ABSTRACT
The use of macroinvertebrates as indicators of water quality is an effective and low-cost tool, which is widely implemented in biomonitoring programmes. Certain taxa are characteristic of impaired watercourses (e.g. Oligochaeta, Chironomidae and Amphipoda), while others are characteristic of good-quality watercourses (e.g. Ephemeroptera, Plecoptera and Trichoptera; EPT). In this work, we evaluated the response of the macroinvertebrate assemblages to different land uses. For this purpose, artificial substrate colonization experiments were conducted in streams including urban (U, n = 3), intensive agriculture and dairy production (AD, n = 4) and extensive cattle ranching (CR, n = 4) land uses. Because in Uruguay pristine ecosystems are practically non-existent and streams of low order streams associated with extensive cattle production represent the lowest deterioration water quality condition, CR sites were used as control reference streams. Physicochemical water parameters were measured. For macroinvertebrate sampling, 10 artificial substrates were installed in each stream. A total of 110 artificial substrates were analysed. Each sample/site consisted of a plastic mesh bag of 1.2 cm opening, which were filled with 160 cm3 (including 73.3 ± 5.8 cm3 interstitial water) of stones sieved between 1.3 and 1.5 cm. During the summer of 2018 (February), devices were left in streams for 15 days for communities to colonize them. U and AD land use represented the stressors with the highest impact on the attributes and composition of the macroinvertebrate communities. Abundance of EPT was higher in CR, meanwhile Caenogastropoda dominated in AD, and Amphipoda in U sites. Macroinvertebrate groups to order level classification were able to effectively discriminate between different land uses. The use of artificial substrate also demonstrated to be efficient method for monitoring macroinvertebrate community. We did not find a correlation between the physicochemical water parameters and the macroinvertebrate community. In this context, we propose a rapid and cost-effective biomonitoring approach, capable of estimating the degree of impact of different land uses.
Introduction
Changes in land use by human activities produce negative impacts on river systems [Citation1-4]. The magnitude of impacts on ecosystems depends on the intensity and frequency of the different activities. These impacts can include effects on water quality, environmental heterogeneity and biological communities [Citation4–6]. Different activities such as urbanization, intensive agriculture, and livestock have been related to degradation of aquatic ecosystems, but urban land use causes the greatest negative effects due to permanent changes in both the watershed and stream channel, impacts that have limited flexibility for restoration [Citation7]. The soil impermeability generated by cities lead to an increase of magnitude and frequency of floods, generating important hydrological changes, especially in small watersheds with asignificant urbanized area [Citation1,Citation8–10]. In addition, urban land use generates multiple inputs of pollutants in both punctual and diffuse discharge. Punctual discharges of domestic sewage and effluents from industries are frequent in urban systems [Citation1,Citation8,Citation11,Citation12]. Also, the input of contaminants via diffuse sources is very widespread in cities. For instance, the runoff of contaminants during precipitation events [Citation1,Citation4,Citation8]. Among the most common contaminants are heavy metals, hydrocarbon, nutrients, pesticides, [Citation1,Citation4,Citation8] and emerging environmental contaminants, for example pharmaceuticals, hormones and drugs of abuse [Citation4,Citation13,Citation14].
In the last century, the global nitrogen (N) cycle has accelerated due to the extensive use of N fertilisers to boost agricultural production [Citation3]. In addition, intensive farming and land use by this sector causes important changes in freshwater systems, due to significant inputs of organic matter, nutrients, and pesticides, leading to changes in aquatic community and stream functioning [Citation3,Citation6,Citation14–17]. Also, the development of intensive and semi-intensive livestock farming (cattle fattening in confinement) has also been associated with changes in the ecological status of rivers and streams. The effects of these activities on watercourses include changes in the physicochemical conditions of the water, nutrient enrichment, and the arrival of compounds such as metals, animal pharmaceuticals and pesticides, which can harm the aquatic biota [Citation18].
The input of these contaminants into streams causes effects in aquatic populations and communities [Citation2,Citation6,Citation9,Citation11,Citation19–21] that can globally affect ecosystem functioning [Citation17,Citation22]. Communities can respond differently to environmental degradation by different land uses which make the good indicators of different types of pollution [Citation6,Citation20,Citation23]. Within the macroinvertebrate fauna, there are families classified as sensitive to environmental impacts or changes in land use, such as the families Leptophlebiidae (Ephemeroptera), or Helicopsychidae (Trichoptera), which are characterized by requiring high concentrations of dissolved oxygen and low concentrations of organic matter in the water [Citation6,Citation24,Citation25]. Opposite to this group, there are families that tolerate very well high organic matter loads as well as low oxygen concentrations such as the families Hyalellidae (Amphipoda) and Cochliopidae (Caenogastropoda) [Citation26,Citation27].
In the last 20 years, numerous studies have shown that biomonitoring methods can potentially offer different application possibilities in streams [Citation28–30]. These alternatives provide rapid and easy information for the assessment of the environmental quality of aquatic systems [Citation22,Citation31]. Macroinvertebrates, in particular, are widely used as bioindicators because they reflect ecosystem health effectively, due to their high taxonomic diversity, their central position in the food webs, and wide range of sensitivity-tolerance to water quality [Citation32,Citation33]. The application of bioindicators along with physicochemical parameters offers a more integrated view of ecosystem health [Citation7,Citation34]. The use of macroinvertebrates as bioindicators can be implemented using different attributes of the community, like the taxonomic richness, diversity, and the percentage of sensitive taxa (e.g. EPT index, proportion of Ephemeroptera + Plecoptera + Trichoptera), and tolerant taxa (e.g. proportion of Oligochaeta or Amphipoda) [Citation24,Citation25].
