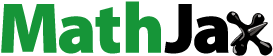
ABSTRACT
This study aims to provide a framework to analyse future flood scenarios considering the effects of three main drivers of flood aggravation: climate change (rainfall intensification and sea level rise); unplanned urbanization; and poor maintenance of urban drainage systems. These stressors were chosen because they represent the aggravation of a natural phenomenon, the urban sprawl effects and the degradation of the drainage system. This analysis intends to make it clear what the main drivers of increasing risks are. Subsequently, a uchronic scenario is developed to analyse how adequate urban planning and infrastructure provision can contribute to a sustainable and resilient city regarding flood alleviation. The method is supported by hydrodynamic simulation. Results demonstrate that precarious urban growth without providing adequate infrastructure coverage can be even more dramatic than climate change. Otherwise, the uchronic scenario demonstrates the benefits of adequately planning cities in a sustainable way, giving lessons to this process.
1. Introduction
Flooding is a worldwide disaster that negatively impacts socioeconomic systems causing significant damages and losses (Nofal & Van De Lindt, Citation2022). Recent examples of noteworthy events in Germany, the Netherlands, Belgium and China illustrate their substantial capacity to drastically damage cities and impact their citizens. Although greatly varying in magnitude and depending on several site-specific factors, floods affected more than 1.65 billion people between 2000 and 2019, representing around 44% of all natural disasters recorded during this period (CRED & UNDRR, Citation2020). For decades, a plethora of distinct urban drainage strategies have been developed and implemented as an attempt to minimize the potential of floods to disrupt urban systems (Fletcher, Shuster, Hunt, et al., Citation2015). However, it is commonly seen that new implementations and more investments continue to be done without really reversing the situation. Flooding remains an issue even after the adoption of hard engineering measures such as underground drainage systems, detention basins and artificial channels (Sayers, Yuanyuan, Galloway, et al., Citation2013), which means that even though several efforts are being carried out to mitigate floods, their consequences remain significant and can even increase in time, following urbanization and population increasing trends, which, in turn, expose more people and assets to floods.
Realising this situation is a fact that allowed a reasoning shift, in which it becomes more important to reduce flood risks than simply reducing flood depths. In this context, flood hazards are combined with the socio-economic aspects of the exposed systems regarding its vulnerability, intrinsic value and resilience, being a topic that is gaining more space in the technical literature.
1.1. Previous studies
The performance analysis of the critical urban infrastructure was traditionally made by classical risk assessment methods, influencing the evolution of flood risk management approaches over the past two decades. The transition from structural protection towards a risk-based strategy followed by a more holistic approach has been mainly driven by the concept of resilience, which is becoming more frequent since the beginning of this century (Zevenbergen, Gersonius, & Radhakrishan, Citation2020). Additional efforts have been requested beyond the well-established state-of-the-art to ensure efficient recovery from low probability high-impact disruptive events (Panteli & Mancarella, Citation2015).
In this context, increased attention is given worldwide to the resilience of infrastructure systems, which is considered a key property to deal with disruptions avoiding cascading effects and spreading losses in the territory (S. Jackson, Citation2015). Resilience is associated with the capacity of a system to absorb impacts and maintain its functions, that is, to survive or persist in an environment with variations and uncertainties over time. In this way, the resilience concept matches the sustainable purposes, since both approaches look into the future trying to guarantee functional systems and better quality of life. However, risks are constantly evolving and that is why current approaches may no longer be fully sufficient in the future (Gasser, Lustenberger, Cinelli, et al., Citation2019). The way the system responds to a given challenge is what will determine the degree of its resilience.
In this way, the uncertainties brought by climate change scenarios, as long as they are well-mapped to probable future scenarios, can be useful and necessary tools for urban planning (Brandt, Lim, Colding, et al., Citation2021). Mapping the areas affected by climate change allows for properly managing the city risks and preparing it to respond appropriately (Avand, Moradi, & Ramazanzadeh Lasboyee, Citation2021).
In addition, more resilient and adaptive flood mitigation structures respond better to future uncertainties (Li et al., Citation2019; Singh, Amekudzi-Kennedy, Ashuri, et al., Citation2022). Low Impact Development practices (W. Yang, Zhang, & Krebs, Citation2022) and nature-based solutions such as those composed by the use of green and blue infrastructure (O’donnell, Thorne, Ahilan, et al., Citation2020) have been presented as alternatives to better respond to climate uncertainties.
Infrastructure can reduce runoff, increase biodiversity, provide cultural and health benefits through public access to valuable natural resources (Kaur & Gupta, Citation2022) and generate the necessary connections to fragmented natural open spaces (Oliveira, Sousa, Rezende, et al., Citation2022).
Thorne, Lawson, Ozawa, et al. (Citation2018) highlight that green and blue infrastructure can help to extend the useful life of old gray assets, bringing multifunctional characteristics to the system. The traditional infrastructure approach is generally based on impermeable pavements and direct drainage rapidly conveying discharges through storm drains and artificial channels to receiving water bodies (Johnson, Citation2013). This type of infrastructure heavily driven by concrete works is usually called gray infrastructure Thus, green and blue infrastructure can be combined with the traditional gray infrastructure to optimize the drainage system and offer the best of flood risk reduction, continuous service delivery, and other benefits (Thorne, Lawson, Ozawa, et al., Citation2018).
A multitude of factors can be cited to explain the persistence of flood-related issues. However, two factors usually appear as the most prominent, referring to climate change increasing rainfall intensity, and urban growth processes leading to land use/land cover (LULC) changes that significantly alter the urban water cycle (Adnan, Abdullah, Dewan, et al., Citation2020). In fact, these two factors bring an interesting discussion to light. The climate change effects can affect the natural phenomenon of rainfall events, increasing floods. On the other hand, inadequate land use planning with unsustainable changes can aggravate the runoff generation, also increasing floods, even if the rainfall is the same. Therefore, flooding is consequence of the rainfall intensity reaching a watershed where its interaction with local land use and landscape will determine the criticality of the consequent flood events. If rainfall intensity increases and urban growth occurs in an undesired way, floods will get worse. In this context the drainage system occupies an intermediate position between natural and urban needs, offering a floodway, while maintaining the city safe and functional.
Climate change is expected to occur worldwide (IPCC, Citation2022; Kharin, Zwiers, Zhang, et al., Citation2013). For instance, Imbach, Chou, Lyra, et al. (Citation2018) indicate that countries of the Atlantic coast of Central America are expected to experience events of more than 50 mm per day more frequently, and Dos Santos, Pedra, Silva, et al. (Citation2020) verified increases of up to 25% of total annual rainfall for the South, Southeast and Centre-West states of Brazil. Several other studies also indicate similar trends of rainfall intensification in distinct locations of the world (Brêda, Paiva, Collischon, et al., Citation2020; Karki, Hasson, Schickhoff, et al., Citation2017; Mukherjee, Aadhar, Stone, et al., Citation2018; Tabari, Citation2020; Y. Zhang, Wang, Chen, et al., Citation2019). Increases of rainfall intensity have a great potential to aggravate flood problems, as higher volumes of water would reach city environments and put current urban drainage strategies to the test. Moreover, climate change effects on urban water cycles could potentially aggravate even more flood problems in coastal areas because of mean sea level rise. On the other hand, during dry seasons, droughts and water scarcity are also expected to be more frequent, also spreading socioeconomic and environmental negative consequences.
