ABSTRACT
In mountain ecosystems, temperature and precipitation change along elevation and determine the lower and upper limits of tree species which form forests. Early life performance of tree species has been assessed along elevational gradients as a proxy for several environmental variables. Here, we attempt to answer the following question: How does elevation provenance influence seed mass, viability, and germination of Queñoa (Polylepis spp.)? We worked with four species from two contrasting environments from northwest Argentina. We gathered seeds along elevation gradients, seeds were weighed, tested for viability (Triphenyltetrazoliumchlorid; TTC) and germination percentage in a controlled environment. The analysis was performed using Generalized Linear Models (GLM). We found low germination percentages in all species, probably related to low seed viability. The two species from humid environments had lower seed mass and higher germination percentages than the two species from dry environments. The effect of elevational provenance negatively affected the germination of two species, while the other two species were not affected. We conclude that although the four species studied belong to the same genus, their early life characteristics differ between environments and along elevational gradients according to species. Trends regarding future temperatures suggest increases in germination for two of our study species and no effects on the other two species.
Resumen
En los ecosistemas de montaña, la temperatura y la precipitación cambian a lo largo de la elevación y determinan los límites superior e inferior de las especies de árboles que forman los bosques. El rendimiento en la vida temprana de las especies de árboles de montaña se ha evaluado a lo largo de gradientes de elevación como proxy de varias variables ambientales. En este trabajo contestamos la siguiente pregunta: ¿Cómo influye la elevación sobre la masa, la viabilidad y la germinación de las semillas de Queñoa (Polylepis spp)? Trabajamos con cuatro especies de dos ambientes contrastantes del noroeste argentino. Recolectamos semillas a lo largo de gradientes de elevación y probamos la viabilidad (cloruro de trifeniltetrazolio; TTC) y la germinación (ambiente controlado). Pesamos las semillas e incluimos la variable individuo para desarrollar modelos lineales generalizados (GLM). Encontramos bajos porcentajes de germinación en todas las especies, probablemente relacionados con la baja viabilidad de la semilla. Encontramos diferencias significativas entre los porcentajes de germinación de ambientes contrastantes. El efecto de la procedencia elevacional afectó negativamente la germinación de dos especies, mientras que las otras dos especies no se vieron afectadas. Concluimos que aunque las cuatro especies estudiadas pertenecen al mismo género, sus características de vida temprana difieren entre ambientes y a lo largo de gradientes de elevación según la especie. Las tendencias con respecto a las temperaturas futuras sugieren aumentos en la germinación para dos de las especies de nuestro estudio y ningún efecto en las otras dos especies.
PALABRAS CLAVE:
Introduction
Elevational and latitudinal gradients are the main drivers of the global diversity patterns [Citation1,Citation2] and they are used as proxy for several environmental variables (namely temperature, UV exposure and precipitation). Temperatures and precipitation change along elevation and determine tree species distribution ranges [Citation3,Citation4]. Climate constraints seed production and explains the absence of trees at high elevations according to the “reproduction hypothesis” [Citation5]. Various environmental variables, as temperature and precipitation, influence seed germination and seedling emergence directly [Citation6], but also indirectly by influencing seed mass [Citation7]. In contrast to several studies addressing tree regeneration at upper treelines [Citation8,Citation9], lower distribution limits are not profoundly studied, but it is assumed that they are determined by inter-specific competition rather than by climate [Citation10].
Seed and fruit traits play a central role on understanding ecological processes and how global environmental change will impact plants and ecosystems [Citation11]. In particular, seed mass is a critical character of a plant’s life history as it may highly influence the regeneration process of a population [Citation12,Citation13]. Increasing seed mass within species was shown to correlate with an increase in seed germination [Citation14,Citation15], but not always [Citation16]. Particularly in adverse conditions or with high competition pressure, larger seeds can have a higher establishment success, as they provide more reserves for seedlings [Citation17]. Lighter seeds, instead, would be able to have larger dispersal distance [Citation17,Citation18].
At mid- and high-elevation regions of the Andean Cordillera of South America, from Venezuela to central Argentina, forests are dominated by 28 species of the genus Polylepis Ruiz & Pavon [Citation19]. Like other mountain forests, Polylepis forests offer valuable environmental services and they harbour a rich endemic biota [Citation20,Citation21]. The elevational distribution ranges of Polylepis species are defined by both land-use and climatic factors, with the lower elevation limits being shaped mainly by climate and competition, but also by anthropic factors [Citation22]. Human activities as logging, fire making or cattle raising [Citation23] as well as several climatic factors [Citation21] as temperature [Citation24] and precipitation [Citation25] control the upper distribution limit. Polylepis forests are increasingly at risk by habitat loss, fragmentation, and degradation, mining activities, cattle grazing and fire, and they face future threats from climate change [Citation26,Citation27].
