ABSTRACT
Mountain ecosystems are characterized by steep environmental gradients. Species with broad elevation distribution are exposed to contrasting climatic conditions along their gradients. In response to those changes, species might develop ecotypes adapted to the local climate. Early regeneration is the most critical stage for plant populations and, therefore, withstands strong selection pressures. Previous studies showed a unimodal pattern of intraspecific variability in fitness components among Polylepis australis populations along their elevation gradient in the mountains of central Argentina. Whether this variability is the result of local populations’ adjustments to their respective site remains unknown. We tested the hypothesis that P. australis populations from different elevations would be locally adapted to their local environments, where they would perform better than populations from other elevations (origins). We applied an experimental approach by establishing common gardens at three contrasting elevations. Seeds and saplings of P. australis populations from the low, mid- and high elevation belts of the species distribution gradient (i.e. three origins) were reciprocally sown and transplanted in three elevation sites (low, mid- and high). Seed germination success, sapling growth and mortality were monitored for three years. Our main results show that the origin of populations influenced germination success and sapling growth, whereas sapling mortality was marginally affected. Populations of both elevation extremes seem to be more adjusted to local conditions than the population from the intermediate elevation, probably due to the harsher environmental conditions for plant regeneration typical of the lower and upper elevation belts of semiarid mountains. Finally, our results strongly suggest that temperature rise due to climate change in this mountain area might have negative effects on the regeneration of P. australis populations.
Introduction
Mountain ecosystems are characterized by steep environmental gradients. Shifts in elevation are associated with variations in temperature, atmospheric pressure, UV radiation, length of the growing season and other factors at relatively small spatial scales [Citation1]. Species distributed along the elevation gradients of mountain ecosystems are consequently exposed to contrasting climatic conditions. In response to those changes, and to express the best possible performance, species might develop ecotypes adapted to the local climate [Citation2–5]. If ecotype differentiation occurs, populations from different elevations are expected to exhibit distinctive characteristics as a result of the adjustment to their local conditions [Citation6–8].
According to Kaweki and Ebert [Citation9], the assessment of local adaptation in populations along the elevation gradient may reveal three alternative and extreme responses: i) all origins belong to a single generalist population showing a similar degree of adaptation to all sites (there are no differences in response variables among sites); ii) all origins belong to a single specialist population optimally adapted to one elevation and poorly adapted to other belts (there are no differences in response variables among origins); iii) all origins are a set of specialist populations, with each one performing best at their local elevation. Local adaptation requires an outcome close to iii [Citation9].
Early stages of the life cycle are the most critical for plant populations [Citation10]. In particular, in semi-arid mountains, seedlings face different environmental constraints at each elevation belt. Plants occurring at the lowest extreme undergo harsh conditions for seedling emergence and survival [Citation11], mainly water deficit [Citation12–14] and high temperatures [Citation15,Citation16]. On the opposite extreme, at the highest elevation, seedlings are exposed to low temperatures and a reduced length of the growing season [Citation3,Citation17], whereas at mid-elevations seedlings might face intermediate levels of the constraints found at both extremes. Therefore, populations along the entire elevation gradient are expected to be exposed to different selection pressures that might promote local adaptations when gene flow is reduced and/or overcome by selection pressures [Citation5,Citation6,Citation18,Citation19].
Polylepis australis is dominant in highland woodlands of central Argentina mountains [Citation20,Citation21], where it is distributed from 900 to 2700 m a.s.l [Citation22]. Previous studies found variation in the species regeneration traits, such as seedling growth and survival, along its elevation range [Citation22–25]. In particular, these studies suggest optimal conditions for P. australis at mid-elevations, since its performance decreases towards both elevation extremes in response to the constraining factors typical of semi-arid mountain ecosystems [Citation22–26]. The differences in plant performance between elevations described in those field studies could be a consequence of phenotypic plasticity, considering that populations appear to be connected by effective long-distance pollination [Citation27,Citation28] and that they are not genetically structured throughout elevation gradients [Citation29]. However, common garden experiments under greenhouse conditions showed that populations from different elevations inherit morphological, physiological and metabolic adjustments [Citation30,Citation31]. In addition, it is known that populations at marginal habitats might become locally adapted despite gene flow [Citation19]. Whether those differences in features among origins improve their performance under local conditions remains to be tested. In the case of P. australis, the phenological delay in flowering towards higher elevations [pers. Obs.], anemochorous short-distance dispersion [Citation32] and different levels of ploidy [Citation33] might contribute to the isolation of populations and the development of local adaptations. Thus, the aim of our study was to evaluate if populations of P. australis are adapted to local environmental conditions along its elevation range of distribution. We hypothesize that along the elevation gradient of species distribution, changes in environmental conditions would promote local adaptation. Consequently, we expect that at each elevation the local population expresses higher performance than populations from other elevations. To test this hypothesis, we established common gardens at different elevations using reciprocal sowing and transplant experiments. Specifically, we assessed how seeds and saplings from low, mid- and high elevation origins varied in germination success, growth and survival when translocated to three sites corresponding to the low, intermediate, and high elevations of the species distribution range in the mountains of central Argentina.
