ABSTRACT
A striking feature of most scorpion species is fluorescence under ultraviolet light, but few studies have investigated the adaptive benefit of this trait. A hypothesis is that fluorescence may lure prey towards the scorpion improving foraging success. In this study, we investigated whether the fluorescence of the scorpion Centruroides granosus Thorell, 1876 lures the house cricket Acheta domesticus Linnaeus, 1758. We performed two experiments: 1) an arena in which crickets were exposed to both fluorescing and black-painted non-fluorescing scorpions and 2) a tunnel in which crickets could walk out through one of the two exits, each with a scorpion treatment. None of the experiments provided evidence that the scorpion fluorescence is attractive to house crickets since they did not show preference for any of the two scorpion types. Variables such as cricket sex or scorpion sex did not influence their response towards the fluorescence; however, crickets were on average closer to male scorpions in the outdoor arena and closer to female scorpions in the laboratory arenas. Moreover, male crickets were more active than females in the tunnel experiment, in line with the results of the arena experiment showing that male crickets were on average closer to the scorpions in the laboratory. We discuss potential implications and suggest that more experimental work is required to investigate potential adaptive benefits of the scorpion fluorescence under various biotic and abiotic factors.
Introduction
A particular trait common to most scorpion species is fluorescence under UV light. However, it is unclear whether this trait has an adaptive function and the topic has received little attention from experimental studies. Some hypotheses have been proposed and the only ones that received experimental scrutiny include the use of the fluorescence to detect UV light [Citation1] and helping the individuals to avoid UV light [Citation2,Citation3], although the adaptive significance of the latter remained unclear. Some authors have proposed that fluorescence may reduce detection by prey and predators through camouflage [Citation2] but this hypothesis requires experimental evidence. The latter study by Blass and Gaffin [Citation2] exposed scorpions to different wavelengths of light (red, green, UV, or no light) and found avoidance of UV (and green) only when half of the arenas were illuminated. However, when the entire arena was illuminated, scorpions did not show avoidance for any of the type of light used. The biological relevance of exposing scorpions to isolated wavelength it is debatable; since nocturnal light is composed of all of them in different proportions [Citation4].
Another hypothesis proposes that fluorescence may help scorpions to lure prey but only one study has tested this idea under natural light conditions [Citation5]. That study investigated whether aerial insects were attracted to fluorescent scorpions, but contrarily to the hypothesis, it found that aerial insects avoided fluorescing scorpions during the full moon as compared to non-fluorescing scorpions (coated with UV-blocking varnish). Moreover, the study found no preference of aerial insects for any of the two types of scorpions when the experiments were carried out during the new moon phase, indicating that moonlight intensity influenced the response of the prey.
Here, we investigated the “prey-attraction hypothesis” for fluorescence in the scorpion Centruroides granosus Thorell, 1876, a tropical species from Panama. Different from Kloock [Citation5] who used a scorpion species that feeds largely on aerial insects, we tested this hypothesis using crickets and a species of scorpion that preys mostly on terrestrial prey [Citation6]. Our study species cohabits environments with macrofungi () that fluoresce similar to scorpions, at least to the human eye. If fungi with autofluorescence (induced by UV light) attract arthropods to disperse their spores, similar to fungi with bioluminescence [Citation7], then we hypothesize that scorpions may use deceiving signals to lure prey. In analogy with diurnal predators, orb-weaving spiders use deceiving signals resembling food sources (e.g. UV patterns in flowers) to attract insects to the web [Citation8,Citation9]. Some nocturnal spiders also use their body coloration to lure prey [Citation10–12].