As a result, in recent decades in South America, countries such as Argentina, Chile, Brazil, Colombia, Ecuador, and Venezuela have added the use of benthic macroinvertebrates to physicochemical water parameters, for water quality monitoring [Citation35–41]. In the case of Uruguay, the use of macroinvertebrates as indicators of water quality has been poorly implemented, especially in monitoring programs carried out by the environmental authorities. In this regard, it is urgent to develop biomonitoring protocols, especially in the streams that are subjected to greatest impacts from land-use changes [Citation42]. In this case, Uruguayan subtropical streams are influenced by a gradient of anthropogenic deterioration that increases from south to north with the increase of urbanization, which could have stronger and negative effects on water parameters and macroinvertebrates communities [Citation6,Citation17,Citation43].
Given the degradation of the Uruguayan aquatic ecosystems, there is a great need to complement the analysis of physicochemical water parameters with an easy and rapid sampling technique using macroinvertebrate communities, by environmental authorities (DINACEA, Dirección Nacional de Calidad y Evaluación Ambiental).
Among the different sampling techniques, the use of artificial substrates offers an alternative that allows eliminating the effect of sediment heterogeneity, and colonization times, which may exist between different streams [Citation28,Citation44-46]. Although artificial substrates may represent a subsample of the community, they present certain advantages such as easy installation, a homogeneous sample size independent of the characteristics of the stream substrate, the same time of colonization, and the same type of substrate, which favours comparison between different systems and between different times [Citation28,Citation44-46].
The aim of this study was to assess the response of the macroinvertebrate assemblages, to the effects of degradation by different land uses, from Uruguayan lowland streams employing artificial substrates and taxonomic high level. We expected to find an increase of macroinvertebrate richness, abundance and diversity values from less to higher impacted streams, and found representative groups of macroinvertebrates for each land use (extensive cattle ranching, intensive agriculture and dairy production, and urbanization).
Methods
Study area
The study was conducted on 11 streams with three predominant land uses: extensive cattle ranching (CR; n = 4), intensive agriculture and dairy production (AD; n = 4), and urbanization (U; n = 3) (, Table S1). The selected CR sites present 87.2 ± 13.9% of this activity in the basin (), this extensive activity in Uruguay is characterized by including an average of 0.7 cattle per hectare [Citation47]. The selected AD sites represent two combined uses, they present 100% of their watershed cultivated (grains and pastures) for the generation of forage for dairy cattle. These sites also include these cattle in the same area and may present higher densities than the one mentioned for CR. In Uruguay 65% of these farms are characterized by an area between 50 and 500 hectares and generally have more than 60% of improved pastures [Citation47]. The selected urban streams represent sites with a high urban coverage (70 ± 17.3%) corresponding to the most urbanized systems in the city of Maldonado ().
Table 1. Values of the main characteristics of the streams (mean ± standard deviation) in control reference (CR), intensive agriculture and dairy production (AD) and urban sites (U). TP: Total Phosphorus, TN: Total Nitrogen, TDS: Total Dissolved Solids, k: specific conductivity (corrected at 25°C), DO: Dissolved Oxygen. Letters indicate post hoc (Bonferroni adjust) comparison procedure, and different letters indicate significant differences (α < 0.05)
Figure 1. Study area, above is the location at the Uruguayan level. CR: control reference sites are identified in blue, AD: intensive agriculture and dairy production sites are identified in Orange, and U: urbanization sites are identified in red. Three streams are shown below as an example of each of the three selected land uses: del Ombú stream CR, Sauce stream AD, and Salada stream U.
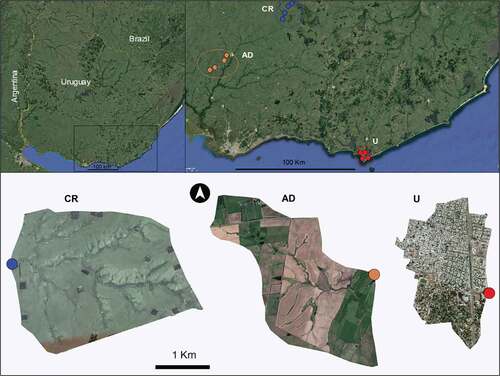
Physicochemical parameters of water quality and habitat
Experiment procedure was carried out during summer 2018. For each site, water samples were taken and the physicochemical parameters were recorded punctually in two dates, at the beginning and the end of the collection of the artificial substrates. For the analyses, the average between both days of sampling was used for each physicochemical variable. In the field, temperature (°C), conductivity (K, µS. cm−1), pH, dissolved oxygen (DO, mg. L−1), and total dissolved solids (mg. L−1) were measured using a YSI 6600 sonde. Water samples were taken and transported at 4°C for subsequent analysis of total phosphorus (PT, mg. L−1) [Citation48], and total nitrogen (NT, mg. L−1) [Citation49] in the laboratory. In addition, depth, width and predominant sediment type were measured at each site in five transects each 10 meters ().
Macroinvertebrate community
For macroinvertebrate sampling, 10 artificial substrates were installed in each stream. A total of 110 artificial substrates were analysed. Each sample/site consisted of a plastic mesh bag of 1.2 cm opening, which were filled with 160 cm3 (including 73.3 ± 5.8 cm3 interstitial water) of stones sieved between 1.3 and 1.5 cm. Artificial substrates were settled in the streams and after 15 days of colonization were removed and transferred to a 500 µm sieve, washed and fixed in 70° ethanol. In the laboratory, the macroinvertebrates were identified to order level, using the taxonomic keys of Dominguez and Fernández, [Citation24], and Hamada et al. [Citation50]. The abundance and taxonomic richness were also determined.
Statistical analysis
Kruskal–Wallis analyses were performed to compare basin area and water variables, width, depth, and substrates between the three land use groups. Then, a post hoc Tukey test with Bonferroni correction was performed [Citation51].
