Abstract
Insect community structures in tropical regions appear to be related to the hydrological regime and energy paths trough autochthonous and allochthones sources. Macrophyte and metaphyton permanent coverage throughout the year generate conditions and resources, such as food and refuge, allowing the establishment of a large number of aquatic insect species. The hydrological regime is based on rain seasonality and has a direct influence on insect lifecycles. We evaluated insect community structures in five systems with differences in macrophyte/metaphyton coverage and hydrological regimes (two temporary wetlands and three permanent cenotes) in the Sian Ka’an Biosphere Reserve in southeast Mexico. The insect diversity values highly correlated with metaphyton abundance but not with macrophyte coverage. We also found that cenote hydrological stability could produce higher insect diversity in comparison with temporary wetlands.
La comunidad de insectos en regiones tropicales está relacionada con el régimen hídrico y las rutas de energía tanto alóctonas como autóctonas. A lo largo del año, la cobertura permanente de las macrófitas y el metafiton generan condiciones y recursos propicios para la sobrevivencia de insectos, lo que ayuda a establecer un mayor número de especies. Por su parte, el régimen hídrico basado lluvias y secas, tiene un efecto directo en el ciclo de vida de los insectos. En este trabajo evaluamos la estructura de la comunidad de insectos en cinco sistemas con diferencias en la cobertura de los productores primarias y en el régimen hídrico (dos humedales temporales y tres cenotes con agua todo el año) en la Reserva de la Biósfera de Sian Ka´an al sudeste de México. Encontramos que la diversidad de insectos está relacionada con la abundancia de metafiton, pero no lo está con la cobertura de macrófitas. También encontramos que la estabilidad hídrica de los cenotes podría producir una mayor diversidad de insectos en comparación con los humedales más temporales.
Introduction
Aquatic insect diversity is positively related to environmental heterogeneity.[Citation1,2] In aquatic systems, primary producers mostly generate spatial heterogeneity by modifying the local environment and increasing the number of microhabitats [Citation3] for different animal species.[Citation4–6] Nevertheless, each primary producer has different morphologies and physiologies that generate dissimilar local conditions, such as microclimate, stream flow, food source and shelter against predators,[Citation7–10] which is related to the number of available habitats.[Citation11]
In aquatic systems, primary producers can be grouped into the following four categories: (1) phytoplankton, (2) periphyton, (3) metaphyton and (4) macrophytes. Because most phytoplankton do not generate structures suitable for macroinvertebrates, this group mostly affects habitats as a food source.[Citation12–14] In contrast, periphyton, metaphyton and macrophytes not only provide a food source but also provide shelter and modify the microclimate for macroinvertebrates and fish.[Citation7,8,10,15]
In tropical wetlands, temperature seasonal variation is lower than in temperate systems. This small temperature variability has been related to larger macrophyte biodiversity in the neotropics than in the rest of the world.[Citation16] Nevertheless, a marked seasonal variation in rainfall exists in most tropical wetlands that provokes floods as well as affects populations and macrophyte community structures.[Citation17,18]
The morphology of primary producers may also influence the aquatic fauna composition.[Citation19–21] Macrophytes can be classified according their morphology and growth form as emergent, free-floating or submerged.[Citation22,23] Each morphological characteristic allows the establishment of different aquatic insect communities.[Citation21,24]
Metaphyton has been defined as a floating mat composed mainly by macroscopic filamentous cyanoprokaryotes, diatoms, detritus and other components.[Citation25] These free-floating structures are formed by several types of algae, protozoans, bacteria, fungi and detritus that serve as food sources for aquatic insects.[Citation6,26–30] The influence of metaphyton in aquatic systems in tropical systems has received relatively little attention, although this algae community greatly influences the aquatic ecosystem ecology dynamics, such as nutrient cycles,[Citation31] primary production, fauna diversity,[Citation15,31–35] and even the number of larvae of the emergent insects.[Citation33]
Usually, complex structures generated by these primary producers contain higher richness and diversity values of aquatic insects within a lake or river in temperate zones. For example, in the St. Lawrence River, Canada, metaphyton show larger macroinvertebrate biomasses than less complex substrates, such as submerged plants, which in turn show larger insect biomasses than emergent plants with simpler structures.[Citation25] Nevertheless, this relationship depends on local conditions. For example, in eutrophic ponds in Fleetwood, UK, macroinvertebrate diversity values were higher in emergent rather than submerged or free-floating macrophytes.[Citation21] However, these studies were focused on temperate systems with high anthropogenic disturbances that may affect these relationships.