This study attempts to improve our understanding of local adaptations, particularly contributing to the scarce knowledge about woody species in the temperate portion of the Southern Hemisphere [Citation34]. In addition, this knowledge is critical for improving species management under current global change scenarios [Citation35]. For instance, the level of local adaptation in populations distributed at the extremes of a species elevation gradient may reflect the probability of distributional upward shifts in the future [Citation35–37].
Methods
Study species
Polylepis australis BITT. (Rosaceae) is a perennial woody species endemic to the mountains of central and north western Argentina, where it dominates the treeline [Citation38]. Mature individuals annually produce flowers in which self-pollination is prevented by protogyny. Pollen has high viability and longevity, and is effectively long-distance dispersed by wind [Citation27]. Accordingly, populations in the mountains of central Argentina appear to be connected by effective long-distance pollination [Citation28]. Fecundity increases with distance from the pollen source [Citation27,Citation39]. Fruits are single-seeded nutlets wind-dispersed over short distances [Citation32]. Fruit ripening timing varies along the elevation gradient, peaking in December at the lowest elevation, and in April at the highest elevation (i.e. early summer and autumn, respectively).
Study area
The study was conducted in the Sierras Grandes Mountains of central Argentina (). The system comprises mountains of middle elevation (the Champaquí peak reaches 2790 m a.s.l.) that rise from the Pampas plain. Mean annual temperature at the lower elevation is 15.7 °C, dropping to 7.4 °C at the summit [Citation22]. There is no frost-free period above 1800 m a.s.l. Mean annual precipitation varies between 750 and 970 mm along the elevation gradient, with most rainfall being concentrated in the warmer months (from October to April [Citation40]). Accordingly, there is a significant decrease in soil temperature and a significant increase in soil moisture towards the upper belt of the elevation gradient [Citation26]. At present, vegetation consists of a mosaic of tussock grasslands, grazing lawns, outcrop communities, highland woodlands, and eroded areas with exposed rock surfaces [Citation41]. Sites selected to perform the experiment corresponded to tussock grasslands and short grazing lawns ()).
Figure 1. Map of the study area. The location of the mountains of central Argentina in South America is shown in the upper left. The elevation belts are of the mountains are shown in grey scales. Circles represent the three elevation sites (at 1600, 2200 and 2400 m a.s.l.). The most important city in the region is indicated
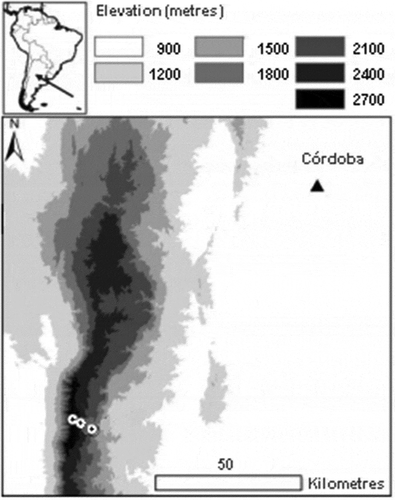
Experimental design
A full site × origin design was used following the classical reciprocal transplant experiments [Citation9]. In our study, the sites corresponded to three elevations with contrasting climatic and biotic conditions [Citation26,Citation42]. The sites were located at lower, mid- and upper elevation belts in the Sierras Grandes Mountains (1600, 2200 and 2400 m a.s.l., respectively) near Linderos road (S32° 53ʹ W64° 3ʹ). Three origins were considered, represented by P. australis populations established at the lower (~1500 m a.s.l.) and upper (~2700 m a.s.l.) ends of the species elevation gradient, and at the intermediate elevation of this range (~2100 m a.s.l.). Seeds collected from each origin were used in the sowing experiment in the field as well as to produce saplings in a greenhouse to be used in the reciprocal transplant experiment (see below for details of both experiments). These experiments consisted of exposing seeds and saplings from each of the three origins to each of the three site-conditions (). At each site, two 15 x 15-m common gardens were established and protected from livestock browsing and trampling with a wire fence.
Figure 3. Schematic representation of the reciprocal sowing and transplanting experiments. Seeds were collected from established populations at the low, mid- and high elevation belts of Polylepis australis distribution range in the mountains of central Argentina (i.e. low, mid- and high origins). Saplings were produced under greenhouse conditions. Seeds and saplings from all origins were established at three elevation sites (low, mid- and high sites) within two common gardens protected from livestock. At each site, eight sowing plots were distributed in both common gardens. Each sowing plot corresponding to one mother per origin had 500 seeds. Nearly 17 saplings from a variable number of mother plants per origin were established in each common garden
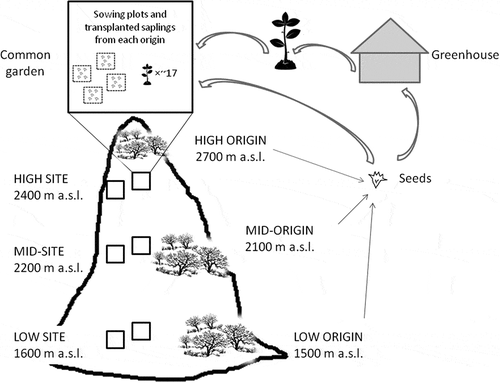
Seeds from 20 mother plants of each origin were collected in separate bags from January to March 2015. Seeds were stored in paper bags and kept in the lab at room temperature until the beginning of the experiments. Seedling production in the greenhouse started in July 2015 (winter). Due to the low seed viability of some trees, the final number of mother plants that yielded enough saplings in the greenhouse was eight for the low and the intermediate origins, and 10 for the high origin. Seeds from the same mother plants were then used for the sowing experiment.