To determine the effects of land use on water quality variables, a principal component analysis (PCA) was performed [Citation52] using the physicochemical mean values of the two samples. To achieve this, multiple correlations between variables were made in order to discard highly correlated variables (>70%), and the Bartlett’s sphericity test was calculated to determine if the number of variables was optimal to perform the PCA. Subsequently, variables were centered and scaled to obtain a correlation matrix, and thus perform multivariate analysis [Citation53]. Percentages of accumulated variance in the synthetic axes or components, and the correlation coefficients of the water variables with the two components were obtained [Citation53]. The PCA was carried out in R using “FactoMineR” [Citation54] and “Factoextra” [Citation55] packages. Values or loads of the first two components were extracted, and ANOVA test were performed to analyse the effects of land use on the water variables summarised in the first two axes of the PCA. Shannon diversity index and richness of orders were determined using Past 2.7 [Citation56]. To assess whether these community attributes differed with basin area and among streams with different land uses, one-way ANCOVA test, followed by a post hoc Tukey test with Bonferroni correction were performed [Citation51].
A non-metric multidimensional scaling (NMDS) ordination was performed in order to understand the spatial variability in the composition of macroinvertebrates. For testing for statistical differences between the macroinvertebrate communities of three groups of sites with different land use, we used a test of similarity or ANOSIM. For ANOSIM and NMDS analyses, the matrix of abundance was transformed into a dissimilarity matrix using Bray–Curtis dissimilarity measure and 9999 permutations [Citation57]. SIMPER analysis was used to detect macroinvertebrate orders responsible for separating each group.
To evaluate whether the taxonomic groups of each land use (SIMPER) differ significantly, we used one-way ANCOVA, including basin area as covariable. Post hoc Tukey test with Bonferroni correction was used when groups were significantly different (p < 0.05) [Citation52].
Mantel test was used to determine the correlation between community composition and water quality parameters [Citation58–61]. For this, macroinvertebrate abundance dissimilarity matrix was created using a distance measure (Bray–Curtis dissimilarity), and water quality parameter distance matrix was generally created using Euclidean distance. For this analysis, we used Spearman correlation for non-parametric data and 999 permutations. Functions “metaMDS”, “anosim”, and “mantel” in the R package “vegan” were used for NMDS, ANOSIM and Mantel test, respectively [Citation53,Citation62].
Results
Physicochemical parameters of water quality
The PCA explained 83.7% of the variability in water quality for the first two components (). The first component (PCA 1 = 48.3% of variance) reflected the effect of water quality separating the most degraded U sites from AD and CR sites (). ANOVA analyses corroborated these groups (F2,10 = 12.2; p = 0.004) and post hoc analyses revealed that urban sites were different from CR and AD sites. In this case, U sites were related to negative values of PCA 1 where total nitrogen content was higher, and AD and CR sites were associated with positive values of PCA 1 where pH was the most influential variable (). The second component (PCA 2 = 35.1%) discriminated between U and CR sites, and these differences were marginally significant (ANOVA: F2,10 = 4.46; p = 0.05). For this component, AD was separated from other sites due to higher values of conductivity (except for AD-2), and CR sites and AD-2 were related to higher values of dissolved oxygen.
Figure 2. Principal component analysis (PCA) of water quality variables in different land use types in the study area. Sampling sites: CR control reference sites are identified in blue, AD: intensive agriculture and dairy production sites are identified in Orange, and U: urbanization sites are identified in red. NT: total nitrogen, K: specific conductivity (corrected at 25°C), OD: dissolved oxygen.
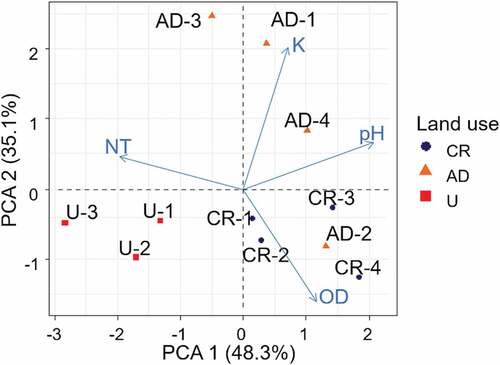
Macroinvertebrate community
A total of 8893 individuals comprising 20 orders () were collected: 7 orders of aquatic insects (47.88% of the total abundance), followed by Mollusca with 5 orders (29.64% in abundance), Annelida (2 orders, 14.63%), and Crustacea (3 orders, 7.70%). The orders with higher occurrence frequency were Ephemeroptera, Coenagastropoda and Diptera.
Table 2. Mean and standard deviation for the total abundance of aquatic macroinvertebrates, for each land use: control reference (CR), intensive agriculture and dairy production (AD) and urban (U)
A total of 2115, 5349 and 1420 macroinvertebrates were caught in CR, AD and U sites, respectively. Abundance showed significant differences among sites (ANCOVA, F2,7 = 5.12, p = 0.04), and did not show an interaction with the basin area (ANCOVA, F2,5 = 0.47, p = 0.65). In this case, AD showed significantly higher abundances than the other two uses ()). Richness also showed significant differences among sites (ANCOVA, F2,7 = 2.11, p = 0.006) and did not show an interaction with the basin area (ANCOVA, F2,5 = 1.11, p = 0.31). Significantly lower richness was presented in U streams with eight orders, followed by AD streams (13 orders) and CR (14 orders) ()). Shannon index showed significant differences among sites (ANCOVA, F2,7 = 2.11, p = 0.006) and did not show an interaction with the basin area (ANCOVA, F2,5 = 0.45, p = 0.95). The differences were significant between AD and U sites ()).
Figure 3. Attributes of the macroinvertebrate community. (a): Total abundance; (b): Order richness; (c): Shannon diversity index. Letters indicate post hoc (Bonferroni adjust) comparison procedure, and different letters indicate significant differences (α < 0.05).