In tropical regions, hydrological lake dynamics may influence the relationship between insects and primary producers. Changes in the hydraulic regime can affect the insect life strategies, which alters their survival capabilities and, consequently, their diversity.[Citation26]
In this study, we aimed to evaluate these relationships within a pristine tropical system, in the Sian Ka’an Biosphere Reserve (SKBR), the largest reserve in Mexico, to better understand the relationships between insects and different primary producers related to hydrological changes. Our main purpose was to determine the relationship between the diversity of aquatic insect community with metaphyton and emergent macrophytes in a pristine setting, using both permanent and temporary tropical water bodies.
Methods
The SKBR is located in the Quintana Roo state in southeast México between 20° 07′ 28′′ and 18° 07′ 28′′ latitude and 88° 12′ 12′′ and 87° 24′ 36′′ longitude (Figure ). The reserve is 5517.5 km2 in area and an average of nine meters above sea level.[Citation34] The climate is tropical wet and dry seasons (AW); it is warm and sub-humid with a summer rainy season and an annual average temperature of 26 °C with between 1100 and 1200 mm of annual precipitation. The rainy season lasts from May to March, and it is divided in two periods. The first period, a warmer rainy season due to hurricanes, lasts from May to October with 70% (800–1000 mm) of the rainfall. The second period, with colder precipitation from the humidity from by the Gulf of Mexico nortes, lasts from November to March with 150–300 mm of rainfall. April to July is the dry season, with approximately 60 mm of rainfall.[Citation34–36]
Figure 1. Geographical distribution of cenotes and wetlands sampled at Sian Ka’an Biosphere Reserve in the southeast of Mexico.
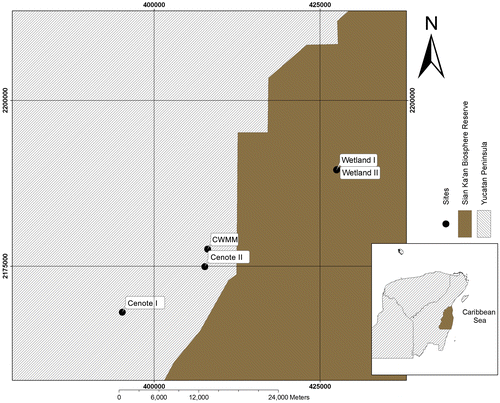
The SKBR is part of one of the biggest karst systems on the earth, with soil composed of sedimentary rocks including limestones and dolomites.[Citation34–37] The characteristics of the soil permit the existence of karst formations from the weathering of calcium carbonate in the zone, generating complex hydrological systems in a small geographic area composed of temporal wetlands and permanent cenotes.[Citation38–40]
Cenotes are formed when calcareous soil collapses over an underground river leaving the underground water supply open to the outside.[Citation37,41] Seasonal changes have a small influence on these water bodies. In contrast, wetlands totally depend on the rainfall, which causes their size to vary by season throughout the year.[Citation41] Wetlands are water-covered surfaces with a depth below two meters.[Citation36] Their large surface and low depth makes these water bodies susceptible to changes in temperature throughout the day and higher evaporation rates; thus, they depend on a constant supply of water from rainfall.[Citation42]
SKBR vegetation is characterized by semi-deciduous and flooded forests, in which the dominant species include Brosimum alicastrum, Manilkara zapota, Thrinax radiata, Bursera simaruba, Caesalpinia gaumeri, Haematoxylum campechianum and Bucida buceras.[Citation34–36] The aquatic vegetation community is dominated by emergent plants such as Typha domingensis, Cladium jamaicense, Phragmites australis and Echinodorus andrieuxii.[Citation43,44] According to metaphyton studies of areas close to the Reserve, the metaphyton is mainly composed of filamentous cyanoprokaryota with a high presence of diatoms.[Citation45]
Two wetlands and three cenotes were sampled within a year in the following three seasons: (1) warm rainy season (June), (2) cold rainy season (November) and (3) dry season (April). One of the cenotes has no littoral vegetation, while aquatic vegetation is present in the other two cenotes. To avoid species–area relationships, wetlands sampled areas were similar to cenotes (500–7000 m2). We choose cenotes and wetlands to evaluate the role of macrophytes and metaphyton on insects in aquatic systems with different water regimes, permanent in cenotes and temporary in wetlands.