One month before starting the field experiments (November 2015), grasses and herbs of all common gardens were cut to avoid initial differences in vegetation structure. By cutting the vegetation we also expected to mimic the herbaceous sites with low grazing pressure, where the highest P. australis recruitment is recorded () [Citation25,Citation32,Citation43]. Regeneration of this species within woodlands is severely reduced by litter, ferns and moss, and canopy cover [Citation43–46]. Therefore, herbaceous areas are the sites where the species spread from neighbouring woodlands at each elevation.
Reciprocal sowings
In June 2016 (autumn), the time of the natural seed dispersion, seeds of each origin were sown in 50 x 50-cm plots. At each site, 8 plots per origin were distributed in 2 common gardens (4 seed plots x 2 common gardens x 3 sites x 3 origins = 72 plots of seeds, see ). These plots were previously weeded by hand and delimited with a 1 cm-mesh plastic green net staked to the ground ()). After that, 500 seeds of a single mother plant were sown in each plot. Seed germination success was assessed as seedling emergence for three years (i.e. monthly during the first six months, and every six months thereafter). Since viability values among origins can differ in one order of magnitude [Citation22,Citation30], the percentage of emergence per plot was estimated as the total number of new emerging seedlings during the three years divided by the total number of viable seeds. The number of viable seeds per origin was estimated as the number of emerged seedlings in the site where the maximum emergence was recorded.
Reciprocal transplants
Seedlings were produced in a greenhouse and transplanted to the field common gardens when they were four months old (December 2015). A total of 35 saplings of each origin were randomly distributed within both common gardens in each site (35 saplings × 3 origins × 3 sites = 315 saplings; ). Inside each common garden, the number of saplings per mother plant ranged between 1 and 8. Each sapling was identified with a numbered metal stake ()). Sapling height was measured when transplanted and three years later, at the end of the experiment. Growth per sapling was then calculated as the difference between these final and initial height measurements. Accumulated sapling mortality was recorded at the end of the experiment (three years after transplanting).
Data analysis
Seed germination success, sapling growth and mortality accumulated over the three years were analyzed using generalized linear mixed models (GLMMs) including origin, site and their interaction as fixed factors. Local adaptations imply an interaction between origin and site [Citation9]. Common garden and mother plant were included as crossed random factors.
The contribution of mother plants to the variance of each response variable was evaluated by comparing the models with and without this factor using Akaike information criteria (AIC) and by performing likelihood ratio tests. If the inclusion of this random factor is significant, it indicates that seeds and saplings from the same mother plant are more similar between them (within the family) than to those from other mothers, suggesting a genetic component [Citation47,Citation48].
The Models and likelihood-ratio test were performed using R-software v.1.1.463 [Citation49]. Log10 of seed germination and sapling growth were modelled as normal variables. Assumptions of homogeneity of variance and normal distribution were examined. Log10 of seed germination was analysed using lmer function. Sapling growth was analysed using lme function. For mortality, the live/dead condition of each individual was analysed with GLMM for binomial data using the glmer function (link function logit), and overdispersion was assessed. A contrast test was used to compare local versus foreign origins within each elevation site to test the prediction that local populations outperform foreign populations. Contrasts were performed with Infostat software v.2020 [Citation50].
Results
Percentage of seed germination success under field conditions was low for all origins at all sites (). The effects of origin and site interactions on seed germination were significant (). At the mid-elevation site, the local origin had higher seed germination success than the low origin (), P = 0.0098). In turn, at the low elevation site, the local origin showed higher germination success than the high origin (P = 0.0501). The remaining contrasts were statistically non-significant (all P > 0.05). Moreover, the three origins showed different trends in germination responses of each population along the elevation gradient. The low origin showed a reduction of seed germination with increasing elevation. In contrast, both the mid- and high origins exhibited a reduction towards the low site ()). The random effects of mother plants were significant (). The variance was higher among different mother plants than within sowing plots of each mother plant (45 and 30%, respectively).