Even though projections of future mean sea levels vary across different research works and are inherently uncertain, studies agree that sea level rise is likely to happen in the future (Garner, Weiss, Parris, et al., Citation2018; L. P. Jackson, Grinsted, & Jevrejeva, Citation2018; Spencer, Strobl, & Campbell, Citation2022). Therefore, the consideration of potential increases of current mean sea level for a specific region should be a mandatory aspect for flood modelling in coastal watersheds when prospecting urban drainage solutions.
Whilst climate change potentially increases the volumes of water reaching the urban surface, LULC changes due to urbanization can greatly contribute to higher runoff generation, affecting the other side of the problem, related with the way through which rainfall interacts with the watershed. While natural land covers offer several opportunities for water to infiltrate or accumulate in the watershed, urban areas commonly disrupt important hydrologic processes, reducing interception, infiltration and retention, favouring water to remain over the surface and move faster (Chow, Maidment, & Mays, Citation1998; Ogden, Pradhan, Downer, et al., Citation2011; Sheng & Wilson, Citation2009). Studies have already shown that LULC changes have significantly contributed to aggravate flood problems in several locations. Bahrawi, Ewea, Kamis, et al. (Citation2020) and Bayazit, Koç, and Bakiş (Citation2021) demonstrated significant increases of flood peaks, volumes and flooded extensions in both studies where the authors correlate LULC changes and flood records from recent decades in Jeddah, Saudi Arabia, and Bodrum Province, Turkey. Considering that urban population is likely to expand in the future (United Nations [UN], Citation2019; X. Q. Zhang, Citation2016), it is plausible to assume that cities are also prone to expand and densify (and face more critical floods). According to several studies, like the one conducted by G. Yang, Bowling, Cherkauer, et al. (Citation2011), flood responses significantly vary according to the urban configuration. Therefore, projections of potential LULC changes considering local aspects have emerged as key elements in flood risk modelling. Researches considering potential LULC changes, such as Du, Rompaey, Shi, et al. (Citation2015), Mustafa, Bruwier, Archambeau, et al. (Citation2018) and Nigussie and Altunkaynak (Citation2019), have demonstrated the importance of urbanization processes to flood risk, indicating significant differences of flood aspects when considering distinct scenarios of urban dynamics.
In the past recent years, climate change has received increasing attention as one of the most prominent factors influencing the occurrence of floods of significant severity. However, uncontrolled urbanization, urban sprawl and lack of infrastructure should be considered at least in the same level of concern, as several authors recognize that LULC changes have an enormous potential to intensify flooding. However, it seems that this awareness is not the same of that related to climate change consequences. Besides, the routine and procedures to maintain the existing urban drainage structures should be considered another key aspect capable of aggravating floods when they are not properly in place. Artificial channels and other drainage structures require regular maintenance to sustain their capability to receive and convey waters at adequate rates, as flood water commonly carry sediments, debris, organic matter and other solid materials which could reduce the effectiveness of urban drainage systems (Chow, Citation1959). Levees, for example, if submitted to structural failure or overflows, due to lack of maintenance and partial or total disruption, can create significant critical situations. Poor maintenance can lead to poor performance of flood protection structures during hazardous events, such as seen during and in the aftermath of Hurricane Katrina, for example, when several impacts could have been avoided if proper administration and oversight were implemented (Van Heerden, Citation2007). Uncertainty regarding future projections is another factor worth mentioning in flood risk management. Flood control structures of New Orleans, U.S.A, are a great example of how uncertainty is essential to guarantee the effectiveness of such strategies. After major failures of structures such as dikes and flood walls during Hurricane Katrina (Van Heerden, Citation2007), major investments were allocated to their reconstruction. Even though this is an important aspect to consider in flood risk modelling, this topic has scarcely been approached in the scientific literature. Besides, regular floods can be further magnified by poor maintenance of urban drainage systems. In this way, the behaviour of the drainage system is a third element to be considered in the discussion, since increasing natural demands, with climate changes affecting rainfall intensity, and running over highly modified watersheds and generating more floods, will have to find room and safe passage through adequate and functional drainage systems.
1.2. Aim and objectives
Therefore, this study aims to provide a framework to analyse future flood scenarios considering the effects of the three main drivers for flood aggravation previously discussed: climate change leading to rainfall intensification and mean sea level rise; unplanned urbanization leading to uncontrolled LULC changes critically modifying the urban water cycle; and poor maintenance of urban drainage systems hindering its functional responses. These drivers will be assessed individually and combined to enable the identification of their responsibility share in aggravating flooding problems. This result will bring light to the importance of each of these aspects, which is one of the main contributions of this research – applying the framework to prospect future responses will point to the critical possible problems to be faced and adequately guide efforts to reduce the main source of problems. Additionally, and complementing the proposed framework, a uchronic scenario will be built. Romano, Fiorini, and Marucci (Citation2019), in the context of urban development, classified uchronia as the scenario of an urban evolution from a rural state, in which projects and development plans guided land use and city occupation on a sustainable basis. This uchronic scenario will be built for comparison purposes, considering what could happen if the present situation could have evolved from past times in a more sustainable way, in terms of LULC concerns, mainly regarding urban rainwater dynamics. This last comparison intends to show that, even if climate changes threaten our future, problems will be fewer if we are aware of urban development responsibilities – which is a significant result, because ordering urban development is something that is objective, concrete and is in the decision makers hands. Besides, building the uchronic scenario allows to exercise the proposition and use of green and blue infrastructure concepts, intending to minimise conflicts between urban growth process and environmental carrying capacity, naturally increasing city resilience and showing a possible way out of the unsustainable development path.
An exploratory case study is set using the Acari River Basin, located in the city of Rio de Janeiro, Brazil, a coastal watershed densely urbanized where floods play a significant role, threatening and impoverishing the local population in a degrading vicious cycle. Urban drainage strategies have traditionally been based on hard engineering measures in this basin without showing effective results.
2. Case study
Most of the Metropolitan Region of Rio de Janeiro (MRRJ) is on flat floodplain areas. Extensive channelization has been used as the main flood mitigation strategy to drain these regions, seeking to allow safer urban occupation. After the accelerated urban growth experienced in the last century (Helders, Citation2005) and the consequent increase of runoff generation, most of these channels are no longer able to capture and convey rainwaters during intense rainfall events. Moreover, the rivers in the MRRJ outflow into the Guanabara Bay. At high tides, the backwater effect caused by water levels rise in the river mouth hinders water conveyance, reducing the outflow rates of coastal watersheds. These characteristics make this region very susceptible to climate change effects because the possible rise in mean sea level can be combined with possible increases in rainfall intensity aggravating flooding in this area.
