecuador: systematics, ecology, and biogeography]. Coleopt. Bull. 2007;61:176–176.10.1649/0010-065X(2007)61[176:LCCDPE]2.0.CO;2