The natural regeneration of the different Polylepis species is jeopardized by low germination (˂25%) for P. australis [Citation2,Citation28] and extremely low (˂10%) germination for P. neglecta, P. incarum and P. pacensis [Citation29]. The large production of non-viable seeds has been attributed mainly to parthenocarpy and resource limitation [Citation28,Citation30]. Reforestation programs are limited by scarce knowledge about the ecology of the species, particularly information about reproductive biology, seed germination and seedling habitat requirement [Citation31,Citation32].
The geographic and climatic variability of the Andean Cordillera creates conditions where organisms develop special adaptations [Citation33]. Some species (for example, P. tomentella Wedd. and P. tarapacana Phil.) withstand a high amplitude of temperatures, in semi-arid or arid environments in the southern tropical Andes [Citation34]. Other species, as P. australis and P. hieronymi, grow in more humid environments such as subtropical clouded forests [Citation27]. Climate change can affect precipitation and temperature belts in mountain regions. Elevational gradients heavily influence the general performance of mountain tree species [Citation1] and treeline ecotones are expected to be among the systems most susceptible to the expected global climate changes worldwide [Citation35].
In northwest Argentina two main climate types can be delimited: a cold arid or semi-arid climate in the western areas with higher elevations and warmer subtropical climate in the eastern lower elevations areas of the region [Citation36]. Global scenarios available in Chelsa (http://chelsa-climate.org) predict warmer annual conditions and a decrease in annual precipitations for northwest Argentina. In this work, we aim to answer the following question: How does elevation influence the seed mass, viability, and germination of Queñoa (Polylepis spp.) originating from two contrasting environments; P. tarapacana and P. tomentella of semi-arid environments and P. australis and P. hieronymi of humid forests? We expect that our study can give insights to the potential tree distribution shifts in response to climate change and we expect to provide information that may aid tree production for forest restoration purposes.
Methods
Study area
Our study area comprised the mountain range of the Central Andes (between −25 and −21° of latitude) in the Jujuy province of Argentina, considering three different eco-regions: Puna, High-Andes and Yungas [Citation37,Citation38].
The Puna ecoregion ranges between 3200 and 3700 m asl. The climate is cold and dry with intense solar radiation and large daily and seasonal thermal variations. Mean annual temperature varies from 5 to 7°C, with periods of night frost between March and October. Rainfall is scarce and largely seasonal, mean annual precipitation is 250 mm [Citation39]. P. tomentella is present in the Puna ecoregion.
The High-Andes encompasses all the mountainous areas that are higher than the Puna region and range in general between 3700 and 5000 m asl but the highest peaks can exceed 6000 m asl and have permanent snow. Mean annual temperature varies from 0 to 4° and monthly average temperatures tend to be below zero degrees for more than half of the year. There is high solar radiation, the thermal amplitude is very large and mean annual precipitation is about 190 mm [Citation38]. The only arboreal component is P. tarapacana, which generally grows as a shrub.
In contrast, P. australis and P. hieronymi appear in the subtropical Yungas ecoregion or Tucuman-Bolivian Forests where rainfall ranges from 700 to 2000 mm/year [Citation31]. Yungas are located on the eastern hillside of the Andes, ranging in elevation from 400 to 3400 m asl. Polylepis species appear in the highest parts of this ecoregion in the Open Woodlands (2500–3000 m asl) and they sometimes appear above the treeline in the Foggy Montane Grasslands (˃3000 m asl). Yungas are seasonal with discontinuous precipitation regimes [Citation40] and 80% of rain falls during summer. The Yungas ecosystem is considered vulnerable [Citation41] as more than one-half of the original forest has disappeared, due to logging, agriculture, exotic Pinus invasion, road development, human colonization and tourism [Citation42].
Species characteristics
The genus Polylepis belongs to the Rosaceae family and is distributed along the Andes from Venezuela to Northern Chile and in the Sierras de Córdoba in central Argentina. The fruit is an achene (hereafter called seed), with different types of protrusions that appear irregularly over the surface. We selected four Polylepis species present in northwest Argentina to study contrasting ecological situations at a relatively small distance. P. australis are small trees (mean height in the study area = 5.9 m), the glabrous leaves have 7 leaflets. The aches have undulating small ridges. The species conservation status is not reported by the International Union of Conservation Network (https://www.iucnredlist.org/). P. hieronymi, assessed as Vulnerable by the IUCN, grows as bushes or small trees (mean height = 2.1 m), its leaves have 9 leaflets that are very tomentose. The seeds have spiny ridges. P. tarapacana grows as patches of small bushes, has 3 glabrous leaflets per leaf and seeds have tiny hooks. The species is classified as Near Threatened and inhabits the High-Andes. P. tomentella are small trees (mean = 3.4 m), and each tomentose leave has 5 leaflets. The ridges of the seeds are almost absent. The species was assessed as Near Threatened by the IUCN.