The Acari River Basin is part of this reality. In addition, this basin covers an extensive area of 107 km2 and shelters a population of over one million inhabitants (Brazilian Institute of Geography and Statistics, Citation2010), being one of the largest and most densely occupied basins in the entire metropolitan region, with only 28.3% of open spaces still available (Oliveira, Citation2018) mainly in protected vegetated hills.
Social vulnerability is a problem in all 31 neighbourhoods located in the Acari River Basin, from which 25 are below the Rio de Janeiro city index average of the Social Development Index (Cavallieri & Lopes, Citation2008). In addition, the most susceptible area to flooding encompasses two of the ten most populous slums (known as ‘favelas’, in Portuguese) in the entire municipality, which are the Pedreira Complex and the Acari Complex, with more than 40,000 residents (Brazilian Institute of Geography and Statistics, Citation2010) living in precarious conditions and under recurrent effects of significant floods.
A previous study carried out for this area (Guimarães & Miguez, Citation2020) showed that a great part of the population living in the region is unable to recover from flood losses over a 50-year horizon, even when considering rainfall events of lower return periods (RP) (of 2 or 5 years, for example).
The lack of open spaces in the watershed also makes it difficult to implement solutions to support drainage system recovery. It is worth noting that most of these open spaces are hilly slopes located upstream of the watershed, inside environmental protection areas and far from the main watercourses, making it difficult to use them as reservoirs or fluvial parks. Even though these areas are usually unsuitable for urban development, the common trend perceived in Rio de Janeiro of occupying similar high-risk areas could lead to an expansion of the urbanization towards this natural landscape, which could contribute even more to the generation of runoff in the watershed and aggravate flooding. shows the location of the Acari River Basin, highlighting the main characteristics mentioned in this section. Furthermore, shows the current state of degradation of the rivers in the Acari River watershed, caused by the urbanization process and the lack of maintenance.
3. Materials and methods
The proposed method will be exposed in a step-by-step framework (). The method is supported by hydrodynamic mathematical simulation to measure the impacts of future scenarios on urban drainage in the study area.
In general lines, the following procedures compose the framework.
3.1. Characterization of the target urban area and basic diagnosis of the current situation
The first procedure follows the order presented in the following topics:
identification of the main watercourses;
landscape analysis (topography, natural areas of water storage);
land use analysis (formal urban areas, identification of environmental protected areas, identification of slums).
3.2. Proposed scenarios to explore future urban flooding responses
This work proposes scenarios that consider the system stressed under isolated and combined influences of adverse effects of: climate change; urban expansion; and irregular maintenance of the drainage system. These possibilities were simulated using the baseline scenario as reference. The baseline scenario represents the current situation and is also the one adopted for the hydrodynamic model calibration using real observed data.
Additionally, a uchronic scenario was developed in parallel, with the aim of raising the discussion about the carrying capacity of the natural drainage systems and possible approaches to face this issue. In this case, the natural system was used as the main conveyor of the urban water (therefore, composing the formal urban drainage system), in an alternative reality based on a city growth path that would have respected the riverine areas and the valley bottom as natural storage areas, giving space to the flood plains. Elements of green and blue infrastructure appear in this uchronic scenario.
The paper puts forward a uchronic reconstruction of the physiognomy of the case study area, in a process similar to what was suggested by Romano, Fiorini, and Marucci (Citation2019), focusing on adequately managing urban pluvial water instead of assuming the real and weak urban development policies implemented over the last decades. In this case, the urbanization process favoured urban compacity and protected the territory landscape and environment against urban sprawl. In this uchronia, urban planning also considered natural environmental needs and the importance of the ecosystem service related to flood damping.
3.3. Stressing drivers for analysing the future
In this work, the possible drainage system stressors will consider three main drivers evolving in time. They are urban growth, climate change, and a lack of maintenance of the drainage network. These drivers can affect urban flooding responses, causing significant losses and degrading the city over time.
All the proposed scenarios, including the uchronic scenario will be stressed according to these drivers and compared with the baseline scenario, so that lessons can be learned and the relative importance of the proposed stressors can be investigated.
In the following topics, the methods adopted for each simulation driver will be described. The construction of these scenarios is schematically represented in , showing their combinations. All scenarios will consider a time span of 25 years.
3.3.1. Urban expansion
To simulate the expansion of the urban areas, the occupation of the remaining free areas of the watershed will be considered, as well as the densification of the already occupied areas. This process will simulate a non-controlled or disordered urban growth. A runoff coefficient increase was adopted for the entire urban area of the watershed to accomplish this purpose. This number was based on the highest runoff observed within the catchment area in the baseline scenario – that is, the most dense existing area, already in a consolidated shape, was taken as reference to infer the future situation of a disordered occupation spread over the watershed. In the uchronic scenario, population growth is absorbed by urban densification, considering the vertical expansion of the city, while urban sprawl is no longer allowed. In this way, urbanization does not change the runoff coefficient in the uchronic scenario, because it is considered that there is no increase in the imperviousness of the watershed.
3.3.2. Lack of maintenance of the drainage system
The maintenance status of the drainage system is being represented by the cleaning and silting conditions of the main rivers and channels in the study area. An increase of the Manning Coefficient in the main watercourses was adopted to represent the degradation of the urban drainage system maintenance conditions – this increase is based on Chow (Citation1959), in a scenario that considers the worsening of the degree of irregularity, clogging effect by solid sediments and inappropriate vegetation growth in the channels. Moreover, it should be remembered that the adopted model does not represent the watercourses as movable bed channels; therefore, silting represents a partial obstruction to the flow in the channels, which is represented by a higher coefficient of rugosity. Departing from the baseline scenario, it will be assumed that the maintenance of channels, in general, will not be carried out, while in the uchronic scenario, more natural fluvial system demands less interventions and maintenance is in place. Therefore, in the uchronic scenario, maintenance does not change the Manning Coefficient because it is considered that a periodic maintenance occurs and holds the hydraulic capacity of the channels.
3.3.3. Effects of climate change
The effects of climate change were represented by an increase in rainfall intensity and a rise in the mean sea level. Most studies aiming to prospect future climatic change in the city of Rio de Janeiro indicate that rainfall events will most likely intensify, even though they also often show that total annual rainfall is most likely to decrease (Barata, Confalonieri, Marinho, et al., Citation2014; Dereczynski, Silva, & Marengo, Citation2013; Zilli, Carvalho, & Silva, Citation2016). Thus, this study considers rainfall intensification as a major consequence of climate change, which is represented by a percentual increase of the design rainfall. The strategy applied to the sea level rise was the adoption of a conservative value for sea level rise based on models developed by IPCC (Citation2022).