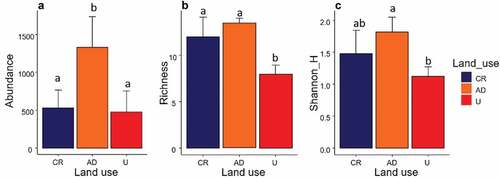
The ordination of spatial variation of stream sites considering the relative abundance of macroinvertebrates taxa, separate CR and AD from U sites (), and the differences between groups were significant (ANOSIM, r2 = 0.75, p < 0.001).
Figure 4. Non-metric Multidimensional Scaling (NMDS) ordination of macroinvertebrate community in different land use types in the study area. Stress: 0.071. Sampling sites: CR: control reference sites, AD: intensive agriculture and dairy production sites, and U: urbanization sites.
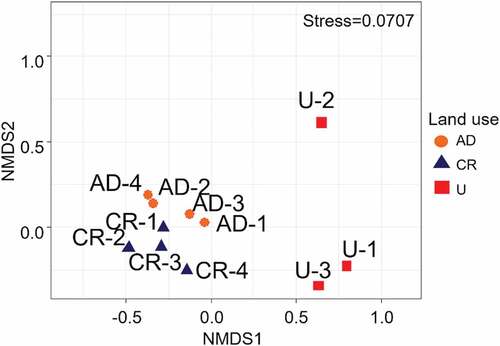
The SIMPER analysis indicated that taxa representing several order groups contributed to differences between the three land uses. In particular, U sites were characterised by a high relative abundance of Amphipoda (U mean = 22.68 vs. AD mean = 3.72, and CR mean = 0.25). Intensive AD sites were dominated by a greater relative abundance of Caenogastropoda (AD mean = 47.01 vs. U mean = 0.33, and CR mean = 0.37). In CR, Trichoptera (CR mean = 6.30 vs. AD mean = 4.52, U mean = 1.0) was highly represented (). Ephemeroptera was highly represented in AD and CR streams (AD mean = 14.85, and CR mean = 12.63 vs. U mean = 0.0) ().
Table 3. SIMPER analyses: Percent contribution of the species by land use group
The ANCOVA analysis (using basin area as a covariable) was performed for each of the above-mentioned groups. In the case of Ephemeroptera and Trichoptera, we have grouped them in the EPT index, due to its wide use and the presence of some Plecoptera individuals. EPT index showed significant difference between land uses (ANCOVA, F2,7 = 34.02, p = 0.0003) and did not show an interaction with the basin area (ANCOVA, F2,5 = 1.58, p = 0.293). In this case, CR presented significantly higher values than AD and U sites ()). Coenogastropoda showed significant difference between land uses (ANCOVA, F2,7 = 25.55, p = 0.0006) and did not show an interaction with the basin area (ANCOVA, F2,5 = 0.34, p = 0.729). Coenogastropoda showed higher and significant abundance in AD compared with CR and U sites ()). Amphipoda showed significant difference between land uses (ANCOVA, F2,7 = 41.43, p = 0.0001), and did not show an interaction with the basin area (ANCOVA, F2,5 = 0.56, p = 0.604). The U sites showed significant higher values ()). The water parameters were poorly correlated with the macroinvertebrate community (Mantel statistic r: 0.283, p = 0.068).
Figure 5. Relative abundance of the most influential macroinvertebrates on each site (above) and land use type (below). CR: control reference sites, AD: intensive agriculture and dairy production sites, and U: urbanization sites. Letters indicate post hoc (Bonferroni adjust) comparison procedure, and different letters indicate significant differences (α < 0.05).
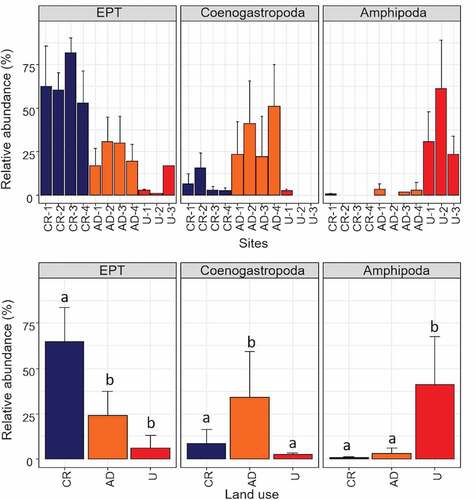
Discussion
Our results provide the first rapid description of macroinvertebrate community composition in lowland Uruguayan streams with different land use performed with artificial substrates. The results showed that different land uses have strong and differential effects on water quality, and in the macroinvertebrate community. However, based on Mantel test, water quality was not related to the macroinvertebrate communities. All water quality parameters analysed comply with the legislation (National Decree, Dec 253/79 of Uruguay), except phosphorus (maximum allowed 25 µg.L−1), values that very few streams in Uruguay satisfy. This allows us to observe that in compliance with national regulations, the streams present a clear degradation of their macroinvertebrate communities. This work, as well as others [Citation12], shows that the current normative values should be reconsidered, a clear example is the allowed concentration of 10 mg.L−1 for Nitrogen (equivalent to 44.3 mg.L−1 of Nitrate, NO3), concentrations that could only be exceeded by the most degraded urban streams in Uruguay.
Anthropogenic activities have disturbed and modified existing processes and/or have included new sources of water pollution through different pathways (e.g. sewage, superficial and sub-superficial drainage) [Citation63]. Physicochemical water parameters provide a correct view of the system; however, they only show the information in a particular moment because of their high temporal variability [Citation31]. Accordingly to other studies, it could be observed that the highest nutrient concentrations, conductivity and organic matter values, were associated with urban and intensive agriculture and dairy production streams [Citation3,Citation4]. These are the expected results due to punctual and diffuse contamination that increased nutrients and sediment reaching the streams [Citation1,Citation3,Citation64]. The low oxygen concentration in these streams, which has been observed in other lowland streams, could be a response to low flow conditions, increased nutrient concentrations, organic matter and higher temperatures leading to periods of hypoxia [Citation3,Citation65].