Seed collection
Seeds were collected during December 2017–January 2018 (P. australis and P. tomentella), November–December 2018 (P. hieronymi) and December 2019 (P. tarapacana) at four locations situated 125 ± 12 km from each other: Yala (24.09112°S; 65.50431°W) for P. australis, San Bernardo (23.79700°S, 65.31133°W) for P. hieronymi, both in the Yungas ecoregion. For P. tarapacana, we collected seeds at Lagunillas del Farallón (22.32640° S, 66.07960° W) in the High-Andes and for P. tomentella in Quebraleña (23.28077°S; 65.76641°W) in Puna. All locations are in the Jujuy province in the northwest of Argentina (). At each location, we established an elevational transect including the entire local elevation gradient of the species. We collected seeds ad libitum from different trees accordingly to seed offer. The number of trees sampled per gradient, number of forest fragments, elevational range, and mean number of seeds collected per tree is indicated in . We only selected trees with a mature seed offer of 25% of the total crown (visually estimated). We selected seeds based on criteria of coloration (fruits of light brown colour) and degree of adhesion (easily removable). The collection of seeds was done manually. Because germination varies highly for P. australis [Citation43], we individualized each tree and incorporated individual tree as a variable in all studied species.
Table 1. The number of trees sampled per gradient, number of forest fragments, elevational range, and mean number of seeds collected per tree
Physical and physiological quality
We weighed 100 seeds per tree. Seeds were separated manually and weighed in an analytical balance. For P. australis, P. hieronymi and P. tarapacana, we tested the viability of applying Triphenyltetrazoliumchlorid (TTC) [Citation44] to 50 seeds of each tree. Due to the small amount of P. tomentella seeds collected we did not measure viability, but prioritized germination experiments. For all four species, we measured germination (%) in germination chambers under controlled environmental conditions of humidity, temperature, and light. We performed germination experiments according to availability, sowing 30 seeds per Petri dish. For each tree of P. australis and P. hieronymi, we used 4 Petri dishes per treatment, with a total of 156 and 216 Petri dishes, respectively, or a total of 4680 seeds for P. australis and 3120 seeds for P. hieronymi. For P. tarapacana, we used 3 Petri dishes per treatment per tree, with a total of 72 Petri dishes and 3150 seeds. For P. tomentella, we used 1, 2 or 3 Petri dishes per treatment with a total of 54 Petri dishes and 1590 seeds. Preliminary studies with a small amount of seeds from a previous year (Cuyckens and Hensen, unpublished data) for P. australis and P. hieronymi testing four treatments: 1. 8°C/4°C (day/night), 2. 20°C/10°C (day/night), 3. 32°C/20°C (day/night) and 4. at a constant of 23°C, indicated that germination was highest for treatment 2 and 3 and nearly 0 for treatment 1. For P. australis, temperatures of 20°C/10°C are known to reflect optimum temperatures [Citation43]. No references exist on optimum temperatures for P. tarapacana and P. tomentella. For other species in arid environments (P. neglecta, P. incarum y P. pacensis) Vega et al. [Citation29] used a constant temperature of 25°C and Gareca et al. [Citation45] used a constant of 16.6°C for P. subtusalbida. We selected a temperature and light regime with daily changes because constant temperature and continuous light conditions are not encountered frequently in nature and these conditions are not recommended in experimental conditions [Citation44]. For logistic reasons, we used the same temperature treatments for all the species. Each germination experiment had 45 days of duration. During this period, germinated seeds and non-germinated seeds were recorded twice weekly.
Data analysis
To compare seed mass, viability, and germination between species, we performed Analysis of Variance (ANOVA). When the ANOVA indicated difference, we performed post-hoc tests, multiple pairwise comparisons between species to identify which species were different. To test if elevation affected seed mass, viability, and germination, we developed generalized linear models (GLM) for each species. The GLM is a flexible generalization of ordinary linear regression that allows for response variables that have error distribution models other than a normal distribution [Citation46]. We included individual as a random factor, as germination could differ significantly between individual trees [Citation28]. Individuals were nested within elevation. We explored the associations between seed mass, viability, and germination with linear regressions. For all models, the normality of the residuals and the absence of over-dispersion were verified. We used the software R [Citation47] for all statistical analyses and graphic representation.
Results
Seed masses were similar for the two species from the humid environments, P. australis and P. hieronymi (p = 0.45880) and around 0.57 and 0.43 times smaller as compared to P. tarapacana (p ˂ 0.001) and P. tomentella, respectively, from the arid environments (p ˂ 0.001; ). Along the elevation gradient, seed mass increased with elevation for P. hieronymi (F = 5.353, df = 23, p-value = 0.03) and there was no significant relation between seed mass and elevation for the other three species (; ). We found differences in seed mass between individuals for P. australis, P. tarapacana and P. tomentella and no significant differences for P. hieronymi.