Table 1. Test statistics of the GLMMs performed to assess the effects of site, origin and their interaction on seedling emergence, sapling growth and sapling mortality of three Polylepis australis populations subjected to the reciprocal sowing and transplant experiments. Models with and without mother plant as random factor were compared using the AIC and the likelihood ratio test (LRT), for which P value is provided
Figure 4. Performance of three different origins of Polylepis australis at three sites along an elevation gradient. (a) Seedling emergence, (b) sapling height growth, and (c) sapling mortality. Seeds and saplings were transferred to each site from low (black dots), mid- (grey triangles) and high (light grey squares) origins. Values are mean ± standard error. Different letters indicate significant differences among the local origin and the two foreign populations at each site according to contrasts (*P ≤ 0.05; ** P < 0.001). Letters are not included in growth and mortality figures because there were no significant differences per site (all P > 0.05; see text for details)
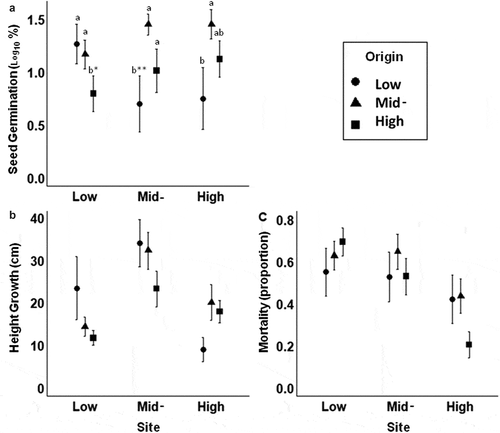
Growth of transplanted saplings was also significantly affected by the interaction between origin and site (). At each site, local origin showed high values of sapling growth, but the trends of differentiation from foreign origins were not statistically significant (all P > 0.05). That is, local saplings at the low elevation site showed a non-significant trend of higher growth than foreign origins, particularly compared to saplings from the high origin. In turn, at the high elevation site, growth of the local saplings was higher than that of the low origin. At mid-elevations, growth of local saplings was higher than that of samplings from high elevation origin. Growth response of each population along the elevation gradient also showed different trends. Growth of saplings from the low origin was lower in the high-elevation site, whereas growth of saplings from high and intermediate origins was lowest in the low-elevation site. Growth of all origins was highest at the mid-elevation site ()), reaching more than twice the size of the same origins transplanted to the low or high elevation sites. The random effect of mother plants on sapling growth was not significant ().
Sapling mortality differed among elevation sites, while the effect of the interaction between site and origin was marginally significant (). At low and high-elevation sites, local origins showed a non-significant trend of lower mortality than foreign origins. All origins showed the lowest mortality values in the high-elevation site ()), but the mortality of the local origin was twice as low as that of the foreign origins. The random effect of mother plants on sapling mortality was not significant ().
Discussion
Unlike predicted, we did not find consistent evidence of local adaptation considering Polylepis australis full elevation range. However, when we considered the marginal habitats, we found support for local adaptation, since populations from the low and high elevation belts were better adapted to their local environments. In agreement with previous studies, our results also show that the environmental conditions at different elevations have a strong influence on the regeneration of this species.
Does ecotype differentiation along the elevation gradient improve Polylepis australis performance under local conditions?
The hypothesis of local adaptation predicts higher performance of local populations than foreign ones at each elevation site [Citation3,Citation7,Citation9]. Our results, considering three sites and origins, did not follow that expected trend. However, we observed a consistent pattern of higher performance under local conditions when considering the populations and environments of the upper and the lower ends of the species distribution. In these marginal habitats, our findings supported local adaptation in terms of germination success, and sapling growth and survival. Similarly, studies on other species distributed along environmental gradients also found that local adaptations were more frequent and consistent at the extreme of species distribution ranges, where selective pressures are stronger [Citation3,Citation6,Citation7,Citation19] than at intermediate sites [Citation9,Citation51]. Consistently, previous works showed that at mid-elevation sites, P. australis populations grow under less restrictive conditions [Citation22,Citation23]. Additionally, these populations would be connected by genetic flow with those of both elevation extremes [Citation9,Citation52,Citation53]. Consequently, the intermediate origin seems to be an intermediate population with similar or lower performance than the local origins at both elevation extremes.
In addition to population origin, maternal effects were also relevant for seed germination, as found for other species [Citation7,Citation54–56]. Mother plant explained an important part of the variance of seed germination success, suggesting some evolutionary potential to develop local adaptations [Citation47,Citation48]. Contrarily, it had no effect on sapling growth and survival. It is possible that maternal effects do not persist in post-germination stages [Citation3,Citation54]. Alternatively, production of saplings under greenhouse conditions, which may have reduced the initial influence of mother plants, may explain the lack of maternal effects. Finally, the influence of the epigenetic process cannot be ruled out.
Disentangling the effects of origin and elevation site on each performance variable
Seed germination must be adjusted to local conditions to occur under optimal climatic conditions for seedlings survival [Citation56–60]. In our study, at the low site the local origin population had higher seed germination than the high origin one ()), suggesting an adaptation to local environmental conditions. Our results are in line with those reported by Cáceres et al. [Citation61], who exposed P. australis seeds from different elevations to drought and heat stress, and found that seeds from intermediate and high origins were less resistant than seeds from low origin. In agreement with those findings, seed germination of the low origin of our study seems to be less adapted to environmental conditions at the mid- and high elevations, where frost events occur throughout the year [Citation62]. The observed adjustments of the low origin seedlings to local conditions are similar to those found in other species occurring in semi-arid mountain ecosystems, where plants should respond to high temperatures and water shortage, the main climatic restrictions for early regeneration at low elevations [Citation7,Citation16,Citation54]. Regarding the population from the mid-elevation, although it maximizes germination success in local environments, it also behaves like a generalist population, with similar responses across the elevation range.