The ecological status of streams and their biota is a response to environmental stressors across several spatial scales, such as changes in land use [Citation4,Citation21,Citation66]. Therefore, urban and agricultural land use represent the stressors with the highest intensity of impact, and in most cases, they are irreversible [Citation1,Citation7]. We observed that urban presented lower values of richness and diversity, as well as a more simplified macroinvertebrate assemblages, implying that this land use represents the main stress factors threatening aquatic communities [Citation7,Citation42,Citation67]. In this case, the effects of urbanization on aquatic biota are usually of great intensity, in many cases causing the local extinction of sensitive populations [Citation21].
With the increase of water pollution, the abundance of sensitive taxa decreased, allowing tolerant taxa to increase, showing a higher numerical dominance [Citation23,Citation68,Citation69]. An interesting aspect is the increased abundance of amphipods in urban streams and their low presence or absence in other streams. These organisms have demonstrated a plasticity of resource use, especially of organic matter in sediment [Citation26,Citation70] which dominates in urban systems from effluent inflow. However, these crustaceans have been considered sensitive taxa in water bodies associated with agriculture due to their high sensitivity to pesticides [Citation71]. In CR and AD streams, communities had higher values of richness and diversity, but AD presented higher abundance of macroinvertebrates, especially the Coenogastropoda (Gasteropoda). These gastropods present great adaptive potential to agrochemicals, which allows them to take advantage of the availability of organic matter produced by agricultural activities [Citation27]. Another difference between AD and CR is that in CR there is a higher relative abundance of EPT. These groups are frequently found in clean and oxygenated water courses [Citation24,Citation25].
The effect of water quality on macroinvertebrate communities is a well-understood phenomenon. However, our results showed a strong relationship with land use, but a weak relationship with water quality. These results may not be expected, but the variability in water quality is usually very high (even daily) compared to the variability of biological communities [Citation25,Citation40]. For this reason, point sampling of water quality is not always explanatory of communities [Citation25,Citation31,Citation40]. The effect of land use is more constant over time, including other effects beyond water quality (e.g. habitat fragmentation), and is often a better indicator of biological communities [Citation31].
The loss of diversity in heavily impacted streams like urban streams is often significant and difficult to recover [Citation72]. The landscape of urban streams also affects the dispersion and fragmentation, which have immediate effects on the biota of the system. This has consequences to life cycles in terrestrial habitats and limits the dispersal of adult insects in streams [Citation5,Citation32,Citation72]. In contrast, streams with extensive livestock (CR) these areas with low cattle densities tend to have lower intensity and the effects are generally reversible, as the biota can persist as long as it adapts to the stress conditions [Citation21]. In our case, CR communities tended to be more diverse, and showed a greater abundance of taxa that are sensitive to disturbance. This indicates that with good management conditions and restoration programs, communities and ecosystem health can be maintained over time. It should be noted that Uruguayan pristine ecosystems are practically non-existent after European colonization [Citation42], and lowland streams associated to low-density cattle production represent the lowest impacted streams with the best water quality condition in Uruguay [Citation66], for this reason many of these streams can be considered as reference streams. The use of a coarse taxonomic level, in this case, was useful and sufficient as it significantly separated the different land uses [Citation25,Citation73]. This situation can be considered an advantage since it avoids one of the main difficulties of biomonitoring, which is the need for increasingly specialized technicians to work with lower taxonomic levels (i.e. family or genus).
On the other hand, it could be demonstrated that the use of artificial substrates allowed discrimination of the impact of different land uses on the benthic community. For these reasons, the use of this technique can allow a quick and reliable evaluation of the streams, granting an accessible monitoring that allows to know the ecological state of the systems over time [Citation45,Citation74]. These points are important because macroinvertebrate communities can be strongly influenced by sediment characteristics and colonization periods [Citation75], and these differences could confound the effects of water quality [Citation76].
In this context, we propose a rapid and cost-effective biomonitoring approach, capable of estimating the degree of impact of three different land uses in streams in Uruguay. It should be noted that here, we present a rapid monitoring strategy that has been proven to be effective, although the taxonomic level is valid in this context. However, for ecological studies, it is necessary to include a lower taxonomic level according to the questions to be answered (e.g. family, genus, species).
Acknowledgments
This work was carried out in the framework of the “Núcleo Interdisciplinario Aguas Urbanas: Proyecto y Gestión” supported by the “Espacio Interdisciplinario de la Universidad de la República”. FTM is supported by the SNI (“Sistema Nacional de Investigadores”) and PEDECIBA “Geociencias and Biologia” (“Programa de Desarrollo de las CienciasBásicas”). MB is supported by the doctoral scholarship of the SNB-ANII (“Sistema Nacional de Becas de la Agencia Nacional de Investigación e Innovación”). We would like to thank Magda Gonzalez, Lucia Urtado, Iván Gonzalez-Bergonzoni, Guillermo Goyenola, Maite Burwood, Natalie Corrales and Natalia Vilaboa, for their collaboration in the field and Camila Vidal for the revision of the English language. To the two reviewers who helped improve the manuscript.
Disclosure statement
No potential conflict of interest was reported by the author(s).
References
- Walsh CJ, Roy AH, Feminella JW, et al. The urban stream syndrome: current knowledge and the search for a cure. J N Am Benthol Soc. 2005;24(3):706–723.
- Zaruki M, González-Bergonzoni I, Teixeira de Mello F, et al. Fish diversity loss in an urban stream of Uruguay throughout the last century. Pan-Am J Aquat Sci. 2011;6(1):71–75.
- Goyenola G, Graeber D, Meerhoff M, et al. Influence of farming intensity and climate on lowland stream nitrogen. Water. 2020;12(4):1021.