Table 2. Species, means ± standard deviation and range (lowest and highest tree values in parenthesis) of mass per 100 seeds (g), viability (%), and germination (%) at 20°C/10°C (day/night) and 32°C/20°C (day/night) of four Polylepis species in northwest Argentina. Different letters within a column indicate significant differences in Post-hoc tests and results from Analysis of Variance (ANOVA) are indicated
Table 3. Results of GLM of four Polylepis species in northwest Argentina for seed viability, germination percentage at 20°C/10°C (day/night) and 32°C/20°C (day/night). Significance level: “**” < 0.01, “*”< 0.05
Figure 2. Seed mass (for 100 seeds) for P. australis, P. hieronymi, P. tarapacana and P. tomentella from different elevations. The blue line indicates a significant trend and grey shading the estimated 95% confidence interval
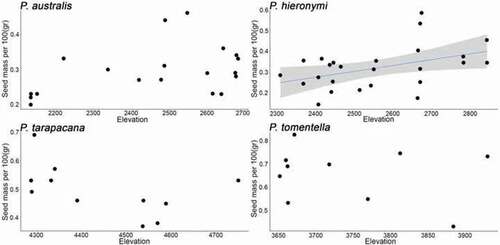
Seed viabilities were similar for P. australis, P. hieronymi and P. tarapacana, while we had no data for P. tomentella. Along the elevation gradient, seed viability did not significantly differ in any of the species studied. For P. hieronymi, the model detected differences between individuals, but not for the other two species ().
Germination percentages for P. australis and P. hieronymi were similar and around 3.7 times higher as compared to P. tarapacana and P. tomentella for the 20°C/10°C treatment (p = 0.001) and around 10 higher for the 32°C/20°C treatment (p = 0.05; ). Along the elevation gradient, seed germination response differed among species (, and ). For P. australis, germination decreased with elevation for both temperature treatments (p = 0.01 at 20°C/10°C and p = 0.05 at 32°C/20°C). For P. hieronymi we found no significant effects on both germination treatments. For P. tarapacana, germination decreased with elevation for one temperature treatment (p = 0.01 at 20°C/10°C) and germination differences were detected between individuals (p = 0.05 at 20°C/10°C), but no significant differences at 32°C/20°C. Finally, for P. tomentella, our models detected no significant differences. For P. tarapacana, the model detected differences between individuals, but not for the other three species ().
Figure 3. Germination at 20°/10° (day/night) as estimated under laboratory conditions in relation to elevation for four Polylepis species. Blue lines indicate significant trends, and grey shading the estimated 95% confidence intervals
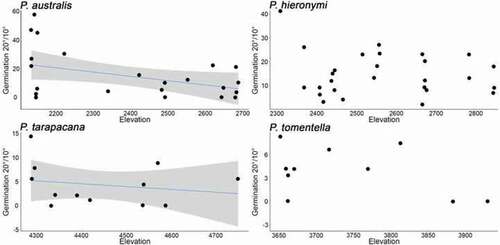
Figure 4. Germination at 32°/20° (day/night) as estimated under laboratory conditions in relation to elevation for four Polylepis species. The blue line indicates a significant trend and grey shading the estimated 95% confidence interval
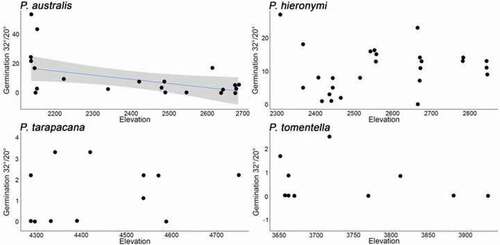
In all four species, a high percentage of non-germinated seeds were found to be without embryo. Based on linear regressions (), there was no effect of seed mass on germination in any of the four species. We detected associations between viability and germination values for P. australis: R2 = 0.17, F = 4.277, df = 22, p-value: 0.047 for treatment (20°C/10°C), R2 = 0.24, F = 6.606, df = 22, p-value = 0.017 for treatment (32°C/20°C) and P. hieronymi: R2 = 0.31, F = 1.22, df = 26, p-value = 0.00257 for treatment (20°C/10°C) and R2 = 0.20, F = 6.014, df = 26, p-value = 0.002152 for treatment (32°C/20°C). Only for P. tarapacana viability and germination were not significantly associated: F = 0.5857, df = 11, p-value = 0.4618 for treatment (20°C/10°C) and F = 0.002585, df = 11, p-value = 0.9604.