In each system, we evaluated physicochemical parameters with a multiparameter probe (Hanna HI9828); wetlands were not measured in dry season because depth was below 5 cm. In addition, the percent coverage by macrophytes and metaphyton was determined. We only evaluated the percentage of emergent macrophytes, as it is the dominant macrophyte community. For both macrophytes and metaphyton, the percent coverage was evaluated with the naked eye within 1 m2 quadrants.[Citation46] We randomly placed 10 quadrants in the wetlands. In contrast, the quadrants were only placed in the littoral zones of the cenotes, as it is the only place macrophytes and metaphyton are established in the system.
The insects were sampled with a standard 30.5 cm D-frame aquatic net (mesh size of 0.25 mm). These nets are frequently used and recommended to sample macroinvertebrates on different kind of substrates in aquatic systems, allowing a homogenized sample.[Citation47] In both cases, (wetlands and cenotes) macroinvertebrates were samples with 12 swings randomly placed on the wetland surface and in the littoral zones of cenotes, sampling nets were swung up from the substrate regardless of whether the substrate was soft or hard.
The collected insects were separated, preserved (in alcohol 80%) and transported to the laboratory. The insects were then counted and identified down to the genus level with taxonomical keys.[Citation48–59]
A non-metric multidimensional scaling (NMDS) was carried out in order to determine correlations among physicochemical variables. Aquatic primary producer coverage was compared among the sample sites with ANOVA, excluding the cenote without any primary producers. The insect community structure was characterized according to its total insect abundance and insect diversity using genus as a base unit and Fisher’s alpha diversity index. We also looked for relationships among macrophytes, metaphyton and insect community attributes with correlation analyses using each sample season as a unit.
Results
Variability in physicochemical parameters was larger in wetlands than in cenotes. (Table ). This last one presented higher values in oxygen concentration and pH and lower values in conductivity than wetlands. According to NMDS (Stress = 0.1; Figure ) temperature and oxygen are the main factors of variance among sites and seasons.
Table 1. Average of temperature, conductivity, dissolved oxygen and pH in the studied aquatic systems during the annual cycle in SKBR, Quintana Roo, Mexico.
Figure 2. Non-metrics multidimensional analysis, NMDS, for environmental variables of SKBR, Quintana Roo, Mexico. C1 = Cenote 1, C2 = Cenote 2, CWMM = Cenote without macrophytes and metaphyton, W1 = Wetland 1, W2 = Wetland 2.
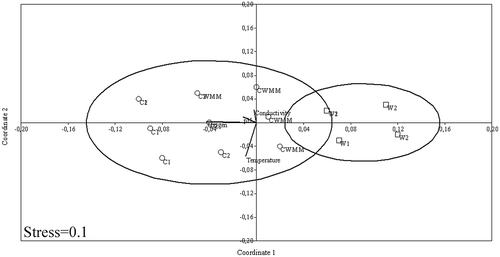
Macrophyte coverage did not change among seasons (Table ). However, there was significantly higher macrophyte coverage in the wetlands than in the cenotes (H = 66.04, p < 0.05). In contrast, metaphyton demonstrated significant coverage differences among the seasons (F = 3.06, p < 0.05) and between the cenotes and the wetlands (F = 4.43, p < 0.05). A negative correlation was found between the macrophyte and metaphyton coverage (Figure ).
Table 2. Averages of the percentages of macrophytes covering periphyton mats in the aquatic systems studied, SKBR, Quintana Roo, Mexico.
Figure 3. Correlation between coverage macrophytes and metaphyton in aquatic systems of SKBR, Quintana Roo, Mexico. Circles are cenotes and triangles are wetlands.
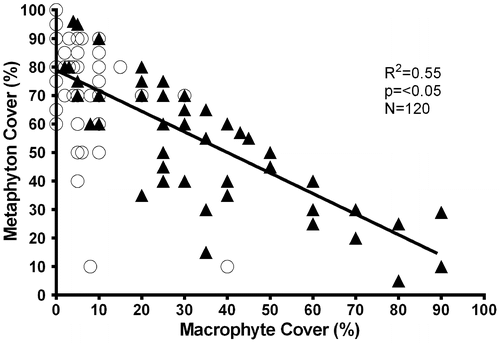
We collected 790 unique aquatic insects distributed in 48 genera, with 22 (48.5%) belonging to the order Dipterans, 9 (18.8%) to Odonata, 8 (16.7%) to Coleoptera, 5 (10.4%) to Hemipera and 4 (8.3%) to Ephemeroptera. The total genus data by site is presented in the Appendix 1. The most abundant genera were Coleopterans Enochrus (Hydrophilidae) and Laccophilus (Dysticidae), with 129 and 91 organisms, respectively. The highest number of unique organisms, 487, was collected in the dry season, followed by the cold rainy season (189 organisms) and the rainy season (114 organisms). Nevertheless, the cumulative curve shows that it is important to have higher sampling to identify all genera present in the system (Figure ).