Figure 1. Fluorescence of fungi and scorpion cohabiting the study site. a) Unknown macrofungi (scale bar: 2 cm), b) fungus Trametes sp. (scale bar: 1 cm) and c) Centruroides granosus. (scale bar: 2.5 cm). The light intensity of the UV lamp varied across pictures
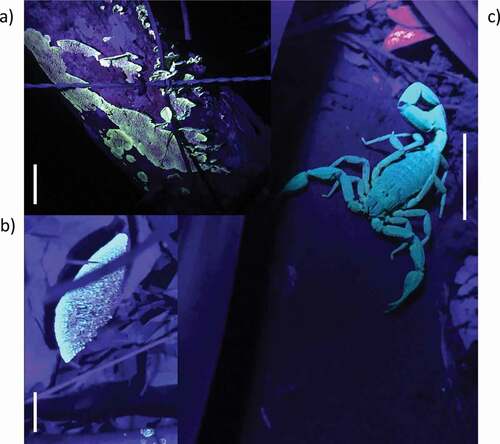
Given the apparent avoidance responses of insects towards fluorescing scorpions during full moon [Citation5], here, we focused on conducting the experiments during non-full moon phases, particularly during the third quarter phase. In addition, to test whether the light source (artificial vs natural) influences the response of the crickets towards the scorpions, we performed experiments in arenas both in the laboratory and outdoors. Moreover, we carried out a selection tunnel experiment in the laboratory in which crickets could walk out of the tunnel through one of the two exits: one with a caged fluorescing scorpion and the other one with a caged non-fluorescing scorpion. Our work is just the second study in the literature to experimentally investigate the capability of the scorpion fluorescence to lure prey and the first study carried out on a tropical species.
Methods
We collected individual of Centruroides granosus scorpions from a dirt road in the town of Polanco in the district of Capira, Panama (08°45ʹ44.3196”, −079°48ʹ22.8618”). We fed all individuals with the house cricket Acheta domesticus once a week before the experiments. This type of prey is promptly accepted by C. granosus [Citation6] and it was also used in the experiments. Information on the visual capacity of A. domesticus is scarce but they are likely to show remarkable night vision similar to other nocturnal crickets [Citation13] and insects [Citation14]. Moreover, A. domesticus seems to respond to fluorescent lights [Citation15] and light intensity plays a role in phonotaxis of females [Citation16]. For all experiments, we compared pairs of fluorescing and non-fluorescing scorpions of similar body size. Non-fluorescing scorpions were painted with a black marker (xylene and toluene free, Pentel N450), which blocked the fluorescence to the human eye, while fluorescing scorpions remained untreated.
Arena experiment
In the laboratory, each arena consisted of a pair of fluorescing and non-fluorescing live scorpions of the same sex (n = 24, 12 replicates per sex) that were placed next to each other in cages (8 cm in diameter, 3 cm in height, C in )). The openings of the cage had an area of 1.2 cm2 which did not impede the detection of the scorpions and avoided contact between the crickets and scorpions. Centruroides granosus remains completely immobile while in the hunting position. Scorpions were placed on one side of the arena and three crickets of the same sex were released on the opposite side (triangle in )). The floor of the arenas was covered with manila paper for each trial. Arenas were illuminated with 100 W Moonlite bulbs (Zoo Med) placed at 2 m above them, mimicking moonlight. The light of these bulbs covers the UV spectrum (Figure S1). Pictures of the arenas were taken with night vision cameras every 10 seconds for 10 minutes. Experiments performed outdoors consisted of the same setup and used natural moonlight (third quarter) as illumination instead of the bulbs. From each picture, we obtained the average distance of the three crickets to each of the two scorpions. We had equal proportion of scorpion treatments assigned to each cage side (left or right from the crickets’ perspective).