Discussion
In accordance with previous studies [Citation2,Citation28,Citation29,Citation48], we found low germination percentages for all species and presumably the low seed viability is the main reason for low germination percentages. The germination percentage for P. australis was similar to 20.3% and 23.0% ± 15.7 reported for the southern part of its distribution [Citation28,Citation43], and P. tarapacana germination percentages were in the range of 2–10% found in Bolivia [Citation49]. We could not find published results on P. hieronymi, but Hensen (unpublished) found 20% of the viable seeds in Peru, similar to our germination of 14%. Surprisingly, viability (TTC test) of P. tarapacana was high (23%) when compared to germination, this suggests the possibility of dormancy in a part of the seeds. In contrast, the average germination percentage for P. tomentella (3.8%) was lower than 7.4 ± 7.9% [Citation48] and 13.5–23.5% found in Bolivia for that species [Citation50] but similar to other species of dry environments; P. incana (2 to 4%) in a dry Andean region in Puno (Peru) [Citation51] and P. incarum (2%) at 3860 m asl in Bolivia [Citation49].
We found differences between germination percentages for species from contrasting environments but not within the same environment (). Environmental conditions experienced by plants during seed development and maturation have been reported to affect seed germination [Citation52,Citation53]. At our study sites at High-Andes and Puna, precipitation of wettest quarter was of 116 and 334 mm, respectively, in contrast to 570 and 635 mm at Yungas (values extracted from http://chelsa-climate.org [Citation54]). Therefore, low precipitation at Puna and High-Andes could be responsible for lower germination percentages of P. tomentella and P. tarapacana when compared to P. australis and P. hieronymi. Seed germination is influenced by water availability which was shown to be an important regulator in P. besseri [Citation55].
Contrary to our findings, seed mass is mentioned to vary between individuals of the same species [Citation56,Citation57]. We did not find a general pattern of seed mass variation with elevation within one species which was in line with a study analysing 29 Alpine species [Citation58]. Variation in seed mass could be an indirect measure of reproductive success, but in this study higher seed mass did not translate into higher germination, so seed mass could be responding to different environmental conditions. In mountainous regions, temperature and insolation varies with topography, even between very close localities [Citation59]. Between species, seed mass differed due to the responses to selective pressures of the environment [Citation58]. Species at higher elevation, P. tomentella followed by P. tarapacana, had higher seed mass than P. australis and P. hieronymi at lower elevations.
Furthermore, concerning the relationship between seed mass and germination, published results have been rather inconsistent [Citation60–62]. For example, most classic studies [Citation62–64] indicate a positive relationship between seed mass and germination, also found for P. tomentella in Bolivia and P. australis in central Argentina [Citation2,Citation43,Citation48]. Nevertheless, Bu et al. [Citation16] found a significant negative correlation in 570 alpine species. In many cases where clear patterns in the relationship between germination characteristics and seed mass were found, these patterns differed both between species and between populations [Citation65]. In our study, species with the heaviest seeds (P. tomentella and P. tarapacana) had the lowest germinations.
For P. hieronymi and P. taracapana, individual affected germination. For these species, we found both arboreal and individuals with a bushy growth. Most bushy individuals reproduce by ramets and form widespread patches. Localized clonal spread may interfere with sexual reproduction by reducing pollen transfer and mating between genets [Citation66], so it is possible that those bushy “islands” concentrate on asexual reproduction. A better understanding of trade-offs between sexual and clonal reproduction in Polylepis could be fundamental to understand patterns in germination.
Neither seed viability nor germination did peak at intermediate elevations. In the subtropical mountains from North-western Argentina tree growth at high elevations in the region may be seriously limited by water supply as annual precipitation decreases with elevation from 1500 mm at 1200–1500 m asl, to 200 mm above 4000 m asl [Citation25]. According to the reproduction hypothesis, limited reproduction could be limiting the upper treeline. Cierjacks et al. and Wesche et al. [Citation8,Citation67] reported decreasing sexual reproduction at the upper altitudinal limit for other Polylepis species. Our results adjust to this hypothesis, as for P. tarapacana and P. australis we found a decrease in germination percentage with elevation in the 20°C/10°C treatment. In the southern limit of its distribution, Marcora et al. [Citation2] found the opposite trend for P. australis, i.e. an increase for in-vitro germination with elevation, contrary to our study where germination decreased. As these two contrasting patterns are difficult to explain, it would be interesting to integrate results of germination experiments along the entire latitudinal gradient for P. australis.
Climate change is one of the main factors modifying high mountain vegetation and may result in species migration, adaptation, or extinction in the coming decades [Citation68,Citation69]. In particular, models predict 50% distribution reductions for P. tarapacana [Citation68,Citation69]. Instead, our germination data for P. australis and P. tarapacana (lower germination at higher elevations) suggest that higher future temperatures could have a positive impact. Nevertheless, they could be limited by water supply as aridness will increase. In particular, the differing tendencies with climate change for P. tarapacana between modelling [Citation68,Citation69] and germination experiments (in the present study) are probably due to the potential importance of future droughts which we did not evaluate in the present study but is taken into account in the modelling approach. Furthermore, germinations being only a small part of all the tree life cycle. We recommend more experimental studies on the effects of climate change on the genus and on all the important stages of the tree life cycle
In conclusion, we did not find evidence of negative global environmental change on the seed germination in our study species and region. The patterns in seed germination which we report with elevation and between individuals may be used to better direct seed collection for forest restoration purposes. To enhance germination, seeds could be collected at the lower elevations for P. australis and P. tarapacana, and individuals with seeds that have higher germination could be identified for P. tarapacana. Decreased germination may hamper reforestation measures which are being undertaken with Polylepis species along the Andes https://www.globalforestgeneration.org/our-work. Our results are only a first step to further studies on how ecological strategies are constructed by different Polylepis species that habitat contrasting ecological situations. Other plant functions that should be investigated [Citation11] are seed dispersal, persistence, germination timing, seedling, juvenile and adult establishment, growth and survival patterns.