The unimodal pattern of sapling growth in response to elevation, observed for populations of the three elevation origins, is in line with previous studies in P. australis and likely a consequence of the hard environmental conditions present at both ends of species distribution range [Citation22,Citation23]. Accordingly, trends indicative of local adaptation in terms of growth were mainly observed in both elevation ends. At low elevations, the local population seems to outperform the other populations in terms of growth. This could be achieved by higher heat tolerance under drought conditions, as described for alpine species growing in environments similar to our low elevation site [Citation63]. In turn, at the upper elevation, the higher growth of local saplings than of those of low elevation origin suggests local adaptations to low temperatures (see below). The lower peak in growth observed for saplings of high elevation origin under the less severe environment of the mid-elevation highlights the strength of this population’s adjustments. Accordingly, previous studies showed that the high-origin population had lower growth rates than other origins under optimal irrigation, temperature and light conditions in a greenhouse [Citation30]. Moreover, in other species, populations from high elevation were found to have shorter active growth periods due to a strong photoperiod control of growth cessation [Citation3], which may also explain the observed pattern for the high origin population.
We found a consistent increase in mortality of sapling of all origins towards the low elevation. Similarly, previous studies on other woody species showed that the main regulator of seedling mortality along elevation gradients is water stress, which can even reduce survival to almost zero [Citation7]. Indeed, dead saplings with all their leaves dry were found at the low elevation site (pers. obs.). Additionally, towards low elevations, an increase in the abundance of herbivores is expected due to higher temperatures and plant productivity [Citation64–67]. In this regard, some saplings disappeared at the low elevation site between sampling dates, suggesting consumption by small animals that were not excluded by fences (e.g. insects, rodents, etc). At the opposite extreme of the species distribution, in the upper elevation, sapling mortality was lowest, particularly for the local origin. The higher resistance of local populations could be a consequence of metabolic adjustments in response to cold stress. Frost resistance involves molecular and physiological processes that protect plant tissues against ice formation and maintain the photosynthetic apparatus [Citation68]. Increased resistance of photosynthetic function to cold is a common feature in evergreen species under cold environments [Citation51]. Thus, plants from the high origin might show a trade-off between growth and freezing resistance, and prioritize security rather than vigour [Citation3]. In fact, previous studies assessing the same elevation gradient found that P. australis populations from high elevation exhibit more pronounced metabolic cold stress responses, with a partially broader reliance on cold stress intensity than populations from low elevations [Citation31]. Given these results, it would be important to experimentally separate the influence of climatic factors and herbivory by small animals on the regeneration of this species and on the development of ecotypes along the entire elevation gradient of the distribution range. All in all, our results suggest that P. australis survival would be more threatened by drought, heat and herbivory than by cold, at least in the studied gradient. Actually, the species reaches higher elevations (~3800 m a.s.l.) in its northern extreme of distribution, where it is exposed to lower temperatures.
In summary, our results support the hypothesis of P. australis local adaptations only for the low and high extreme elevations of the species distribution range. Local adaptations might help to cope with, but not compensate, environmental restrictions on performance at both elevation extremes of the species distribution. Additionally, our results show that the lowest site might present the most restrictive conditions for the regeneration of P. australis, and suggest that if these site conditions spread upwards (e.g. as a consequence of global warming), the areas where the species shows highest growth (i.e. mid-elevation) and lower mortality (i.e. high elevation) will likely be reduced. However, our field experiment does not discriminate among environmental stressors, and understanding morpho-physiological adjustments in response to the main environmental constraints is needed to improve inferences regarding climatic change scenarios.
Climate change consequences for Polylepis australis conservation
The frequency and intensity of major extreme events like droughts are increasing as a consequence of global climatic change [Citation69], with a significant influence on forest regeneration in many regions of the world [Citation6,Citation37,Citation67,Citation70]. In the mountains of central Argentina, water shortage has been increasing in the last few years [Citation71]. In these mountains, P. australis seeds and saplings from the lower elevation seem to exhibit a better response to drought and high temperatures than those coming from the mid- and high elevation belts. Therefore, these populations on the low margins of the distribution range could be disproportionately important for the long-term conservation of genetic diversity and evolutionary potential of the species [Citation6]. Our results suggest that in the face of climate change, the expected increase of temperatures and drought towards high elevations might have significant and negative impacts on P. australis regeneration all across its elevation range. Globally, our work highlights the importance of studying local adaptation to improve the success of ecosystem restoration programs, mainly for temperate southern hemisphere species, for which this mechanism is mostly unknown [Citation34,Citation72].
Disclosure of potential conflicts of interest
No potential conflict of interest was reported by the author(s).
Geolocation information
The study was conducted near the Linderos road (S32° 53ʹ W64° 3ʹ), in Sierras Grandes, Córdoba, Argentina.