The final set of simulation scenarios is listed in the sequence:
Baseline Scenario – Current Situation and model calibration;
Uchronic Scenario – Idealized scenario considering that urban growth took place in an orderly manner, without advancing over the natural fluvial spaces or vegetated hills, and watercourses’ maintenance was kept up to date. This scenario evolved from a past moment in time to a current situation of a preserved valley bottom where green fluvial park areas were defined;
U25 Scenario – Scenario of disordered urban occupation of the watershed, projecting an urban sprawl that reproduces the densest sector of the watershed in current days. ‘U’ represents the disordered Urban occupation, and ‘25’ indicates 25 years in the future;
M25 Scenario – Scenario representing the same situation found today in term of urban occupation, but accumulating the lack of maintenance of the urban drainage system along the years ‘M’ represents the lack of Maintenance of the urban drainage;
U25M25 Scenario – Sum of U25 and M25 scenarios;
U25 Uchronic Scenario – future urbanization of the Uchronic scenario with the population growing through the verticalization of buildings in the study area (without increasing the imperviousness of the watershed area);
M25 Uchronic Scenario – Uchronic scenario with proper maintenance of drainage system over time;
U25M25 Uchronic Scenario – Sum of U25 and M25 uchronic scenarios;
Climate Change Scenarios – increase of 20% in the rainfall event, combined with a mean sea level rise of 0.30 m, in the horizon of 25 years, applied to all previous scenarios. Scenarios considering climate change will receive the suffix C25. The values presented were chosen within the uncertainty range of the Intergovernmental Panel on Climate Change (IPCC, Citation2022).
3.4. Hydrodynamic mathematical modeling
Hydrologic and hydrodynamic modelling is used as a supporting tool to model floods representing the current situation, where a calibration process is conducted to simulate the spatial behaviour of possible future scenarios, offering a predicting tool to assess flood maps and overflow volumes of the main river channels.
The modelling process considers the following the steps:
model calibration;
diagnosis of the water dynamics and flooding processes in the interest area, considering a design rainfall related to a return period of 25 years (which is the design rainfall reference officially adopted in Brazil);
analysis of the main channel overflow volumes in all the previous defined scenarios.
3.4.1. Urban flood cell model (MODCEL)
MODCEL is a hydrodynamic model that is also capable of representing rainfall-runoff transformation process. It calculates the watershed responses in terms of superficial flows, storm drains and river and channels flows. This model is free and can be considered as a quasi-2D model that simulates urban floods over a complex flow network representing river channels and floodplains, as well as streets and urban storm drains using one-dimensional connections (Sousa, Oliveira, Rezende, et al., Citation2022).
The model interprets watersheds as a set of interconnected compartments called flow-cells, representing the general aspects of the area. Each cell encompasses a set of information such as slope, LULC, total area, storage area, runoff coefficient and others.
The separation of surface runoff in MODCEL uses the runoff coefficient, defined according to the LULC for each cell. The LULC definition for the Acari River watershed was made by visual interpretation of satellite images of the region.
The hydraulic laws defined between the cells are one-dimensional. However, the system can represent a 2-D surface response on an irregular spatial mesh. Therefore, the division of the floodplain into cells does not use homogeneous elements – the cells can vary in shape and size, adapting themselves to represent portions of the modelled area. Nevertheless, it is not an arbitrary process because it uses natural or constructed boundaries such as elevated roads, dikes, walls, buildings, and other natural or built structures which introduce local particularities to the flow patterns.
Considering these aspects, single cells or groups of cells can adequately represent water dynamics that can occur in natural landscapes, urban environment and rivers, simulating the behaviour of floodwaters passing through cities. The continuity equation is applied to each cell and the water flowing between cells is represented through well-established hydraulic laws, such as the Saint-Venant dynamic equation to represent cells connections in rivers and canals and also for street flows. Other possibilities include broad crested weirs, orifice, pumps, flap gates, etc.
Other studies using MODCEL were developed by Scionti, Miguez, Barbaro, et al. (Citation2018), Oliveira, Rezende, Sousa, et al. (Citation2019, Citation2022), Lourenço, Oliveira, Marques, et al. (Citation2020), Lourenço, Guimarães, Alves, et al. (Citation2020), Gomes, Battemarco, Guimarães, et al. (Citation2021), Radesca, Sousa, Veról, et al. (Citation2021), Guimarães, Battemarco, Oliveira, et al. (Citation2021), Battemarco, Tardin-Coelho, Veról, et al. (Citation2022) and Sousa, Oliveira, Rezende, et al. (Citation2022). A thorough description of MODCEL is available in Miguez et al. (Citation2017).
4. Model calibration
The model calibration was done by comparing the simulated results and water levels recorded in the event of 11 December 2013, which was a significant rainfall event recorded in the recent past.
There are four gauges in the watershed capable of measuring rainfall and water levels. Two gauges are located on tributaries of the main river (Catarino River and Sapopemba River) in the upstream reaches of the basin, one gauge is in the Acari River near its mouth, and one gauge is in the Meriti River (which is the river that receives the Acari River and has the Guanabara Bay as outlet).
Analysing the rainfall events, considering the time of concentration of the Acari River watershed (about 5 hours) and using the intensity – duration–frequency equations of the municipality of Rio de Janeiro for the watershed, it was possible to estimate the Return Period (RP) at each station for the 2013 calibration event. The rainfall measured in the Catarino River indicated an RP of 20 years (109 mm in 5 hours), in the Sapopemba River an RP of 33 years (119 mm in 5 hours), in the Acari River an RP of 150 years (150 mm in 5 hours), and in the Meriti River an RP of less than one year (8 mm in 5 hours). Most of the watershed was affected by a rainfall with RP between 20 and 30 years, values close to the RP recommended for river drainage projects in Brazil (25 years), validating this event as the calibration reference.
Furthermore, the aftermath of this event exhibited devastating outcomes, with several locations of the watershed experiencing high water levels, consequently damaging properties and disrupting services. Flood records, related to inundated portions of the watershed for this specific event were also made available by Fundação Rio-Águas, the public entity responsible for flood management in the city of Rio de Janeiro. The main calibration results are shown in . The representation of the water levels in the Meriti River were equivalent to the tidal variation in the Guanabara Bay, obtained from levels recorded by the Brazilian Navy.
The results show maximum simulated errors in the water levels of 0.09%, −0.65%, 0.24, and −3.58% for the river gauges of Catarino, Sapopemba, Acari, and Meriti, respectively. The calibration process is essential to reduce the uncertainty of the results inherent to the input of model coefficients (Luo, Luo, Li, et al., Citation2022). According to Teng, Jakeman, Vaze, et al. (Citation2017) this error is one of the most relevant uncertainties generated in the results of hydrodynamic simulations.
Water levels on the remaining areas of the watershed, where measurements were not officially recorded, were derived from a survey of newspapers, websites and social media posts displaying local outcomes of the rainfall event. This type of investigative calibration, when done correctly, can bypass the lack of official data for the mathematical model calibration and validation process (Oliveira, Rezende, de Sousa, et al., Citation2019).