Acknowledgments
CONICET partially funded the study through PIP#11220170100143C. We thank Joel Soto and his family and the community of Quebraleña for allowing us to collect seeds from their properties and receiving us. We thank field assistants for helping collecting seeds. We thank two anonymous reviewers and the editor who improved previous versions of the manuscript.
Disclosure statement
No potential conflict of interest was reported by the authors.
Additional information
Funding
References
- Francis AP, Currie DJ. A Globally Consistent Richness‐Climate Relationship for Angiosperms. Am Nat. 2003;161(4):523–536.
- Marcora P, Hensen I, Renison D, et al. The performance of Polylepis australis trees along their entire altitudinal range: implications of climate change for their conservation. Divers Distrib. 2008;14(4):630–636.
- Körner C. A re-assessment of high elevation treeline positions and their explanation. Oecologia. 1998;115(4):445–459.
- Körner C, Paulsen J. A world-wide study of high altitude treeline temperatures: study of high altitude treeline temperatures. J Biogeogr. 2004;31(5):713–732.
- Obeso JR. The costs of reproduction in plants. New Phytol. 2002;155(3):321–348.
- Chauhan BS, Johnson DE. Influence of Environmental Factors on Seed Germination and Seedling Emergence of Eclipta (Eclipta prostrata) in a Tropical Environment. Weed Sci. 2008;56(3):383–388.
- Baraloto C, Forget P-M, Goldberg DE. Seed mass, seedling size and neotropical tree seedling establishment. J Ecol. 2005;93(6):1156–1166.
- Cierjacks A, Rühr NK, Wesche K, et al. Effects of altitude and livestock on the regeneration of two tree line forming Polylepis species in Ecuador. Plant Ecol. 2007;194(2):207–221.
- Bader MY, Van Geloof I, Rietkerk M. High solar radiation hinders tree regeneration above the alpine treeline in northern Ecuador. Plant Ecol. 2007;191(1):33–45.
- Bruelheide H, Lieberum K. Experimental test for determining the causes of the altitudinal distribution of Meum athamanticum Jacq. In: the Harz Mountains. Flora. 2001. p. 227–241.
- Saatkamp A, Cochrane A, Commander L, et al. A research agenda for seed‐trait functional ecology. New Phytol. 2019;221(4):1764–1775.
- Hendrix SD, Nielsen E, Nielsen T, et al. Are seedlings from small seeds always inferior to seedlings from large seeds? Effects of seed biomass on seedling growth in Pastinaca sativa L. New Phytol. 1991;119(2):299–305.
- Leishman MR. Does the seed size/number trade-off model determine plant community structure? An assessment of the model mechanisms and their generality. Oikos. 2001;93(2):294–302.
- Cordazzo CV. Effect of seed mass on germination and growth in three dominant species in southern Brazilian coastal dunes. Braz J Biol. 2002;62(3):427–435.
- Vera FWM. Grazing ecology and forest history. Wallingford, Oxon; New York, NY: CABI Pub.; 2000.
- Bu H, Chen X, Xu X, et al. Seed mass and germination in an alpine meadow on the eastern Tsinghai–Tibet plateau. Plant Ecol. 2007;191(1):127–149.
- Moles AT, Westoby M. Seedling survival and seed size: a synthesis of the literature: seedling survival and seed size. J Ecol. 2004;92(3):372–383.
- Seiwa K, Kikuzawa K. Phenology of tree seedlings in relation to seed size. Can J Bot. 1991;69(3):532–538.
- Segovia-Salcedo MC, Domic A, Boza T, et al. Situación taxonómica de las especies del género Polylepis. Implicancias para los estudios ecológicos, la conservación y la restauración de sus bosques. Ecol Austral. 2018;28(1bis):188–201.
- Fjeldså J, Kessler M. Conservación de la biodiversidad d los bosques de Polylepis de las tierras altas de Bolivia. Una contribución al manejo sustentable de los Andes. San Cruz de la Sierra, Bolivia: FAN; 2004.
- The KM. “Polylepis problem”: where do we stand? Ecotropica. 2002;8:97–110.
- Sylvester SP. Relict high-Andean ecosystems challenge our concepts of naturalness and human impact. In: Sci Rep. 2017. p. 13.