- Liu Z, Zhou T, Cui Y, et al. Environmental filtering and spatial processes equally contributed to macroinvertebrate metacommunity dynamics in the highly urbanized river networks in Shenzhen, South China. Ecol Process. 2020;10(1):1–12.
- Monaghan RM, Wilcock RJ, Smith LC, et al. Linkages between land management activities and water quality in an intensively farmed catchment in southern New Zealand. Agric Ecosyst Environ. 2007;118(1–4):211–222.
- Chalar G, Arocena R, Pacheco JP, et al. Trophic assessment of streams in Uruguay: a trophic state index for benthic invertebrates (TSI-BI). Ecol Indic. 2011;11(2):362–369.
- Rodríguez-Olarte D, Barrios M, Caputo L, et al. Criterios para la evaluación de estresores y parámetros en la estimación del estado ecológico de ríos en Suramérica. [Criteria for the evaluation of stressors and parameters in the estimation of the ecological status of rivers in South America]. Barquisimeto: Serie PublicacionesEspeciales. Museo de Ciencias Naturales. Universidad Centroccidental Lisandro Alvarado (UCLA); 2020. Spanish.
- Paul MJ, Meyer JL. Streams in the urban landscape. Annu Rev Ecol Evol Syst. 2001;32(1):333–365.
- Gurnell A, Lee M, Souch C. Urban rivers: hydrology, geomorphology, ecology and opportunities for change. Geogr Compass. 2007;1(5):1118–1137.
- Roy AH, Purcell AH, Walsh CJ, et al. Urbanization and stream ecology: five years later. J N Am Benthol Soc. 2009;28(4):908–910.
- Vidal N, Loureiro M, Hued AC, et al. Female masculinization and reproductive success in cnesterodondecemmaculatus (Jenyns, 1842) (Cyprinodontiforme: poeciliidae) under anthropogenic impact. Ecotoxicology. 2018;27(10):1331–1340.
- Alvareda E, Lucas C, Paradiso M, et al. Water quality evaluation of two urban streams in Northwest Uruguay: are national regulations for urban stream quality sufficient? EnvironMonitAssess 2020;192(10):1–22.
- Griffero L, Alcántara-Durán J, Alonso C. Rodríguez-Gallego L, et al.Basin-scale monitoring and risk assessment of emerging contaminants in South American Atlantic coastal lagoons. Sci Total Environ. 2019;697:134058.
- Goyenola G, Meerhoff M, Teixeira Demello F, et al. Monitoring strategies of stream phosphorus under contrasting climate-driven flow regimes. Hydrol Earth Syst Sci. 2015;19(10):4099–4111.
- Soutullo A, Ríos M, Zaldúa N, et al. Soybean expansion and the challenge of the coexistence of agribusiness with local production and conservation initiatives: pesticides in a Ramsar site in Uruguay. Environ Conserv. 2020;47(2):97–103.
- Graeber D, Goyenola G, Meerhoff M, et al. Interacting effects of climate and agriculture on fluvial DOM in temperate and subtropical catchments. Hydrol Earth Syst Sci. 2015;19(5):239–2377.
- Burwood M, Clemente J, Meerhoff M, et al. Macroinvertebrate communities and macrophyte decomposition could be affected by land use intensification in subtropical lowland streams. Limnetica. 2020;40(2):343–357.
- Horak CN. Influencia de las prácticas agropecuarias sobre cursos de agua de Patagonia: empleo de macroinvertebrados y sistema de defensa a múltiples agentes xenobióticos (MXR) comobiomonitores. [Influence of agricultural practices on Patagonian water courses: use of macroinvertebrates and multiple xenobiotic defence systems (MXR) as biomonitors]. [ dissertation], Buenos Aires, Universidad Nacional de La Plata, Argentina; 2020. Spanish.
- Carrasco-Letelier L, Eguren G, Teixeira de Mello F, et al. Preliminary field study of hepatic porphyrin profiles of astyanax fasciatus (Teleostei, Characiformes) to define anthropogenic pollution. Chemosphere. 2006;62(8):1245–1252.
- Benejam L, Teixeira de Mello F, Meerhoff M, et al. Assessing effects of change in land use on size-related variables of fish in subtropical streams. Can J Fish Aquat Sci. 2016;73(4):547–556.
- Gituanja GG. Impacts of land use and land cover on water quality and benthic macroinvertebrates in theta river catchment. [ dissertation]. Nairobi: University of Nairobi; 2020.
- Fenoglio S, Doretto A. Monitoring of neotropical streams using macroinvertebrate communities: evidence from Honduras. Environments. 2021;8(4):27.
- Castro M, Capurro L, Chalar G, et al. Macroinvertebrados bentónicos indican empeoramiento de la calidad de agua en una zona de influencia urbana en el Río Negro [Benthicmacroinvertebratesindicateworsening wáter quality in a zone of urban influence in the Rio Negro]. Bol Soc Zool Uruguay. 2020;29(2):116–125. Spanish.
- Domínguez E, Fernández HR, editors. Macroinvertebrados bentónicos sudamericanos. Sistemática y biología [South American benthic macroinvertebrates. Systematics and biology]. Tucumán: Fundación Miguel Lillo; 2009. Spanish.
- Springer M. Chapter 7: Trichoptera In: Springer M, Ramírez A, Hanson P, editors. Macroinvertebrados de agua dulce de Costa Rica I [Freshwater macroinvertebrates of Costa Rica I]. Costa Rica: Rev Biol Trop. 2010;58 Suppl 3:151–198. Spanish.
- Saigo M, Marchese Garello MR, Montalto L. Hábitos alimentarios de Hyalella curvispina Shoemaker, 1942 (Amphipoda: gammaridea) en ambientes leníticos de la llanura aluvial del río Paraná Medio [Feeding habits of Hyalella curvispina Shoemaker, 1942 (Amphipoda: gammaridea) in Lenithic environments of the alluvial plain of the Middle Paraná River]. Nat Neotrop. 2010;40(1,2):43–59.