Acknowledgments
This work was supported by the German Research Foundation (Deuthsche Forschungsgemeinchaft Grant/ Award Number: HE3041/21-1), and SECyT (05/1781 and 30720150101020CB). Special thanks to colleagues and students that collaborated during sapling transplants and field measurements: MR Rosetti, N Kushmanish, LD Gorné, MA Giorgis, AI País-Bosch, AM Rubini-Pisano, J Meneghello, G González, D Simian. Additionally, we are thankful to owners that permitted us to establish common gardens in private properties. AG got a research assistant position at FCEFyN (UNC) to participate in this project (Resolution 330-H.C.D.-2018). We also wish to thank two anonymous reviewers for useful comments on the manuscript and to Jorgelina Brasca who assisted with the English editing.
Additional information
Funding
References
- Körner C. Alpine plant life. Alpine climate. Springer, Berlin, Heidelberg, 1999:21–30. https://link.springer.com/book/10.1007/978-3-642-98018-3#about
- Hampe A, Bairlein F. Modified dispersal-related traits in disjunct populations of bird-dispersed Frangula alnus (Rhamnaceae): a result of its quaternary distribution shifts? Ecography. 2000;23(5):603–613.
- Körner C. Alpine treelines: functional ecology of the global high elevation tree limits. Springer Science & Business Media; Basel, 2012. p. 233.
- Valladares F, Matesanz S, Guilhaumon F, et al. The effects of phenotypic plasticity and local adaptation on forecasts of species range shifts under climate change. Ecol Lett. 2014;17(11):1351–1364.
- Vitasse Y, Hoch G, Randin CF, et al. Elevational adaptation and plasticity in seedling phenology of temperate deciduous tree species. Oecologia. 2013;171(3):663–678.
- Hampe A, Petit RJ. Conserving biodiversity under climate change: the rear edge matters. Ecol Lett. 2005;8(5):461–467.
- Giménez-Benavides L, Escudero A, Iriondo JM. Local adaptation enhances seedling recruitment along an altitudinal gradient in a high mountain Mediterranean plant. Ann Bot. 2007;99(4):723–734.
- Premoli AC, Brewer CA. Environmental v. genetically driven variation in ecophysiological traits of Nothofagus pumilio from contrasting elevations. Austral J Bot. 2007;55(6):585–591.
- Kawecki TJ, Ebert D. Conceptual issues in local adaptation. Ecol Lett. 2004;7(12):1225–1241.
- Kitajima K, Fenner M Seeds: the ecology of regeneration in plant communities. Cabi; 2000. Chapter 2, Ecology of seedling regeneration; p. 331–359.
- Arrieta S, Suárez F. Marginal holly (Ilex aquifolium L.) populations in Mediterranean central Spain are constrained by a low-seedling recruitment. Flora. 2006;201(2):152–160.
- Pigott CD, Pigott S. Water as a determinant of the distribution of trees at the boundary of the Mediterranean zone. J Ecol. 1993;81(3):557–566.
- Wang Q, Zhao C, Gao C, et al. Effects of environmental variables on seedling-sapling distribution of Qinghai spruce (Picea crassifolia) along altitudinal gradients. For Ecol Manage. 2017;384:54–64.
- Castro J, Zamora R, Hódar JA, et al. Seedling establishment of a boreal tree species (Pinus sylvestris) at its southernmost distribution limit: consequences of being in a marginal Mediterranean site. J Ecol. 2004;92(2):266–277.
- Peñuelas J, Boada M. A global change induced biome shift in the Montseny mountains (NE Spain). Glob Chang Biol. 2003;9(2):131–140.
- Broadhead JS, Ong CK, Black CR. Tree phenology and water availability in semi-arid agroforestry systems. For Ecol Manag. 2003;180(1–3):61–73.
- Rehm EM, Feeley KJ. Freezing temperatures as a limit to forest recruitment above tropical Andean treelines. Ecology. 2015;96(7):1856–1865.
- Premoli AC. Isozyme polymorphisms provide evidence of clinal variation with elevation in Nothofagus pumilio. J Hered. 2003;94(3):218–226.
- Kawecki TJ. Adaptation to marginal habitats. Annu Rev Ecol Evol Syst. 2008;39(1):321–342.
- Cingolani AM, Renison D, Tecco PA, et al. Predicting cover types in a mountain range with long evolutionary grazing history: a GIS approach. J Biogeogr. 2008;35(3):538–551.
- Renison D, Chartier MP, Menghi M, et al. Spatial variation in tree demography associated to domestic herbivores and topography: insights from a seeding and planting experiment. For Ecol Manage. 2015;335:139–146.
- Marcora P, Hensen I, Renison D, et al. The performance of Polylepis australis trees along their entire altitudinal range: implications of climate change for their conservation. Divers Distrib. 2008;14(4):630–636.
- Marcora PI, Renison D, País-Bosch AI, et al. The effect of altitude and grazing on seedling establishment of woody species in central Argentina. For Ecol Manage. 2013;291:300–307.
- País-Bosch AI, Tecco PA, Funes G, et al. Efecto de la temperatura en la regeneración de especies leñosas del Chaco Serrano e implicancias en la distribución actual y potencial de bosques. Boletín de la Sociedad Argentina de Botánica. 2012;47(3–4):401–410.