To obtain the results presented, the watershed coefficients underwent a calibration process to adjust the total volume of rainfall that becomes surface runoff – this hydrological adjustment was the first step. Additionally, there was an adjustment of the Manning coefficients for the Saint-Venant connections of the river cells, as well as the other hydraulic coefficients, like the weir coefficients that represented river overflows to the lateral streets.
The runoff coefficients obtained for the calibration indicate that 7% of the watershed has runoff coefficients up to 0.25. These values indicate vegetated areas upstream of the watershed. In addition, 24% of the watershed has coefficients between 0.25 and 0.50, representing peri urban areas and low-density urbanization. The range with values between 0.50 and 0.75 occupies 43% of the watershed and characterizes urban areas of medium to high density. The remaining 26% of the watershed make up regions with a runoff coefficient greater than 0.75 and represent urban areas with very high density, wetlands, and water. Regarding Manning’s coefficients, 8% of the watercourses have values up to 0.025, 71% have values between 0.025 and 0.050, and 21% have values above 0.050. The cited Manning coefficients used the publication set by Chow (Citation1959) and presented in as the starting point for the process.
Table 1. Manning coefficient base values and adjustments based on the channel condition.
4.1. Hydrodynamic and hydrological parameters for the stressing drivers and uchronic scenario
The calibrated hydrodynamic-hydrological model assumed several changes to simulate the stressing drivers. The urban expansion scenario considered an increase in the runoff coefficient proportional to the watershed population increase in a biased scenario and without urban occupation control in the next 25 years. For this scenario, 66% of the basin assumed a runoff coefficient from 0.50 to 0.75, and 34% has a runoff coefficient greater than 0.75.
The lack of maintenance scenario for the next 25 years considered an increase in the Manning coefficient because of the degree of irregularity caused by the accumulation of sediments at the bottom of rivers and channels, the obstruction effects caused by solid waste and vegetation growth (which mainly affects the upper river flows). Thus, 100% of the watercourses assumed a Manning coefficient greater than 0.050.
The climate change scenario had a 20% increase in total design rainfall. Neither runoff nor Manning coefficients have changed. The sea level rise was directly incorporated into Guanabara Bay boundary condition. This way, the water level in the Bay received another 30 centimetres, according to the sea level rise trends indicated by the IPCC (Citation2022).
In the future uchronic scenario (25 years ahead), despite the population growth, the runoff coefficients reproduce the current situation, which is possible by verticalization policies. Furthermore, the Manning coefficients do not increase over time as recurrent maintenance policies are encouraged. Additionally, policies to restore the spaces of rivers and channels were encouraged, and the use of green and blue infrastructure through the incorporation of fluvial parks is part of the reality of the basin for this scenario. Fluvial parks entered the simulation process as riverine cells capable of receiving overflow volumes on rainy days, protecting occupied urban regions.
5. Results
5.1. Design rainfall and rainfall intensification
The design rainfall chosen represents an event of 25 years of Return Period (RP) with a duration of 5 hours, which corresponds to the time of concentration of the watershed. The rain gauges of Irajá, Realengo and Bangu were considered to account for possible spatial variations of rainfall patterns. An Intensity-Duration-Frequency (IDF) equation is available for each rain gauge (Águas, Citation2010), allowing the calculation of a specific design rainfall falling over areas of influence of each gauge, defined by the Thiessen Polygons method. Additionally, temporal distribution for each design rainfall is built using the alternating block method. The Equation 2, Equation 3, and Equation 4 represent the IDF curves for the considered gauges.
Where: i – rainfall intensity (mm/h); RP – Return Period (years); t – duration of rainfall (minutes).
5.2. Flooded areas
The hydrodynamic simulations allowed to confirm the criticality of the floods in the watershed in the baseline scenario. The most critical region is settled to the northeast of the watershed, near its river mouth. As shown in , it is evident that future stress scenarios significantly worsen the already critical local floods, especially in combined scenarios. On the other hand, the uchronic scenario responds better to the design rainfall and is more resilient in the face of the climate change. Residual flooding in the uchronic scenario is related to the lack of representation of minor drainage networks (not included in the current modelling). It is worth noting that there is no river overflows in the uchronic scenario.
5.3. Overflow volumes
exhibits the summary of the main results of this study. The model estimates a total water overflow of 5.63 × 106 m3 for the Baseline scenario. Considering the scenarios without climate change effects on rainfall intensification, unplanned urbanization favours the overflow of higher volumes of water from the main channel of the Acari River. Whilst the M25 scenario demonstrates a 28.0% increase of overflow volumes when comparing to the Baseline scenario (+1.57 × 106 m3), the U25 scenario generates an increase of 90.0% (+5.07 × 106 m3). The U25M25 scenario demonstrates that the combination of unplanned urbanization and poor maintenance of urban drainage structures results in a total overflow volume of 18.92 × 106 m3, representing an increase of 236.1% when comparing with the current situation of the watershed.
When considering rainfall intensification due to climate change, the Baseline scenario demonstrates that the overflow volume increases by 39.2% (+2.21 × 106 m3). U25C25 shows an increase of 140.2% (+2.94 × 106 m3), while M25C25 exhibits an increase of 148.3% (+6.77 × 106 m3). U25M25C25 demonstrates an increase of 315.2%, reaching a total water overflow volume of 23.38 × 106 m3. It is noticeable that climate change can turn the overall situation even more critical. However, when comparing the Baseline scenario modified by climate change (Baseline + C25 = 7.84 × 106 m3) with the same Baseline scenario modified by uncontrolled urban expansion (U25 = 10.70 × 106 m3), the latter is approximately 36.5% worse than the former. That is, a precarious urban growth, not adequately planned or controlled can be more dramatic, in terms of urban flooding, than climate change.
On the other hand and confirming the importance of an adequately conducted urban growth process, the scenario considering the uchronic concept demonstrates significant reductions of total overflow volumes when compared to the other scenarios, including the Baseline. For the uchronic scenarios, it was necessary to zoom in on the results presented in to visualize the overflow volumes. Considering the design rainfall with no effects of climate change, the uchronic scenario results in a total water overflow volume of 0.04 × 106 m3, representing a reduction of 99.3% when compared to the Baseline scenario. When considering the effects of climate change on the uchronic scenario, reductions remain significant, as the total water overflow volume reaches 0.22 × 106 m3. When comparing to the Baseline scenario modified by climate change, this final value still represents a reduction of 96.1%. These results show that controlling urban growth and introducing green and blue concepts in urban development can effectively increase city resilience to floods.