Figure 4. Accumulation curve of genus of aquatic insects’ genus, SKBR, Quintana Roo, Mexico. Solid lines are the water bodies and the dotted lines are the range of 95% confidence.
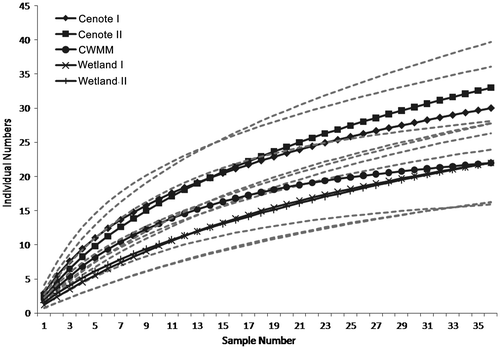
There were no noticeable trends in the change in the diversity values during the year, and the cenotes have higher diversity values than the wetlands (Table ). The insect diversity index showed a positive correlation with metaphyton coverage (R2 = 0.5, p = 0.01), while there were negative correlations between macrophyte coverage and insect richness (R2 = 0.2, p = 0.07, Figure ).
Table 3. Fisher’s alpha diversity index of aquatic insects for every season and annual in SKBR, Quintana Roo, Mexico.
Discussion
Cenotes have more stable physicochemical variables than wetlands because they are deep aquatic systems with permanent water sources along the year. Wetlands are temporal aquatic systems that only receive water during the rainy season. The small variation on abiotic factors in cenotes were well described in previously.[Citation60] Other studies show that permanent and temporary wetlands in Sian Ka’an present similar abiotic conditions in rainy seasons but differ significantly during dry season.[Citation39,60] Nevertheless, nutrients and productivity variables such as chlorophyll a were low without any differences in all systems and seasons.[Citation39]
The inverse relationship between macrophytes and metaphyton suggests a potential competitive exclusion pattern between these two types of primary producers.[Citation61–63] Generally, plants present different strategies for competitor exclusion. During this competition, both macrophytes and metaphyton may exude allelopathic substances that inhibit the growth of their competitor.[Citation64] The effect of allelopathy depends on the population density of the plants that produce the chemicals and the sensitivity of the plants that receive them as well as on environmental variables, such as the lack of nutrients.[Citation65,66]
The SKBR wetlands have a low diversity and abundance aquatic insect families compared to other tropical systems.[Citation67,68] This could be explained by the oligotrophic status of these water body types.[Citation40] A low level of nutrients is directly related to the diversity and abundance of aquatic biota in similar systems, such as the subtropical Everglades in Florida.[Citation68,69]
Macroinvertebrates abundance and richness increase with habitat complexity or morphology, as this is directly related to the number of microhabitats and therefore the number of biotic interactions and the greater availability of microclimates.[Citation1] In our case, emergent plants compose the dominant macrophyte lifestyle which usually support lower macroinvertebrate diversity than other types of macrophytes.[Citation19,70–72] This confirms that a less complex substrate may offer lower protection against predators and lower food sources.[Citation10,11] The metaphyton is a more complex structure formed by filamentous cyanoprokaryotes, diatoms, detritus and other components.[Citation45] This combination of elements make metaphyton wrinkled structures that produce more niches than emergent macrophytes, and therefore more aquatic insect diversity.[Citation27] Metaphyton are usually a major component in the diet of diverse aquatic insects.[Citation73] Nevertheless, the role of allochthones energy on aquatic insects on SKBR must be clarified, since in others studies in the same area but on fish community, the allochthones material is the most important mainly resource on their diet.[Citation64]
Although the primary producers are important sources for insects, sampled cenotes without primary producers showed similar diversity to wetlands. This lack in differences suggests that other factors are playing important roles in insect diversity in tropical wetlands. One of these factors appears to be ecological stability caused by the hydrological regime. The wetland hydroperiod is unstable with long periods lacking water,[Citation74] and wetland primary producers support only a limited insect larval community that is capable of surviving through these harsh dry-season conditions. These larvae are the initial seeds for repopulation during the following rainy season.[Citation75] In contrast, cenotes are a more stable system that allow the establishment of more stable aquatic communities compared with wetlands.[Citation39,40]
The relationship between primary producers and aquatic insects in the RSBK is complex. Macrophytes and metaphyton have a negative correlation, which suggests a competitive relationship that must be further explored in later studies. However, these interactions appear to affect the aquatic insect community; in areas where metaphyton was abundant, insect communities were also more abundant and diverse than in communities with emergent macrophytes. Coupled with primary production, water supply and aquatic system hydrodynamics appear to have a strong relationship with aquatic insect diversity and abundance. This study is a first approach in identifying this three-actor relationship in the tropical calcareous system of the SKBR.