- Juárez I, Crettaz Minaglia MC, Gianello D, et al. Diagnóstico ambiental de la cuenca media-baja del arroyo Santa Bárbara (Gualeguaychú, Entre Ríos) [Environmental diagnosis of the lower-middle basin of the Santa Bárbara stream (Gualeguaychú, Entre Ríos)]. Scientia Interfluvius. 2018;9:46–70. Spanish.
- Cairns J Jr, Pratt JR. A history of biological monitoring using benthic macroinvertebrates. In: Rosenberg DM, Resh VH, editors. Freshwater biomonitoring and benthic macroinvertebrates. New York (NY): Chapman/Hall; 1993. p. 10–27.
- Lenat DR, Resh VH. Taxonomy and stream ecology—the benefits of genus-and species-level identifications. J N Am Benthol Soc. 2001;20(2):287–298.
- Waite IR, Herlihy AT, Larsen DP, et al. The effects of macroinvertebrate taxonomic resolution in large landscape bioassessments: an example from the Mid‐Atlantic Highlands, USA. Freshw Biol. 2004;49(4):474–489.
- Valverde-Legarda NL, Caicedo Quintero O, Aguirre-Ramírez NJ. Análisis de calidad de agua de la quebrada La Ayurá con base en variables fisicoquímicas y macroinvertebrados acuáticos [Water quality analysis of the Ayurá stream based on physicochemical variables and aquatic macroinvertebrates]. Rev P+L. 2009;4(1):45–60. Spanish.
- Sermeño-Chicas JM, Serrano-Cervantes L, Springer M, et al. Determinación de la calidad ambiental de las aguas de los ríos de El Salvador, utilizando invertebrados acuáticos: índice biológico a nivel de familias de invertebrados acuáticos en El Salvador [Determination of the environmental quality of the waters of El Salvador’s rivers using aquatic invertebrates: biological index at the level of families of aquatic invertebrates in El Salvador]. El Salvador: Editorial Universitaria; 2010 Spanish.
- Akamagwuna FC, Odume ON. Ephemeroptera, plecoptera and trichoptera (EPT) functional feeding group responses to fine grain sediment stress in a river in the Eastern Cape, South Africa. Environ Monit Assess. 2020;192(4):1–11.
- Crettaz-Minaglia MC, Juaréz RA, Aguer I, et al. Aplicación de índices de calidad de agua en un arroyo pampeano utilizando macroinvertebrados bentónicos como bioindicadores (Gualeguaychú, Entre Ríos, Argentina) [Application of water quality indices in a Pampean stream using benthic macroinvertebrates as bioindicators (Gualeguaychú, Entre Ríos, Argentina)]. Biol Acuat. 2014;(30:93–105. Spanish.
- Barrios M, Rodríguez-Olarte D, García E. Índice de integridad de los ecosistemas fluviales con base a las comunidades de insectos acuáticos en el río Misoa de la cuenca del lago de Maracaibo, Venezuela [Index of river ecosystem integrity based on aquatic insect communities in the Misoa River of the Maracaibo Lake basin, Venezuela]. Entomotropica. 2014;30:69–83. Spanish.
- Ríos-Touma B, Acosta R, Prat N. The Andean biotic index (ABI): revised tolerance to pollution values for macroinvertebrate families and index performance evaluation. Rev Biol Trop. 2014;62:249–273.
- Damanik-Ambarita MN, Lock K, Boets P, et al. Ecological water quality analysis of the Guayas river basin (Ecuador) based on macroinvertebrate indices. Limnologica. 2016;57:27–59.
- Fierro P, Valdovinos C, Vargas-Chacoff L, et al. Macroinvertebrates and fishes as bioindicators of stream water pollution Hlanganani, T, Bronwyn, P G . In: Chapter 2. SPi global, editors. water quality. Croatia: Intechopen, Rijeka; 2017. p. 23–38.
- Santos JDL, da Conceição AA, Macedo CF, et al. Water quality biomonitoring of a stream in serra da jiboia (Bahia/Brazil) using benthic macroinvertebrates. Magistra. 2017;29(2):235–245.
- Alonso JA. Índice multimétrico basado en macroinvertebrados acuáticos para evaluar la calidad de las aguas en arroyos del sur del departamento de Itapúa. Encarnación, Paraguay [Multimetric index based on aquatic macroinvertebrates to evaluate water quality in arroyos del sur of the department of Itapúa. Encarnacion, Paraguay]. [ dissertation]. Encarnación: Universidad Nacional de Itapúa, Paraguay; 2018. Spanish
- López JPS, Mc Cann DSF, Macías FDJV, et al. An image processing method for recognition of four aquatic macroinvertebrates genera in freshwater environments in the Andean region of Colombia. Environ Monit Assess. 2020;192(10):1–11.
- Torremorell A, Hegoburu C, Brandimarte AL, et al. Current and future threats for ecological quality management of South American freshwater ecosystems. Inland Water. 2021;11(2):125–140.
- Arocena R. La comunidad bentónica como indicadora de zonas de degradación y recuperación en el arroyo toledo (Uruguay) [The benthic community as an indicator of degradation and recovery zones in the toledo stream (Uruguay)]. Rev Biol Trop. 1996;44(2A):659–671. Spanish.
- Rosenberg DM, Resh VH. The use of artificial substrates in the study of freshwater benthic macroinvertebrates. In: Cairns J, editor. Artificial substrates. Ann Arbor. Michigan (MI): Ann Arbor Science Publishers Inc; 1982. p. 175–235.
- Gómez S, Manríque E. Colonización de sustratos por macroinvertebrados acuáticos en las aguas de la Quebrada Charquira del municipio de Carmen de Carupa [Colonization of substrates by aquatic macroinvertebrates in the waters of the Quebrada Charquira of the municipality of Carmen de Carupa]. [dissertation]. Caldas:Universidad Distrital Francisco José de Caldas; 2017. Colombia.