6. Discussion
Even though the Acari River Basin is already extensively urbanized, land use and land cover changes due to unplanned urbanization in the remaining open spaces along with the densification of the existing built environment (Scenario U25) contributes to higher overflow volumes than any other result of the individual Stressing Drivers. Therefore, it is noticeable that the availability of open spaces, their interactions with water dynamics, offering storage volumes to the drainage system, and the maintenance of green buffers are contributing factors to reduce the total volume of water reaching floodplains during significant rainfall events. This finding further validates the results from studies such as Lourenço, Oliveira, Marques, et al. (Citation2020), where the authors demonstrate significant reductions of flood extensions when sustainable urban planning is prioritized.
Avoiding urban sprawl and incentivizing urban compacity are also highlighted as practices that contribute to maintain floods under control. This is an important result because it shows how urban growth can act negatively in city flood responses. Although climate change is very challenging theme, incorporating green and blue infrastructure approaches into urban development could represent the best strategy to pave the way to sustainable and flood resilient cities, mitigating flood risks from rainfall events of the current climate and from potentially worse precipitation due to the changing climate. It is important to note that urban planning is in the sphere of responsibility of the Municipalities, while climate change mitigation agreements bring largely diffused responsibilities.
The results also show larger proportional increases of total overflow volumes in the M25C25 than in U25C25, demonstrating that the area would potentially face relatively larger floods when jointly considering climate change scenarios with urban related stressors. Moreover, and somewhat unexpectedly (mainly due to the lack of publications and discussions on the subject in academic and professional environments), flood aggravation is much more intense when climate change is combined with a poorly functional urban drainage system. This fact represents an interesting finding, which can and should be further explored in future studies, showing that poorly maintained systems will suffer the most with rainfall variation. When closely looking to these results, it is perceivable that increasing flood volumes combined with restricted drainage systems is really a significant threat.
In general, it is expected that a poorly planned urban expansion will be accompanied by insufficient infrastructure and poor maintenance, which can be a time bomb in urban development.
On the other hand, when looking backwards and analysing the simulation results of the uchronic scenario, they also confirm the importance of land use and occupation for the drainage system and city resilience to floods. The hypothetic sustainable and resilient development of the urban area in the watershed (friendly interface between city and pluvial waters) is capable of significantly reducing flooding consequences. The simulations also show that a city that is seriously respecting ‘water spaces’ also reaches high resilience to climate change. Even for the C25 Uchronic Scenario, floods remain small when compared to the Baseline Scenario.
However, implementing more resilient flood measures is not yet a global reality. After an extensive analysis of works on the theme, He and Cha (Citation2021) highlight that most of the initiatives addressing disaster resilience planning are still in the research and development stage. This reality is due to the difficulty of making these initiatives a priority in municipalities. Moreover, public concern tends to deem climate change as the sole responsible for recurrent flood related issues in cities worldwide, with little consideration on how urban configuration has an essential role in flood mitigation. This is a common scenario in developing countries, as exemplified in Abass, Buor, Afriyie, et al. (Citation2020), where the authors highlight a lack of urban planning strategies regarding land use, as green open spaces inside cities are rapidly occupied, and there is a consistent trend to blame climate change due to constant and increasing flood problems. Therefore, the results found in our study can also assume an educational character, acting as a convincing argument that even though climate change should not be ignored, there are imperative topics not receiving the attention they really deserve. It is evident that urban related stressors pose greater significance in the case explored in this study than an increase in rainfall intensity, as both scenarios considering urban related stressors presented greater overflow volumes than that of the scenario considering only climate change effects.
In general, these results agree with similar studies indicating that unplanned urbanization is a major contributor to flood intensification (Bahrawi, Ewea, Kamis, et al., Citation2020; Bayazit, Koç, & Bakiş, Citation2021; G. Yang, Bowling, Cherkauer, et al., Citation2011; Lourenço, Oliveira, Marques, et al., Citation2020; Mustafa, Bruwier, Archambeau, et al., Citation2018), although climate change largely occupies the collective imaginary as the greatest antagonist of urban agglomerations.
As an additional reasoning, it is important to dedicate some attention to the uncertainties that are attached to our framework. We assumed an urban growth related to the capacity of the watershed to shelter a population increase driven by the maximum uncontrolled sprawl. In the same way, we used projections of the IPPC that can change (and aggravate over time). The maintenance gaps were also estimated by looking to previous experiences. Finally, the mathematical modelling process brings uncertainties by its own. Therefore, although the proposed urban growth is possible as well as the lack of drainage system maintenance is (once they reflect something already observed in parts of the watershed), the projections of IPCC are probably the most accepted in the climate change discussion, and the model calibration brings confidence, it is important to highlight that the relative numbers are more important and more reliable than the absolute ones. However, when dealing with resilience discussion, uncertainties are usually present. More than being a problem on their own, it is possible to converse this aspect in an opportunity. If different considerations for the urban development limits (from more sustainable to more unsustainable) and climate change responses (from optimistic to pessimist forecasts) are defined and combined, it could be possible to build a range of flood response behaviours, encompassing the main uncertainties. With these results, critical zones and safe zones could be clearly identified, while uncertainty zones related to possibly floodable areas could be targeted to receive resilient actions. In this sense, critical areas should be avoided in the urban expansion, mitigations actions should be considered to protect the already installed urban areas and, if possible, people living there could be relocated. On the other way, safe areas could be addressed as urban densification areas, but preserving water dynamics needs to avoid aggravating flooding downstream. At last, uncertainty areas could be occupied with proper construction guidelines, recognizing the flood risks and usingCitation2019 flood proof buildings, defining safe routes and controlled flooding areas, and other similar possibilities.
7. Conclusions
A simulation framework was proposed to analyse future city flooding scenarios subjected to different stressing factors, aiming to respond which of these factors is the most significant, intending to guide planning efforts to the right place. This information is one of the contributions of this work and can guide urban planning decisions towards more functional cities in terms of urban flooding.
The proposed method makes it possible to individually assess the effects of three stressing drivers acting on the drainage system over the time: climate change; disordered urbanization; and poor maintenance of urban drainage systems. These stressing drivers were also combined, escalating their effects. Additionally, a novel approach was introduced to contrast these stressors with an ideal urban evolution. A uchronic scenario was built to assess the positive impacts of a hypothetical and idealized alternative urban development. The differences between this scenario and the real baseline scenario can show the lost opportunities, bring important lessons and act as a practical example for the areas still under urban development. When introducing the uchronic scenario in this framework, building a parallel reality based on green and blue infrastructure approach, another contribution to knowledge is made, showing a possible path for flood resilient cities. Comparatively, the simulated uchronic scenarios demonstrate the severe degradation caused by years of low territorial occupation control.
The framework proposed in this research was applied in an exploratory case study that was developed to the Acari River basin, in the city of Rio de Janeiro, considering a 25-year horizon. This is an already dense urban area subjected to heavy flood effects.
Simulating the stressed future scenarios, the disordered urban occupation stands out negatively, showing the worst individual results related to increasing floods, but it is the scenario of lack of maintenance that presents least resilience to climate change, showing the greatest percentual increase. It is noteworthy that the results presented in this work refer to a watershed that is already extensively urbanized. This is a significant aspect, as in this case, disordered urban growth plays just an incremental role and this stressor may be even more important when disordered development occurs in sparse urban occupation.