Disclosure statement
No potential conflict of interest was reported by the authors.
Funding
This work was supported by the Consejo Nacional de Ciencia y Tecnología [grant number 165043].
Associate Editor: Blanca Rios-Touma
References
- Jeffries M. Invertebrate colonization of artificial pondweeds of differing fractal dimension. Oikos. 1993;67:142–148.10.2307/3545104
- Heino J. Lentic macroinvertebrates assemblage structure along gradients in spatial heterogeneity, habitat size and water chemistry. Hydrobiologia. 2000;418:229–242.10.1023/A:1003969217686
- Tews J, Brose U, Grimm V, et al. Animal species diversity driven by habitat heterogeneity/diversity: the importance of keystone structures. J Biogeogr. 2004;31:79–92.10.1046/j.0305-0270.2003.00994.x
- Feller IC, Mathis WN. Primary herbivory by wood-boring insects along an architectural gradiend of Rhizophora mangle. Biotropica. 1997;29:440–451.10.1111/btp.1997.29.issue-4
- Stewart AJA, John EA, Hutchings MJ. The ecological consequences of environmental heterogeneity. In: Hutchings MJ, John EA, Stewart AJA, editors. Environmental heterogeneity: effects on plants in restoration ecology. London: British Ecological Society Blackwell Science; 2000. p. 1–8.
- McAbendroth L, Ramsay PM, Foggo A, et al. Does macrophyte fractal complexity drive invertebrate diversity, biomass and body size distributions? Oikos. 2005;111:279–290.10.1111/oik.2005.111.issue-2
- Gregg WW, Rose FL. The effects of aquatic macrophytes on the stream microenvironment. Aquat Bot. 1982;14:309–324.10.1016/0304-3770(82)90105-X
- Dudley TL, Cooper SD, Hemphill N. Effects of macroalgae on a stream invertebrate community. J N Am Benthol Soc. 1986;5:93–106.10.2307/1467864
- Burdett AS, Watts RJ. Modifying living space: an experimental study of the influences of vegetation on aquatic invertebrate community structure. Hydrobiologia. 2009;618:161–173.10.1007/s10750-008-9573-z
- Warfe DM, Barmuta L. Habitat structural complexity mediates the foraging success of multiple predator species. Oecologia. 2004;141:171–178.10.1007/s00442-004-1644-x
- Thomaz SM, Dibble ED, Evangelista LR, et al. Influence of aquatic macrophyte habitat complexity on invertebrate abundance and richness in tropical lagoons. Freshwater Biol. 2008;53:358–367.
- Reynolds CS. The ecology of freshwater phytoplankton. New York (NY): Cambrige University Press; 1984.
- Reynolds CS. The ecology of phytoplankton. New York (NY): Cambrige University Press; 2006.10.1017/CBO9780511542145
- Harris G. Phytoplankton ecology: structure, function, and fluctuation. New York (NY): Springer; 1986.
- Van der Valk AG. The biology of freshwater wetlands. Oxford: Oxford University Press; 2012.10.1093/acprof:oso/9780199608942.001.0001
- Chambers PA, Lacoul P, Murphy KJ, et al. Global diversity of aquatic macrophytes in freshwater. Hydrobiologia. 2008;595:9–26.
- Thomas SM, Esteves FA, Murphy KJ, et al. Aquatic macrophytes in the tropics: ecology of populations an communities. Impacts of invasions and human use. In Claro KD, Oliveira PS, Rico-Gray V, et al., editors. International commission on tropical biology and natural resources. Tropical biology and conservation management Vol IV. Encyclopedia of life support systems (EOLSS). Oxford: Eolss; 2008. p. 27–60.
- Dos Santos AM, Thomaz SM. Short term fluctuations and succession trends in tropical floodplain vegetation measured by correspondence analysis. Braz Arch Biol Technol. 2008;51:781–791.