Figure 2. Experimental design to test the prey-attraction hypothesis for the scorpion fluorescence. a) View from above of the arena in which crickets were exposed to fluorescing and non-fluorescing scorpions. b) Trial tunnel in which crickets were exposed to the scorpion treatments. For both experiments, scorpions were contained in cages (C, 8 cm in diameter, 3 cm in height). The mesh of the cages is not shown for simplicity. Crickets were released at one side of the tunnel (R, 1.8 cm in diameter) and could walk out of the tunnel through one of the exits (E, 11 cm in diameter) in the opposite site. See text for more details
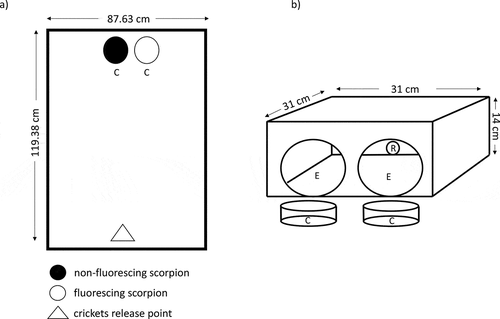
Tunnel experiment
We used 10 crickets of the same sex for each pair of scorpions (fluorescing vs non-fluorescing) and the sex of the crickets was alternated after each trial (n = 24, 12 pairs of each scorpion sex, 10 crickets per replicate). Crickets were released into the tunnel through a hole in one end of the tunnel (R in )) and could walk out through one of the exits at the end (E in )). The exits were illuminated with the same moonlite bulb and scorpions were placed in the same cages used for the arena experiments. We quantified a multinomial categorical response by the crickets, where 0: no response, the cricket did not select any of the exits after 3 minutes; 1: cricket walked through the exit facing the non-fluorescing scorpion; 2: cricket walked through the exit facing the fluorescing scorpion. In addition, we measured the time crickets took to select one of the two exits. The position of the scorpions was switched after every two crickets (5 times for 10 crickets per replicate).
Statistical analysis
We performed all analysis in R [Citation17]. We used repeated-measures ANOVA in order to analyze whether the average distances of the crickets to the two scorpions varied along time, as implemented in the libraries “nlme” and “companion” (Scripts S1). We included scorpion treatment, cricket sex, scorpion sex, experimental setup (laboratory vs outdoor) as explanatory variables and evaluated full interactions. For the tunnel experiment, we carried out a binomial test to examine whether the proportion of crickets walking to each scorpion treatment varied (excluding non-responsive crickets). Moreover, we performed a multinomial logistic regression with a categorical response variable (0: no response, 1: non-fluorescing scorpion, 2: fluorescing scorpion) as implemented in a generalized linear model to test whether the probability of walking towards the fluorescing scorpion was influenced by cricket sex, scorpion sex and scorpion pair per trial. Given the non-normality of time data in the tunnel experiment, we used a Wilcoxon test to compare the time spent by the responsive crickets (1 or 2 from the multinomial response) to walk to each scorpion.
Results
Arena experiment
Overall, there was no difference between the mean distances of the crickets from the fluorescing and non-fluorescing scorpions (). There were no effects of cricket sex and experimental setup on the mean distance of the crickets ( b and c, respectively). There was an interaction between cricket sex and experimental setup, indicating that in the laboratory, male crickets were on average closer to the scorpions as compared to female crickets (, cricket sex: F1,2864 = 34.8, p < 0.0001). For outdoor arenas, male crickets tended to be further from scorpions than females (; cricket sex: F1,2864 = 11.9, p < 0.001). There was no evidence indicating that the effect of the fluorescence depended on the experimental setup ().
Table 1. Summary of repeated-measures ANOVA of the average distances of the crickets related to treatment, cricket sex (Cricket), experimental setup (Setup), time and scorpion sex (Scorpion). For clarity, non-significant two-way interactions are not shown with the exception of the interaction Treatment: cricket sex, Treatment: Experimental setup, Treatment: Time and Treatment: Scorpion sex. Descriptive statistics indicate mean ± standard deviation. For simplicity, we have not added the mean and standard deviations for the variable Time (60 values) or the interaction Treatment: Time (120 values). Top right of each row shows the statistical analysis (F and p values) for the variable or interaction
There was an effect of time (), indicating variation of the average distances along time; however, the response of the crickets towards the two types of scorpions did not vary along time ().
Crickets were on average closer to male scorpions than female scorpions (). Moreover, there was an interaction between scorpion sex and experimental setup indicating that crickets tended to be on average closer to female scorpions in the laboratory arenas (, scorpion sex: F1,2864 = 10.8, p = 0.001). For outdoor arenas, the trend was the opposite, so that crickets tended to be closer to male scorpions (, scorpion sex: F1,2864 = 51.4, p < 0.0001).