Climate change effects showed a potential to aggravate the situation in all scenarios, but when considered alone in this case study, it was not so critical as the other stressors were. However, it is important to note that the results may likely differ in cases of watersheds under different development conditions (see, for instance the results of the uchronic scenario) or different physical aspects (topographic, hydrographic, hydrologic etc.) In this way, the method presented in the work has the potential to be replicated in different case studies, and different results are expected for each urban watershed specificity. The replication of this framework to other cases may create an opportunity to produce a useful knowledge basis for urban planning. The most positive aspect in this discussion is that urbanization is a process that can be controlled if urban planners and managers assume their responsibilities. Nonetheless, it is also necessary to highlight that climate change can (and will) stress the existing systems in a significant way. The results also demonstrate the importance to consider the maintenance of urban drainage structures as another primary aspect acting to favour flood alleviation, which is a factor that has been scarcely approached in the scientific literature and should be further explored for a better understanding of this underestimated factor.
In future research steps, variations in the urban future scenarios and climate change effects should be evaluated to assess the sensibility of the results produced, possibly providing a range of flood response behaviours, both to gain more knowledge on the process and better supporting urban planning decisions.
Additionally, two other urban watersheds will be modelled to increase the representativity of the proposed framework: the first one responds for an already consolidated urban neighbourhood, with formal settlements and adequate infrastructure (contrasting with Acari river basin, where informal settlements and precarious urban services co-exist); and an urban expansion watershed, where urbanization is still rarefied and there are still several free open green spaces (in which the uchronic scenario will not be necessary, since future sustainable scenarios can still be proposed). These results will help to better understand the cross-behaviour of the adopted stressors and the possible synergies with different initial urban occupations.
Acknowledgments
This study was conducted with support from Conselho Nacional de Desenvolvimento Científico e Tecnológico (CNPq), Processes 142284/2018-1 and 303862/2020-3. Special thanks go to the UNESCO Chair for Urban Drainage in Regions of Coastal Lowlands at Federal University of Rio de Janeiro.
Disclosure statement
No potential conflict of interest was reported by the author(s).
Data availability statement
The data that support the findings of the study are available from the corresponding author, Antonio Krishnamurti Beleño de Oliveira, upon reasonable request.
Additional information
Funding
Notes on contributors
Antonio Krishnamurti Beleño de Oliveira
Antonio Krishnamurti Beleño de Oliveira Civil Engineer from the Universidade Federal do Rio de Janeiro (UFRJ) (2016), MSc (2018) and DSc (2022) at the Instituto Alberto Luiz Coimbra de Pós-Graduação e Pesquisa de Engenharia / UFRJ. Professor at the Pontifícia Universidade Católica do Rio de Janeiro and at the Instituto Niemeyer (INPUC).
Lucas Magalhães Carneiro Alves
Lucas Magalhães Carneiro Alves Environmental Engineer from Universidade Federal Fluminense (UFF), with exchange studies at The University of Queensland, Australia, through the Science Without Boarders governmental program. Has experience in environmental legislation and monitoring and in water supply in rural communities. Currently studying for a MSc in Universidade Federal do Rio de Janeiro (UFRJ).
Carolina Lopes Carvalho
Carolina Lopes Carvalho Architect and Urban Planner graduated from the Pontifícia Universidade Católica do Rio de Janeiro (2018). She is currently studying for a MSc in Escola Politécnica da Universidade Federal do Rio de Janeiro (POLI/UFRJ). Specialist in Planning, Control and Management of Civil Works at POLI/UFRJ.
Assed Naked Haddad
Assed Naked Haddad Civil Engineer from the Universidade Federal do Rio de Janeiro (UFRJ) (1986) and MSc from the UFRJ (1992). PhD in Production Engineering from UFRJ (1996) with a Postdoctoral degree from three universities. Professor at the UFRJ and visiting professor at the University of Florida, and at Western Sydney University.
Paulo Canedo de Magalhães
Paulo Canedo de Magalhães Civil Engineering from the Universidade Federal do Rio de Janeiro (UFRJ) (1972), a MSc in Civil Engineering from the UFRJ (1974) and a Ph.D. from Lancaster University (1979). He has been a professor and researcher at the UFRJ since 1974. He is an international consultant at the World Bank.
Marcelo Gomes Miguez
Marcelo Gomes Miguez Civil Engineer and Professor of the Universidade Federal do Rio de Janeiro and responsible for the UNESCO Chair for Urban Drainage in Regions of Coastal Lowlands. Author of the hydrodynamic computational model called MODCEL, he mainly works with flood risks and integrated projects for resilient cities.
References
- Abass, K., Buor, D., Afriyie, K., Dumedah, G., Segbefi, A. Y., Guodaar, L., Garsonu, E. K., Adu-Gyamfi, S., Forkuor, D., Ofosu, A., Mohammed, A., & Gyasi, R. M. (2020). Urban sprawl and green space depletion: Implications for flood incidence in Kumasi, Ghana. International Journal of Disaster Risk Reduction, 51(March), 101915. https://doi.org/10.1016/j.ijdrr.2020.101915
- Adnan, M. S. G., Abdullah, A. Y. M., Dewan, A., & Hall, J. W. (2020). The effects of changing land use and flood hazard on poverty in coastal Bangladesh. Land Use Policy, 99, 104868. https://doi.org/10.1016/j.landusepol.2020.104868
- Avand, M., Moradi, H. R., & Ramazanzadeh Lasboyee, M. (2021). Spatial prediction of future flood risk: An approach to the effects of climate change. Geosciences, 11(1), 25. https://doi.org/10.3390/geosciences11010025
- Bahrawi, J., Ewea, H., Kamis, A., & Elhag, M. (2020). Potential flood risk due to urbanization expansion in arid environments, Saudi Arabia. Natural Hazards, 104(1), 795–809. https://doi.org/10.1007/s11069-020-04190-7
- Barata, M. M. L., Confalonieri, U. E. C., Marinho, D. P., Neto, C. C., Luigi, G., Silvia, H. V. O., Tosta, F. O., Pereira, C. A. R., & Marincola, F. C. V. (2014). Vulnerability map of the population of municipalities in the state of Rio de Janeiro to climate change [In Portuguese]. Fundação Oswaldo Cruz (Fiocruz). http://www.fiocruz.br/ioc/media/20150722_Relatorio_Final_RJ.pdf
- Battemarco, B. P., Tardin-Coelho, R., Veról, A. P., de Sousa, M. M., da Fontoura, C. V. T., Figueiredo-Cunha, J., Barbedo, J. M. R., & Miguez, M. G. (2022). Water dynamics and blue-green infrastructure (BGI): Towards risk management and strategic spatial planning guidelines. Journal of Cleaner Production, 333, 129993. https://doi.org/10.1016/j.jclepro.2021.129993
- Bayazit, Y., Koç, C., & Bakiş, R. (2021). Urbanization impacts on flash urban floods in Bodrum Province, Turkey. Hydrological Sciences Journal, 66(1), 118–133. https://doi.org/10.1080/02626667.2020.1851031
- Brandt, S. A., Lim, N. J., Colding, J., & Barthel, S. (2021). Mapping flood risk uncertainty zones in support of urban resilience planning. Urban Planning, 6(3), 258–271. https://doi.org/10.17645/up.v6i3.4073
- Brêda, J. P. L. F., Paiva, R. C. D., Collischon, W., Bravo, J. M., Siqueira, V. A., & Steinke, E. B. (2020). Climate change impacts on South American water balance from a continental-scale hydrological model driven by CMIP5 projections. Climatic Change, 159(4), 503–522. https://doi.org/10.1007/s10584-020-02667-9
- Cavallieri, F., & Lopes, G. P. (2008). Social Development Index-SDI: Comparing the micro-urban realities of the city of Rio de Janeiro [in Portuguese]. Coleção Estudos Cariocas, 20080401. https://portalgeo.rio.rj.gov.br/estudoscariocas/download/2394_%C3%8Dndice%20de%20Desenvolvimento%20Social_IDS.pdf
- Chow, V. T. (1959). Open-channel hydraulics. McGraw-Hill Book Company.