- Cremona F, Planas D, Lucotte M. Biomass and composition of macroinvertebrate communities associated with different types of macrophyte architectures and habitats in a large fluvial lake. Arch Hydrobiol. 2008;171:119–130.10.1127/1863-9135/2008/0171-0119
- Titus JE, Urban RA. Aquatic plants: a general introduction. In: Likens GE, editor. Encyclopedia of inland waters. Chicago (IL): Elsevier; 2009. p. 43–51.
- Walker PD, Wijnhoven S, van der Velde G. Macrophyte presence and growth form influence macroinvertebrate community structure. Aquat Bot. 2013;104:80–87.10.1016/j.aquabot.2012.09.003
- Schuyler AE. Classification of life forms and growth forms of aquatic macrophytes. Bartonia. 1984;50:8–11.
- Lot A, Novelo A, Olvera M, et al. Catálogo de angiospermas acuáticas de México: Hidrófitas estrictas emergentes, sumergidas y flotantes [Catalog of aquatic angiosperms of Mexico: emerging strict hydrophytes, submerged and floating]. Mexico: UNAM; 1999.
- Hansen JP, Wikström SA, Axemar H, et al. Distribution differences and active habitat choices of invertebrates between macrophytes of different morphological complexity. Aquat Ecol. 2011;45:11–22.10.1007/s10452-010-9319-7
- Tessier C, Cattaneo A, Pinel-Alloul B, et al. Invertebrate communities and epiphytic biomass associated with metaphyton and emergent and submerged macrophytes in a large river. Aquat Sci. 2008;70:10–20.10.1007/s00027-007-0920-3
- Batzer DP, Wissinger SA. Ecology of insect communities in nontidal wetlands. Ann Rev Entomol. 1996;41:75–100.10.1146/annurev.en.41.010196.000451
- Liston SE, Trexler JC. Spatiotemporal patterns in community structure of macroinvertebrates inhabiting calcareous periphyton mats. J N Am Bhenthol Soc. 2005;24:832–844.10.1899/04-067.1
- Chick JH, Geddes P, Trexler JC. Periphyton mat structure mediates trophic interactions in a subtropical marsh. Wetlands. 2008;28:378–389.10.1672/07-121.1
- Donar CM, Condon KW, Gantar M, et al. A new technique for examining the physical structure of Everglades floating periphyton mat. Nova Hedwigia. 2004;78:107–119.10.1127/0029-5035/2004/0078-0107
- Gaiser EE, Childers DL, Jones RD, et al. Periphyton responses to eutrophication in the Florida Everglades: cross-system patterns of structural and compositional change. Limnol Oceanogr. 2006;51:617–630.10.4319/lo.2006.51.1_part_2.0617
- Noe GB, Scinto LJ, Taylor J, et al. Phosphorus cycling and partitioning in an oligotrophic Everglades wetland ecosystem: a radioisotope tracing study. Freshwater Biol. 2003;48:1993–2008.10.1046/j.1365-2427.2003.01143.x
- Vadeboncoeur Y, Steinman AD. Periphyton function in lake ecosystems. Sci World J. 2002;2:1449–1468.10.1100/tsw.2002.294
- Azim ME, Verdegem MCJ, van Dam AA, et al. Periphyton ecology, exploitation and management. Oxfordshire: CABI; 2005.
- CONABIO. Reserva de la Biosfera de Sian Ka’an [Sian Ka’an biosphere]. Mexico: CONABIO; 1998.
- SEMARNAP. Programa de Manejo. Reserva de la Biosfera de Sian Ka’an [Environment and fisheries ministry- management program. Sian Ka´an biosphere reserve]. Mexico: SEMARNAP; 1993.
- Merdiz G. Ficha informativa de los Humedales de Ramsar: Bala’an K’aax [Information sheet on Ramsar Wetlands: bala’an K’aax]. Mexico: Ramsar; 2003.
- Bauer-Gottwein P, Gondwe BRN, Charvet G, et al. Review: the Yucatán Peninsula karst aquifer. Hydrogeol J. 2011;19:507–524.10.1007/s10040-010-0699-5
- Zambrano L, Vázquez-Domínguez E, García-Bedoya D, et al. Fish community structure in freshwater karstic water bodies of the Sian Ka’an Reserve in the Yucatan Peninsula. México Ichthyol Explor Freshwaters. 2006;17:193–206.