- Kessler M. Bosques de Polylepis. In: Botánica Económica Los Andes Cent. 2006. p. 110–120.
- Hoch G, Körner C. Growth, demography and carbon relations of Polylepis trees at the world’s highest treeline. Funct Ecol. 2005;19(6):941–951.
- Morales MS, Villalba R, Grau HR, et al. Rainfall-controlled tree growth in high-elevation subtropical treelines. Ecology. 2004;85(11):3080–3089.
- Cuyckens GAE, Christie DA, Domic AI, et al. Climate change and the distribution and conservation of the world’s highest elevation woodlands in the South American Altiplano. Glob Planet Change. 2016;137:79–87.
- Renison D, Cuyckens GAE, Pacheco S, et al. Distribución y estado de conservación de las poblaciones de árboles y arbustos del género Polylepis (Rosaceae) en las montañas de Argentina. Ecol Austral. 2013;23(1):27–36.
- Renison D, Hensen I, Cingolani AM. Anthropogenic soil degradation affects seed viability in Polylepis australis mountain forests of central Argentina. For Ecol Manage. 2004;196(2–3):327–333.
- Vega CK, Villegas CG, Rocabado PA, et al. Biología reproductiva de tres especies de Polylepis (P. neglecta, P. incarum y P. pacensis), con énfasis en su comportamiento germinativo. Ecol Austral. 2018;28(1bis):310–324.
- Seltmann P, Renison D, Cocucci A, et al. Fragment size, pollination efficiency and reproductive success in natural populations of wind-pollinated Polylepis australis (Rosaceae) trees. Flora - Morphol Distrib Funct Ecol Plants. 2007;202(7):547–554.
- Domic AI, Capriles JM. Allometry and effects of extreme elevation on growth velocity of the Andean tree Polylepis tarapacana Philippi (Rosaceae). Plant Ecol. 2009;205(2):223–234.
- Morales LV, Fuentealba B, Sevillano CS, et al. Oportunidades para acercar la ciencia a la práctica de la restauración de bosques y arbustales de Polylepis. Ecol Austral. 2018;28(1bis):291–300.
- Rahbek C, Borregaard MK, Colwell RK, et al. Humboldt’s enigma: what causes global patterns of mountain biodiversity? Science. 2019;365(6458):1108–1113.
- Simpson BB. A revision of the Genus Polylepis (Rosaceae, Sanguisorbaeae). Smithson Contrib Bot. 1979;43(43):1–62.
- Macek P, Macková J, De Bello F. Morphological and ecophysiological traits shaping altitudinal distribution of three Polylepis treeline species in the dry tropical Andes. Acta Oecologica. 2009;35(6):778–785.
- Bianchi AR, Yáñez CE, Acuña LR Base de datos mensuales de precipitaciones del noroeste argentino [Internet]. Secretaría de agricultura, ganadería pesca y alimentación. Instituto Nacional de Tecnología Agropecuaria; 2005 [cited 2020 Apr 22]. Available from: http://appweb.inta.gov.ar/w3/prorenoa/zonadescarga/Precip_NOA/Precipitaciones_del_noa.pdf
- Cabrera AL, Willink A. Biogeografía de América Latina. Washington DC: Secretaría General de la Organización de los Estados Americanos; 1973.
- Burkart R, Bárbaro NO, Sánchez RO, et al. Eco-regiones de la Argentina. Buenos Aires Argentina: Administración de Parques Nacionales, PRODIA; 1999.
- Bianchi A, Yáñez J. Las precipitaciones en el noroeste argentino. Salta: Estación Experimental Regional Agropecuaria, INTA; 1992.
- Richter M. Tropical mountain forests - distribution and general featurres. In: Sr G, Homeier J, Gansert D, editors. Trop Mt For - Patterns Process Biodivers Hotspot. Göttingen Centre for Biodiversity and Ecology; 2008. p. 7–24.
- Dinerstein E, Olson DM, Graham DJ, et al. Una evaluación del estado de conservación de las eco-regiones terrestres de América Latina y el Caribe. Washington, DC: Banco Mundial; 1995.
- Davis SD, Heywood VH, Hamilton AC, et al. Eds.. Centres of plant diversity: a guide and strategy for their conservation. Cambridge, U.K: World Wide Fund for Nature (WWF) and ICUN - World Conservation Union; 1994.
- Seltmann P, Leyer I, Renison D, et al. Variation of seed mass and its effects on germination in Polylepis australis: implications for seed collection. New For. 2007;33(2):171–181.
- Baskin CC, Baskin JM. Seeds: ecology, biogeography, and evolution of dormancy and germination. Second ed. San Diego, CA: Elsevier/AP; 2014.
- Gareca EE, Martinez YY, Solís C, et al. Efectos de los árboles exóticos y del ambiente materno sobre la producción de semillas, la germinación y el crecimiento inicial de Polylepis subtusalbida (Rosaceae) en el Parque Nacional Tunari, Bolivia. Ecol Austral. 2018;28(1bis):262–277.