There was no interaction between treatment and cricket sex indicating that the fluorescence did not influence the sexes of crickets differently (). Neither there was an interaction between treatment and scorpion sex, indicating that the fluorescence was not more effective for one of the scorpion sexes ().
Finally, there was an interaction between cricket sex and scorpion sex showing that male crickets were on average closer to male scorpions than female scorpions (, scorpion sex: F1,2875 = 28.1, p < 0.0001). There were no differences in the mean distance of female crickets to the male or female scorpions (, scorpion sex: F1,2875 = 27.1, p = 0.08).
Tunnel experiment
Crickets did not walk out of the tunnel preferentially through the exit with the fluorescing scorpion as compared to the non-fluorescing scorpion (binomial test, 26 successes in 240 trials, p > 0.05: 26 chose fluorescing scorpions, 16 chose non-fluorescing scorpion and 198 non-responsive crickets). This result was not influenced by the sex of the scorpion (GLM, t = – 0.25, p = 0.76), the sex of cricket (GLM, t = 0.93, p = 0.35) or the pair of scorpions (GLM, t = 0.53, p = 0.6). The probability of a cricket walking towards the fluorescing scorpions did not depend on its sex and the sex of the scorpion (GLM, interaction cricket sex: scorpion sex, t = 0.62, p = 0.54). Male crickets tended to be more responsive in the tunnel than females towards both types of scorpions (GLM, t = 1.973 p = 0.05). There was no difference in time spent by crickets to walk to either the fluorescing or the non-fluorescing scorpion (81.2 ± 59.4 vs 87.2 ± 68.6 s; Wilcoxon test = 216, p = 0.8).
Discussion
We did not find evidence that the scorpion fluorescence was attractive to crickets in the two experiments, either in laboratory or outdoor conditions. This is in line with previous studies [Citation5] that performed experiments during the new moon. As nocturnal insects, crickets are possibly able to see the scorpions in dim light [Citation18,Citation19]; however, this does not predict what the response towards the fluorescence would be (e.g. avoidance or attraction). It is likely that the visual signal produced by scorpions is not enough to generate a response in the crickets. Perhaps detection is only possible when the scorpions move [Citation20], and in that case, it should not lure the crickets but warn them.
Evidence suggests multimodal communication in crickets since they also use non-visual signals in their communication like pheromones [Citation21], tactile [Citation22], chemical [Citation23,Citation24] and acoustic stimuli [Citation25]; therefore, an isolated visual cue might not be enough to deceive crickets [Citation11]. Our results also indicate that the response of the crickets towards the fluorescence was neither influenced by their sex nor the sex of the scorpion. Future experiments should evaluate variables that affect light intensity in natural environments (e.g. habitat, canopy openness, season, lunar altitude or phase [Citation4]) and that may consequently influence the behavior of scorpions, their prey and their predators. For instance, moonlight detection through fluorescence [Citation1] induces shelter seeking [Citation2]; then, scorpions under a dense forest canopy and lower moonlight detection may be under higher predation risk.
We also found that cricket males in the tunnel tended to be more responsive towards both types of scorpions, which could be simply an innate behavior of males (e.g. males may show more exploratory behaviors) or perhaps female crickets are more capable of detecting predator signals and consequently reducing their activity. For instance, A. domesticus females delay their foraging in the presence of a mammal predator odor [Citation26]. Another potential chemical cue that may have influenced our results is that scorpions were fed with A. domesticus before the experiments and there is evidence that A. domesticus avoid chemical cues produced by predators fed on A. domesticus, as compared to the same predators fed on other insects [Citation27]. Perhaps this weakened any potential luring by the fluorescence. Overall, it seems that predator avoidance in house crickets depends on chemical cues [Citation26–28]. Whether scorpions emit a chemical signal detectable (e.g. faeces) by crickets remains to be investigated and perhaps it may shed some light on why male crickets were on average closer to male scorpions, or why they were closer and reacted more to the scorpions in the laboratory settings (arena and tunnel, respectively), while female crickets were on average closer in the outdoors setting (arena). Further experiments are required to test whether some of these trends persist in the absence of scorpions for both experiments.