- Cáceres Y, Schrieber K, Lachmuth S, et al. Effects of elevation, land use and microsites on early life performance of a high mountain tree: insights from an in situ sowing experiment. Divers Distrib. 2019;25(10):1537–1550.
- Tecco PA, País-Bosch AI, Funes G, et al. Mountain invasions on the way: are there climatic constraints for the expansion of alien woody species along an elevation gradient in Argentina? J Plant Ecol. 2016;9(4):380–392.
- Seltmann P, Renison D, Cocucci A, et al. Fragment size, pollination efficiency and reproductive success in natural populations of wind-pollinated Polylepis australis (Rosaceae) trees. Flora. 2007;202(7):547–554.
- Hensen I, Teich I, Hirsch H, et al. Range‐wide genetic structure and diversity of the endemic tree line species Polylepis australis (Rosaceae) in Argentina. Am J Bot. 2011;98(11):1825–1833.
- Peng Y, Morales L, Hensen I, et al. No effect of elevation and fragmentation on genetic diversity and structure in Polylepis australis trees from central Argentina. Austral Ecol. 2017;42(3):288–296.
- Marcora PI, Tecco PA, Zeballos SR, et al. Influence of altitude on local adaptation in upland tree species from central Argentina. Plant Biol. 2017;19(2):123–131.
- Schreiber K, Cáceres Y, Engelmann A, et al. Elevational differentiation in metabolic cold stress responses of an endemic mountain tree. Environ Exp Bot. 2020;171:103918.
- Torres RC, Renison D, Hensen I, et al. Polylepis australis’ regeneration niche in relation to seed dispersal, site characteristics and livestock density. For Ecol Manage. 2008;254(2):255–260.
- Kessler M, Kühn A, Solís Neffa VG, et al. Complex geographical distribution of ploidy levels in Polylepis australis (Rosaceae), an endemic tree line species in Argentina. Int J Plant Sci. 2014;175(8):955–961.
- Broadhurst L, Boshier D Seed origin for restoration and management: conserving evolutionary potential and utility. Genetic considerations in ecosystem restoration using native tree species. A thematic study for the State of the World’s Forest Genetic Resources. Edited by. Rome: United Nations Food and Agriculture Organization. 2014;27–37.
- Nicotra AB, Atkin OK, Bonser SP, et al. Plant phenotypic plasticity in a changing climate. Trends Plant Sci. 2010;15(12):684–692.
- Jump AS, Peñuelas J. Running to stand still: adaptation and the response of plants to rapid climate change. Ecol Lett. 2005;8(9):1010–1020.
- Mathez-Stiefel SL, Peralvo M, Báez S, et al. Research priorities for the conservation and sustainable governance of Andean forest landscapes. Mt Res Dev. 2017;37(3):323–339.
- Renison D, Cuyckens GAE, Pacheco S, et al. Distribución y estado de conservación de las poblaciones de árboles y arbustos del género Polylepis (Rosaceae) en las montañas de Argentina. Ecología Austral. 2013;23(1):27–36.
- Seltmann P, Cocucci A, Renison D, et al. Mating system, outcrossing distance effects and pollen availability in the wind-pollinated treeline species Polylepis australis BITT. (Rosaceae) Basic Appl Ecol. 2009;10(1):52–60.
- Colladon L. Síntesis pluviométrica1992–2012. cuenca del Río San Antonio: Sistema del Río Suquía, Provincia de Córdoba. Ezeiza, Instituto Nacional del Agua. 2014, 28 pp. ISBN 978-987-45794-4-3 ISBN 978-987-45194-4-3 978-987-45194-4-3.
- Cingolani AM, Renison D, Zak MR, et al. Mapping vegetation in a heterogeneous mountain rangeland using Landsat data: an alternative method to define and classify land-cover units. Remote Sens Environ. 2004;92(1):84–97.
- Marcora PI, Ferreras AE, Zeballos SR, et al. Context-dependent effects of fire and browsing on woody alien invasion in mountain ecosystems. Oecologia. 2018;188(2):479–490.
- Zimmermann H, Renison D, Leyer I, et al. Do we need livestock grazing to promote Polylepis australis tree recruitment in the central Argentinean mountains? Ecol Res. 2009;24(5):1075–1081.
- Enrico L, Funes G, Cabido M. Regeneration of Polylepis australis Bitt. in the mountains of central Argentina. For Ecol Manag. 2004;190(2–3):301–309.
- Cierjacks A, Iglesias JE, Wesche K, et al. Impact of sowing, canopy cover and litter on seedling dynamics of two Polylepis species at upper tree lines in central Ecuador. J Trop Ecol. 2007;23(3):309–318.
- Tapella MP, Marcora PI, Tecco PA. Reciprocal interactions between a non-native shrub and the dominant native trees of a high mountain woodland: who benefits? Biol Invasions. 2020;23:53–67.
- Parker IM, Rodriguez J, Loik ME. An evolutionary approach to understanding the biology of invasions: local adaptation and general‐purpose genotypes in the weed Verbascum thapsus. Conserv Biol. 2003;17(1):59–72.
- Matesanz S, Horgan-Kobelski T, Sultan SE. Contrasting levels of evolutionary potential in populations of the invasive plant Polygonum cespitosum. Biol Invasions. 2014;16(2):455–468.