- Escalera-Vázquez LH, Zambrano L. The effect of seasonal variation in abiotic factors on fish community structure in temporary and permanent pools in a tropical wetland. Freshwater Biol. 2010;55:2557–2569.10.1111/fwb.2010.55.issue-12
- Schmitter-Soto JJ, Comín FA, Escobar-Briones E, et al. Hydrogeochemical and biological characteristics of cenotes in the Yucatan peninsula (SE Mexico). Hydrobiologia. 2002;467:215–228.10.1023/A:1014923217206
- Gondwe BRN, Hong SH, Wdowinski S, et al. Hydrologic dynamics of the ground-water-dependent Sian Ka’an Wetlands, Mexico, derived from InSAR and SAR data. Wetlands. 2010;30:1–13.10.1007/s13157-009-0016-z
- García-Bedoya, D. Distribución de la familia Poeciliidae a nivel regional en la Reserva de la Biosfera de Sian Ka’an [Regional distribution of Poeciliidae family in the Biosphere Reserve of Sian Ka’an]. Mexico: UNAM Dissertation; 2004.
- Olmstend I, Durán R. Vegetación de Sian Ka’an [Sian Ka’an vegetation]. In: Robinson DLJ, editor. Diversidad biológica de la reserva de la biosfera de Sian Ka’an Quintana Roo, México. Mexico: CIQRO; 1990. p. 1–12.
- Elías-Gutiérrez M, Cervantes-Martínez A, Gutiérrez-Aguirre M, et al. Los cenotes y lagunas del centro y sur de la península de Yucatán [The cenotes and lakes of central and southern Yucatan Peninsula]. In de la Lanza G, editor. Las aguas interiores de México: conceptos y casos. México: Agt Editor; 2007. p. 424–464.
- Ibarra C, Talavera R, Novelo E. Diversity and structure of periphyton and metaphyton diatom communities in a tropical wetland in Mexico. Rev Mex Biodivers. 2009;80:763–769.
- Goldsborough G. Sampling algae in wetlands. In: Rader R, Batzer D, Wissinger SA, editors. Bioassessment and management of North American freshwater wetlands. New York (NY): Wiley; 2001. p. 263–295.
- Merritt R, Cummins K, Berg MB. An introduction to the aquatic insects of North America. Dubuque: Kendall/Hunt; 2008.
- Cranston PS, Daly HV. General classification and key to the orders of aquatic and semiaquatic insects. In: Merrit RW, Cummins KW, Berg MB, editors. An introduction to the aquatic insects of North America. Dubuque: Kendall/Hunt; 2008. p. 157–1684.
- Courthey GW, Merrit RW. Aquatic dipteran. In: Merrit RW, Cummins KW, Berg MB, editors. An introduction to the aquatic insects of North America. Dubuque: Kendall/Hunt; 2008. p. 687–722.
- Ferrington LCJ, Coffman WP, Berg MB. Chironomidae. In: Merrit RW, Cummins KW, Berg MB, editors. An introduction to the aquatic insects of North America. Dubuque: Kendall/Hunt; 2008. p. 847–989.
- Mccafferty WP, Lugo-Ortiz CR, Provonsha AB, et al. Los efemerópteros de México: Clasificación superior, diagnosis de familias y composición [Mexico mayflies: higher classification, families composition and diagnosis]. Dugesiana. 1997;4:1–29.
- Merrit RW, Webb DW. Aquatic dipterans: pupae and adults of aquatic díptera. In: Merrit RW, Cummins KW, Berg MB, editors. An introduction to the aquatic insects of North America. Dubuque: Kendall/Hunt; 2008. p. 723–800.
- Novelo-Gutiérrez R. Clave para la separación de familias y géneros de las náyades de odonata de México. Parte I. Zygoptera [Key to the separation of families and genera of the nymphs of Odonata of Mexico. Part I. Zygoptera]. Dugesiana. 1997;4:1–10.
- Novelo-Gutiérrez R. Clave para la determinación de familias y géneros de las náyades de odonata de México. Parte II. Anisoptera [Key to the separation of families and genera of the nymphs of Odonata of Mexico. Part II. Anisoptera]. Mexico:Dugesiana. 1997;4:31–40.
- Polhemus JT. Aquatic and semiaquatic hemiptera. In: Merrit RW, Cummins KW, Berg MB, editors. An introduction to the aquatic insects of North America. Dubuque: Kendall/Hunt; 2008. p. 385–423.
- Tennesen kJ. Odonata. In: Merrit RW, Cummins KW, Berg MB, editors. An introduction to the aquatic insects of North America. Dubuque: Kendall/Hunt; 2008. p. 237–294.
- Wallace JL, Walker ED. Culicidae. In: Merrit RW, Cummins KW, Berg MB, editors. An introduction to the aquatic insects of North America. Dubuque: Kendall/Hunt; 2008. p. 801–823.