Our results do not support the idea that scorpions are able to produce a deceiving signal for prey as the result of a coevolving prey–predator interaction. However, our study system involved species that did not co-evolve since their origins occurred on different continents, A. domesticus is native to Asia [Citation29] while C. granosus is from America [Citation30], which implies that Centruroides may not have developed strategies to “lure” A. domesticus. Therefore, future studies should use co-evolving species of prey and predator (e.g. tenebrionid beetles – vaejovid scorpion [Citation31]) to test whether this is a limiting condition to detect an effect of the scorpion fluorescence or any other potential mean of signaling.
It continues to be intriguing why fluorescence occurs in nocturnal predatory arachnids such as scorpions [Citation32,Citation33], harvestmen [Citation34] and spiders [Citation35]. Comparisons across these taxa may help establish similarities and differences in order to understand the evolution of this trait. Alternately, it is certainly possible that fluorescence has no function and be merely a byproduct of physiological processes conserved across these taxa. Overall, more experimental work is needed to investigate other potential functions of the fluorescence (aposematic signal, intra- or interspecies recognition). For instance, if predators use cues associated with bright moonlight nights to find scorpions [Citation36], the idea that scorpions use their own fluorescence as a signal to seek shelter [Citation2] and avoid predation seems conceivable.
Supplemental Material
Download Zip (311.3 KB)Acknowledgments
We thank Roberto Miranda, Fermin Gil and Milagro Garrido for the support in the field and laboratory. This study was supported by the Sistema Nacional de Investigación (DG).
Disclosure statement
The authors have no competing interests to declare.
Supplementary material
Supplemental data for this article can be accessed here.
Additional information
Funding
References
- Kloock CT, Kubli A, Reynolds R. Ultraviolet light detection: a function of scorpion fluorescence. J Arachnology. 2010;38(3):441–445. .
- Blass GRC, Gaffin DD. Light wavelength biases of scorpions. Anim Behav. 2008;76(2):365–373.
- Gaffin DD, Bumm LA, Taylor MS, et al. Scorpion fluorescence and reaction to light. Anim Behav. 2012;83(2):429–436.
- Veilleux CC, Cummings ME. Nocturnal light environments and species ecology: implications for nocturnal color vision in forests. J Exp Biol. 2012;215(3):4085–4096.
- Kloock CT. Aerial insects avoid fluorescing scorpions. Euscorpius. 2005;21:1–7.
- Miranda R, Bermúdez S, Cleghorn J, et al. Presas de escorpiones (Arachnida: scorpiones) de Panamá, con observaciones sobre el comportamiento de depredación. Revista Ibérica de Aracnología. 2015;27:115–123.
- Sivinski BYJ. Arthropods attracted to luminous fungi. Psyche. 1981;88(3–4):383–391.
- Cheng R-C, Yang E-C, Lin C-P, et al. Insect form vision as one potential shaping force of spider web decoration design. J Exp Biol. 2010;213:759–768.
- Herberstein ME, Craig CL, Coddington JA, et al. The function significance of silk decorations of orb-web spiders: a critical review of the empirical evidence. Biol Rev. 2000;75(4):649–669.
- Blamires SJ, Lai CH, Cheng RC, et al. Body spot coloration of a nocturnal sit-and-wait predator visually lures prey. Behav Ecol. 2012;23(1):69–74.
- Chuang C-Y, Yang E-C, Tso I-M. Diurnal and nocturnal prey luring of a colorful predator. J Exp Biol. 2007;210:3830–3837.
- Tso IM, Liao CP, Huang RP, et al. Function of being colorful in web spiders: attracting prey or camouflaging oneself? Behav Ecol. 2006;17(4):606–613.
- Zufall F, Schmitt M, Menzel R. Spectral and polarized light sensitivity of photoreceptors in the compound eye of the cricket (Gryllus bimaculatus). J Comp Physiol A. 1989;164(5):597–608.
- Warrant EJ. The remarkable visual capacities of nocturnal insects: vision at the limits with small eyes and tiny brains. Philos Trans R Soc B. 2017;372(1717):20160063.