- Casey RJ, Kendall SA. Comparisons among colonization of artificial substratum types and natural substratum by benthic macroinvertebrates. Hydrobiologia. 1996;341(1):57–64.
- DIEA 2020. Anuario Estadístico Agropecuario. Estadísticas agropecuarias. Ministerio de Ganadería, Agricultura y Pesca. [Internet], [2021 Nov 14]. Montevideo. Available from: https://descargas.mgap.gub.uy/DIEA/Anuarios/Anuario2020/ANUARIO2020.pdf
- Valderrama JC. The simultaneous analysis of total N and total P in natural waters. Mar Chem. 1981;10(2):109–122.
- Müller R, Wiedemann O. Die bestimmung des nitrat- ionen in wasser [The determination of the nitrate ions in water]. Von Wasser. 1955;22:247–271. German.
- Hamada N, Thorp JH, Rogers DC, editors. Thorp and covich’s freshwater invertebrates: volume 3: keys to neotropical hexapoda. Fourth edition ed. London: Academic Press. Elsevier; 2018.
- Crawley MJ. The R book. Chichester: John Wiley & Sons Ltd.; 2012.
- Legendre P, Legendre LF. Numerical ecology. Amsterdam: Elsevier.; 2012.
- Borcard D, Gillet F, Legendre P, editors. Numerical ecology with R. Cham: Springer; 2018.
- Lê S, Josse J, Husson F. FactoMineR: an R package for multivariate analysis. J Stat Softw. 2008;25(1):1–18.
- Kassambara A, Mundt F. Factoextra: extract and visualize the results of multivariate data analyses. R Package Version. 2017;1:337–354.
- Hammer Ø, Harper DA, Ryan PD. PAST: paleontological statistics software package for education and data analysis. Palaeontol Electron. 2001;4(1):9.
- Queen JP, Quinn GP, Keough MJ. Experimental design and data analysis for biologists. Cambridge: Cambridge University press; 2002.
- Heino J, Tolonen KT. Ecological drivers of multiple facets of beta diversity in a lentic macroinvertebrate metacommunity. Limnol Oceanogr. 2017;62(6):2431–2444.
- Hu Y, Dou X, Li J, et al. Impervious surfaces alter soil bacterial communities in urban areas: a case study in Beijing, China. Front Microbiol. 2018;9:226.
- Zhou S, Sun Y, Yu M, et al. Linking shifts in bacterial community composition and function with changes in the dissolved organic matter pool in ice-covered Baiyangdian Lake, Northern China. Microorganisms. 2020;8(6):883.
- Galeti G, Capitanio BM, Baldissera R. Variation of benthic macroinvertebrate communities in streams of three landscapes of South Brazilian grasslands. Revista de Biología Tropical. 2020;68(1):108–121.
- Oksanen J, Kindt R, Legendre P, et al. The vegan package. Commun Ecol Pack R pack vers. 2007;10(631–637):719.
- Vrebos D, Beauchard O, Meire P. The impact of land use and spatial mediated processes on the water quality in a river system. Sci Total Environ. 2017;601:365–373.
- Allan JD. Landscapes and riverscapes: the influence of land use on stream ecosystems. Annu Rev Ecol Evol Syst. 2004;35(1):257–284.
- Frau D, Gutierrez MF, Regaldo L, et al. Plankton community responses in Pampean lowland streams linked to intensive agricultural pollution. Ecol Indic. 2021;120:106934.
- Goyenola G, Kruk C, Mazzeo N, et al. Producción, nutrientes, eutrofización y cianobacterias en Uruguay [Production, nutrients, eutrophication and cyanobacteria in Uruguay. INNOTEC. 2021 22 ;e558–e558.
- Sabater S, Elosegi A, Ludwig R. Multiple stressors in river ecosystems. In: Sabater S, Elosegi A, Ludwig R, editors. Status, impacts and prospects for the future. Amsterdam: Elsevier; 2018.
- Jones JR, Smart MM, Tracy BH, et al. Biotic index tested for ability to assess water quality of Missouri Ozark streams. Trans Am Fish Soc. 1981;110(5):627–637.
- Jones FC. Taxonomic sufficiency: the influence of taxonomic resolution on freshwater bioassessments using benthic macroinvertebrates. Environ Rev. 2008;16(NA):45–69.
- Suedel BC, Rodgers JJH. Development of formulated reference sediments for freshwater and estuarine sediment testing. Environ Toxicol Chem. 1994;13(7):1163–1175.
- Hunt L, Bonetto C, Marrochi N, et al. Species at risk (SPEAR) index indicates effects of insecticides on stream invertebrate communities in soy production regions of the Argentine Pampas. Sci Total Environ. 2017;580:699–709.
- Booth DB, Karr JR, Schauman S, et al. Reviving urban streams: land use, hydrology, biology, and human behavior. J Am Water Resour Assoc. 2004;40(5):1351–1364.
- Ruellet T, Dauvin JC. Benthic indicators: analysis of the threshold values of ecological quality classifications for transitional waters. Mar Pollut Bull. 2007;54(11):1707–1714.
- Boothroyd IKG, Dickie BN. Macroinvertebrate colonisation of perspex artificial substrates for use in biomonitoring studies. N Z J Mar Freshwater Res. 1989;23(4):467–478.
- Brooks SS, Boulton AJ. Recolonization dynamics of benthic macroinvertebrates after artificial and natural disturbances in an Australian temporary stream. Mar Freshw Res. 1991;42(3):295–308.
- Quesada-Alvarado F, Solano-Ulate D. Colonización de macroinvertebrados acuáticos en tres tipos de sustratos artificiales, en un río tropical. Rev Biol Trop. 2020;68(S2):S68–S78.