- RStudio. Version 1.1.463 – © 2009-2018 RStudio, Inc. Mozilla/5.0 (Windows NT 6.1) AppleWebKit/538.1 (KHTML, like Gecko) rstudio Safari/538.1 Qt/5.4.1.http://www.rstudio.com.
- Di Rienzo JA, Casanoves F, Balzarini MG, Gonzalez L., Tablada M., Robledo C.W. InfoStat versión 2020. Grupo InfoStat, FCA, Universidad Nacional de Córdoba, Argentina. http://www.infostat.com.ar
- Gimeno TE, Pías B, Lemos-Filho JP, et al. Plasticity and stress tolerance override local adaptation in the responses of Mediterranean holm oak seedlings to drought and cold. Tree Physiol. 2009;29(1):87–98.
- Valladares F, Gianoli E, Gómez JM. Ecological limits to plant phenotypic plasticity. New Phytol. 2007;176(4):749–763.
- Balaguer L, Martínez-Ferri E, Valladares F, et al. Population divergence in the plasticity of the response of Quercus coccifera to the light environment. Funct Ecol. 2001;15:124–135.
- Fenner MK, Thompson K. The ecology of seeds. Cambridge: Cambridge University Press; 2005.
- Baskin CC, Baskin JM. Seeds: ecology, biogeography, and, evolution of dormancy and germination. Lexingtown, Kentucky. Elsevier; 1998.
- Bischoff A, Crémieux L, Smilauerova M, et al. Detecting local adaptation in widespread grassland species–the importance of scale and local plant community. J Ecol. 2006;94(6):1130–1142.
- Bischoff A, Steinger T, Müller‐Schärer H. The importance of plant origin and genotypic diversity of seed material used for ecological restoration. Restor Ecol. 2010;18(3):338–348.
- Donohue K, Rubio de Casas R, Burghardt L, et al. Germination, postgermination adaptation, and species ecological ranges. Annu Rev Ecol Evol Syst. 2010;41(1):293–319.
- García‐Fernández A, Iriondo JM, Bartels D, et al. Response to artificial drying until drought‐induced death in different elevation populations of a high‐mountain plant. Plant Biol. 2013;15:93–100.
- Giménez‐Benavides L, Milla R. Comparative germination ecology of two altitudinal vicariant Saxifraga species endemic to the north of Spain. Plant Biol. 2013;15(3):593–600.
- Cáceres Y, Lachmuth S, Schrieber K, Marcora P, Renison D, Hensen I. Germination responses of two key mountains tree species to single and combined fire-related stresses: does elevational origin matter? Flora. 2021, 280:151832. https://doi.org/https://doi.org/10.1016/j.flora.2021.151832
- Cabido MG, Pucheta FE, Vendramini F. A chorological analysis of the mountains from Central Argentina. Is all we call Sierra Chaco really Chaco? Contribution to the study of the flora and vegetation of the Chaco XII. Candollea. 1998;53:321–331.
- Buchner O, Neuner G. Variability of heat tolerance in alpine plant species measured at different altitudes. Arct Antarct Alp Res. 2003;35(4):411–420.
- Scheidel U, Bruelheide H. Altitudinal differences in herbivory on montane Compositae species. Oecologia. 2001;129(1):75–86.
- Rodríguez-Castañeda G, Dyer LA, Brehm G, et al. Tropical forests are not flat: how mountains affect herbivore diversity. Ecol Lett. 2010;13(11):1348–1357.
- Pellissier L, Fiedler K, Ndribe C, et al. Shifts in species richness, herbivore specialization, and plant resistance along elevation gradients. Ecol Evol. 2012;2(8):1818–1825.
- Anderegg WR, Hicke JA, Fisher RA, et al. Tree mortality from drought, insects, and their interactions in a changing climate. New Phytol. 2015;208(3):674–683.
- Larcher W. Temperature stress and survival ability of Mediterranean sclerophyllous plants. Plant Biosyst. 2000;134(3):279–295.
- IPCC. Climate change 2007: impacts, adaptation and vulnerability. Contribution of working Group II to the fourth assessment report of the intergovernmental panel on climate change. 2007. New York, NY: Cambridge University Press, Press. Cambridge, UK
- Allen CD, Macalady AK, Chenchouni H, et al. A global overview of drought and heat-induced tree mortality reveals emerging climate change risks for forests. For Ecol Manage. 2010;259(4):660–684.
- García CL Utilización de información satelital y terrestre para el manejo integrado del recurso hídrico de una cuenca serrana en la Provincia de Córdoba, Argentina [PhD thesis]. Córdoba (CO): Universidad Nacional de Córdoba; 2013. http://hdl.handle.net/11086/1659
- Cochrane A, Yates CJ, Hoyle GL, et al. Will among‐population variation in seed traits improve the chance of species persistence under climate change? Glob Ecol Biogeogr. 2015;24(1):12–24.
’
Table A1. Summary of response variables after three years of translocation experiments under field conditions. Seeds and saplings of Polylepis australis from three origins across it elevation range of distribution were established at three elevation sites