- Chu C, Chen T-Y, Henneberry T. Attractiveness of flickering and non-flickering cool white fluorescent light to Culex quinquefasciatus (Diptera: culicidae), Musca domestica (Diptera: muscidae) and Pectinophora gossypiella (Lepidoptera: gelechiidae) adults, and Acheta domesticus (Orthoptera: Gryllidae) and Periplaneta americana (Blattodea: blattidae) nymphs. Southwest Entomol. 2006;31(1):77–81.
- Sik Ahn JH. Light intensity and time of day influence female acheta domesticus phonotaxis. Andrews University; 2013. Berrien Springs, Michigan.
- Team RDC. R: a language and environment for statistical computing. R Foundation for Statistical Computing: Vienna, Austria; 2019.
- Honkanen A, Immonen EV, Salmela I, et al. Insect photoreceptor adaptations to night vision. Philos Trans R Soc B. 2017;372(1717):20160077.
- Zagorski ER, Merry JW. How do eye size and facet lens size vary by age and sex in Acheta domesticus? Bios. 2014;85(3):151–159.
- Childs AM. Sensory modalities underlying the escape response of the cricket, Acheta domesticus, to looming stimuli. James Madison University; 2016. Harrisonburg, Virginia.
- Kortet R, Hedrick A. The scent of dominance: female field crickets use odour to predict the outcome of male competition. Behav Ecol Sociobiol. 2005;59(1):77–83. .
- Rek P. Does mating experience of male house crickets affect their behavior to subsequent females and female choice? Behav Ecol Sociobiol. 2012;66(12):1629–1637.
- Hardy T, Shaw K. The role of chemoreception in sex recognition by male crickets: Acheta domesticus and Teleogryllus oceanicus. Physiol Entomol. 1983;8(2):151–166.
- Shephard AM, Aksenov V, Rollo CD. Conspecific mortality cues mediate associative learning in crickets, Acheta domesticus (Orthoptera: gryllidae). J Orthopteran Res. 2018;27(2):187–192. .
- Gray DA. Female house crickets, Acheta domesticus, prefer the chirps of large males. Anim Behav. 1997;54(6):1553–1562.
- Tanis BP, Bott B, Gaston BJ. Sex-based differences in anti-predator response of crickets to chemical cues of a mammalian predator. PeerJ. 2018;6(6):e4923.
- Hoefler CD, Durso LC, McIntyre KD. Chemical-mediated predator avoidance in the European house cricket (Acheta domesticus) is modulated by predator diet. Ethology. 2012;118(5):431–437.
- Storm JJ, Lima SL. Predator-naïve fall field crickets respond to the chemical cues of wolf spiders. Can J Zool. 2008;86(11):1259–1263.
- Walker TJ. House Cricket, Acheta domesticus (Linnaeus) (Insecta: orthoptera: gryllidae). 1999. Gainesville, Florida / University of Florida - IFAS Extension.
- Fet V, Sissom W, Lowe G, et al. Catalog of the Scorpions of the World (1758–1998). 2000. New York / The New York Entomological Society.
- Polis GA. Prey and feeding phenology of the desert sand scorpion Pamroctonus mesaensis (Scorpionidae: vaejovidae). J Zool. 1979;188(3):333–346.
- Lawrence R. Fluorescence in arthropoda. J Entomol Soc South Afr. 1954;17(2):167–170.
- Pavan M. Presenza e distribuzione di una sostanza fluorescente ne tegumento degli scorpioni. Bollettino della Società Italiana di Biologia Sperimentale. 1954;30(7):801–803.
- Acosta L. Sobre la fluorescencia del tegumento en Opiliones (Arachnida). Hist Nat. 1983;3(23):192–195.
- Andrews K, Reed SM, Masta SE. Spiders fluoresce variably across many taxa. Biol Lett. 2007;3(3):265–267.
- Skutelsky O. Predation risk and state-dependent foraging in scorpions: effects of moonlight on foraging in the scorpion Buthus occitanus. Anim Behav. 1996;52(1):49–57.