- Waltz RD, Burian SK. Ephemeroptera. In: Merrit RW, Cummins KW, Berg MB, editors. An introduction to the aquatic insects of North America. Dubuque: Kendall/Hunt; 2008. p. 181–236.
- White DS, Roughley RE. Aquatic coleopteran. In: Merrit RW, Cummins KW, Berg MB, editors. An introduction to the aquatic insects of North America. Dubuque: Kendall/Hunt; 2008. p. 571–671.
- Camargo-Guerra T, Escalera-Vázquez LH, Zambrano L. Fish community structure dynamics in cenotes of the Biosphere Reserve of Sian Ka’an, Yucatan peninsula, Mexico. Rev Mex Biodivers. 2013;84:901–911.10.7550/rmb.33019
- Thomas S, Gaiser EE, Gantar M, et al. Quantifying the responses of calcareous periphyton crust to rehydration: a microcosm study (Florida Everglades). Aquat Bot. 2006;84:317–323.10.1016/j.aquabot.2005.12.003
- Armentano TV, Sah JP, Ross MS, et al. Rapid responses of vegetation to hydrological changes in Taylor Slough, Everglades National Park, Florida, USA. Hydrobiologia. 2006;569:293–309.10.1007/s10750-006-0138-8
- Goldsborough LG, McDougal RL, North AK. Periphyton in freshwater lakes and wetlands. In: Azim ME, Verdegem MCJ, van Dam AA, et al., editors. Periphyton: ecology, exploitation and management. London: CABI; 2005. p. 71–89.
- Gopal B, Goel U. Competition and allelopathy in aquatic plant communities. Bot Rev. 1993;59:155–210.10.1007/BF02856599
- Gross EM, Feldbaum C, Graf A. Epiphyte biomass and elemental composition on submersed macrophytes in shallow eutrophic lakes. Hydrobiologia. 2003;506–509:559–565.10.1023/B:HYDR.0000008538.68268.82
- Hilt S. Allelopathic inhibition of epiphytes by submerged macrophytes. Aquat Bot. 2006;85:252–256.10.1016/j.aquabot.2006.05.004
- Nessimian JL, Venticinque EM, Zuanon J, et al. Land use, habitat integrity, and aquatic insect assemblages in central amazonian streams. Hydrobiologia. 2008;614:117–131.10.1007/s10750-008-9441-x
- Brown MT, Cohen MJ, Bardi E, et al. Species diversity in the Florida Everglades, USA: a systems approach to calculating biodiversity. Aquat Sci. 2006;68:254–277.10.1007/s00027-006-0854-1
- Sokol ER, Hoch JM, Gaiser E, et al. Metacommunity structure along resource and disturbance gradients in everglades wetlands. Wetlands. 2014;34:135–146.10.1007/s13157-013-0413-1
- Tessier C, Cattaneo A, Pinel-Alloul B, et al. Biomass, composition and size structure of invertebrate communities associated to different types of aquatic vegetation during summer in Lago di Candia (Italy). J Limnol. 2004;63:190–198.10.4081/jlimnol.2004.190
- Campeau S, Murkin HR, Titman RD. Relative importance of algae and emergent plant litter to freshwater marsh invertebrates. Can J Fish Aquat Sci. 1994;51:681–692.10.1139/f94-068
- Gerrish N, Bristow JM. Macroinvertebrate associations with aquatic macrophytes and artificial substrates. J Great Lakes Res. 1979;5:69–72.10.1016/S0380-1330(79)72129-0
- Voigts DK. Aquatic invertebrate abundance in relation to changing marsh vegetation. Am Midl Nat. 1976;95:313–322.10.2307/2424396
- Hershey AE, Lamberti GA, Chaloner DT, et al. Aquatic insect ecology. In: Thorp JH, Covich AP, editors. Ecology and classification of North American freshwater invertebrates. Amsterdam: Academic Press; 2010. p. 659–694.10.1016/B978-0-12-374855-3.00017-0
- Gottlieb A, Richards J, Gaiser E. Effects of desiccation duration on the community structure and nutrient retention of short and long-hydroperiod Everglades periphyton mats. Aquat Bot. 2005;82:99–112.10.1016/j.aquabot.2005.02.012
Appendix 1
Number of individuals by genus of aquatic insects in different sites during an annual cycle in SKBR, Quintana Roo, Mexico.
Note: CWMM = cenote without macrophyte or metaphyton.