- Zuur AF, Ieno EN, Walker NJ, et al. Mixed effects models and extensions in ecology with R. New York: Springer; 2009.
- R Development Core Team. The R Project for Statistical Computing [Internet]. Viena, Austria; 2012 [cited 2013 Jan 4]. Available from: http://www.r-project.org./
- Domic AI, Capriles JM, Camilo GR. Evaluating the fitness effects of seed size and maternal tree size on Polylepis tomentella (Rosaceae) seed germination and seedling performance. J Trop Ecol. 2020;36(3):115–122.
- Vega KC, Quezada PJ, Rocabado KP, et al. Assessment of Seed Quality and Germination Response in the Species of the Genus Polylepis. Carlos Jimenez-Lopez J, editor. Seed Dormancy Germination [Internet]. IntechOpen. 2020. cited 2020 Mar 27. Available from. https://www.intechopen.com/books/seed-dormancy-and-germination/assessment-of-seed-quality-and-germination-response-in-the-species-of-the-genus-em-polylepis-em-
- Sánchez JA Determinación de la longevidad de semillas de las especies forestales nativas aliso (Alnus acuminata H.B.K.) y Quewiña (Polylepis tomentella Weddell) [de grado]. [Potosí, Bolivia]: Universidad Autónoma “Tomás Frías”, Facultad de Ciencias Agrícolas y pecuarias, Carrera de Agronomía; 2000.
- Pretell Chiclote J, Ocaña Vidal D, Jon Jap R, et al. Apuntes sobre algunas especies forestales nativas de la Sierra peruana. Lima, Perú: Proyecto FAO/Holanda/INFOR; 1985. p. 86.
- Donohue K, Heschel MS, Butler CM, et al. Diversification of phytochrome contributions to germination as a function of seed-maturation environment. New Phytol. 2007;177:367–379.
- Gorecki MJ, Long RL, Flematti GR, et al. Parental environment changes the dormancy state and karrikinolide response of Brassica tournefortii seeds. Ann Bot. 2012;109(7):1369–1378.
- Karger DN, Conrad O, Böhner J, et al. Climatologies at high resolution for the earth’s land surface areas. Sci Data. 2017;4:170122.
- Gareca EE, Vandelook F, Fernández M, et al. Seed germination, hydrothermal time models and the effects of global warming on a threatened high Andean tree species. Seed Sci Res. 2012;22(4):287–298.
- Völler E, Auge H, Prati D, et al. Geographical and land-use effects on seed-mass variation in common grassland plants. Basic Appl Ecol. 2012;13(5):395–404.
- Zhang ST, Zhen DG, Chen JK. Seed size in relation to phylogeny, growth form and longevity in a subalpine meadow on the east of the Tibetan plateau. Folia Geobot. 2004;39(2):129–142.
- Pluess AR, Schütz W, Stöcklin J. Seed weight increases with altitude in the Swiss Alps between related species but not among populations of individual species. Oecologia. 2005;144(1):55–61.
- Valeix M, Loveridge AJ, Davidson Z, et al. How key habitat features influence large terrestrial carnivore movements: waterholes and African lions in a semi-arid savanna of north-western Zimbabwe. Landsc Ecol. 2009;25(3):337–351.
- Gomez JM. Bigger is not always better: conflicting selective pressures on seed size in Quercus ilex. Evolution. 2004;58(58):71–80.
- Stamp NE. Production and effect of seed size in a grassland annual (Erocium brachycarpum, Geraniaceae). Am J Bot. 1990;77(7):874–882.
- Vera ML. Effects of altitude and seed size on germination and seedling survival of heathland plants in north Spain. Plant Ecol. 1997;133(1):101–106.
- Weis IM. The effects of propagule size on germination and seedling growth in Mirabilis hirsuta. Can J Bot. 1982;60(10):1868–1874.
- Wulff RD. Seed Size Variation in Desmodium Paniculatum: III. Effects on Reproductive Yield and Competitive Ability. J Ecol. 1986;74(1):115.
- Milberg P, Andersson L, Elfverson C, et al. Germination characteristics of seeds differing in mass. Seed Sci Res. 1996;6(4):191–198.
- Handel SN. The Intrusion of Clonal Growth Patterns on Plant Breeding Systems. Am Nat. 1985;125(3):367–384.
- Wesche K, Cierjacks A, Assefa Y, et al. Recruitment of trees at tropical alpine treelines: erica in Africa versus Polylepis in South America. Plant Ecol Divers. 2008;1(1):35–46.
- Hoegh-Guldberg O, Hughes L, McIntyre S, et al. ECOLOGY: assisted Colonization and Rapid Climate Change. Science. 2008;321(5887):345–346.
- Ecological PC. Evolutionary Responses to Recent Climate Change. Annu Rev Ecol Evol Syst. 2006;37(1):637–669.