- Chow, V. T., Maidment, D. R., & Mays, L. W. (1998). Applied hydrology. McGraw-Hill Book Company.
- CRED & UNDRR. (2020). The human cost of disasters: An overview of the last 20 years (2000-2019). Centre for Research on the Epidemiology of Disasters and UN Office for Disaster Risk Reduction. https://www.emdat.be/publications
- Dereczynski, C., Silva, W. L., & Marengo, J. (2013). Detection and projections of climate change in Rio de Janeiro, Brazil. American Journal of Climate Change, 2(1), 25–33. https://doi.org/10.4236/ajcc.2013.21003
- Dos Santos, D. J., Pedra, G. U., Silva, M. G. B., Júnior, C. A. G., Alves, L. M., Sampaio, G., & Marengo, J. A. (2020). Future rainfall and temperature changes in Brazil under global warming levels of 1.5°C, 2°C and 4°C. Sustainability in Debate, 11(3), 57–73. https://doi.org/10.18472/SustDeb.v11n3.2020.33933
- Du, S., Rompaey, A. V., Shi, P., & Wang, J. (2015). A dual effect of urban expansion on flood risk in the Pearl River Delta (China) revealed by land-use scenarios and direct runoff simulation. Natural Hazards, 77(1), 111–128. https://doi.org/10.1007/s11069-014-1583-8
- Fletcher, T. D., Shuster, W., Hunt, W. F., Ashley, R., Butler, D., Arthur, S., Trowsdale, S., Barraud, S., Semadeni Davies, A., Bertrand-Krajewski, J. L., Mikkelsen, P. S., Rivard, G., Uhl, M., Dagenais, D., & Viklander, M. (2015). SUDS, LID, BMPs, WSUD and more – the evolution and application of terminology surrounding urban drainage. Urban Water Journal, 12(7), 525–542. https://doi.org/10.1080/1573062X.2014.916314
- Garner, A. J., Weiss, J. L., Parris, A., Kopp, R. E., Horton, R. N., Overpeck, J. T., & Horton, B. P. (2018). Evolution of 21st century sea level rise projections. Earth’s Future, 6(11), 1603–1615. https://doi.org/10.1029/2018EF000991
- Gasser, P., Lustenberger, P., Cinelli, M., Kim, W., Spada, M., Burgherr, P., Hirschberg, S., Stojadinovic, B., & Sun, T. Y. (2019). A review on resilience assessment of energy systems. Sustainable and Resilient Infrastructure, 1–27. https://doi.org/10.1080/23789689.2019.1610600
- Gomes, M. V. R., Battemarco, B. P., Guimarães, L. F., Oliveira, A. K. B. D., Rutigliani, V. D. A., Cabral, F. M., Bezerra, R. D. O. P., Lourenço, I. B., Rezende, O. M., Magalhães, P. C. D., Miguez, M. G., & Veról, A. P. (2021). The use of blue-green infrastructure as a multifunctional approach to watersheds with socio-environmental vulnerability. Blue-Green Systems, 3(1), 281–297. https://doi.org/10.2166/bgs.2021.119
- Gomes Miguez, M., Peres Battemarco, B., Martins De Sousa, M., Moura Rezende, O., Pires Veról, A., & Gusmaroli, G. (2017). Urban Flood Simulation Using MODCEL—An Alternative Quasi-2D Conceptual Model. Water, 9(6), 445. https://doi.org/10.3390/w9060445
- Guimarães, L., Battemarco, B., Oliveira, A., & Miguez, M. (2021). A new approach to assess cascading effects of urban floods. Energy Reports, 7, 8357–8367. https://doi.org/10.1016/j.egyr.2021.07.047
- Guimarães, L. F., & Miguez, M. G. (2020). Supporting decision-making on urban flood control alternatives through a recovery deficit procedure for successive events. Journal of Flood Risk Management, 13(2), e12596. https://doi.org/10.1111/jfr3.12596
- He, X., & Cha, E. J. (2021). State of the research on disaster risk management of interdependent infrastructure systems for community resilience planning. Sustainable and Resilient Infrastructure, 7(5), 1–30. https://doi.org/10.1080/23789689.2020.1871541
- Helders, S. (2005). World gazetteer. World Gazetteer.
- IBGE - Brazilian Institute of Geography and Statistics. (2010). Census [In Portuguese]. https://www.ibge.gov.br/
- Imbach, P., Chou, S. C., Lyra, A., Rodrigues, D., Rodriguez, D., Latinovic, D., Siqueira, G., Silva, A., Garofolo, L., & Georgiou, S. (2018). Future climate change scenarios in Central America at high spatial resolution. Plos One, 13(4), 1–21. https://doi.org/10.1371/journal.pone.0193570
- IPCC. (2022). Climate change 2022: Impacts, adaptation and vulnerability. Contribution of working group II to the sixth assessment report of the intergovernmental panel on climate change. (H.-O. Pörtner, D. C. Roberts, M. Tignor, E. S. Poloczanska, K. Mintenbeck, A. Alegría, M. Craig, S. Langsdorf, S. Löschke, V. Möller, A. Okem, B. Rama, Eds.). Cambridge University Press. https://doi.org/10.1017/9781009325844
- Jackson, S. (2015). Overview of resilience and theme issue on the resilience of systems. Insight, 18(1), 7–9. https://doi.org/10.1002/inst.12001
- Jackson, L. P., Grinsted, A., & Jevrejeva, S. (2018). 21st century sea-level rise in line with the Paris Accord. Earth’s Future, 6(2), 213–229. https://doi.org/10.1002/2017EF000688
- Johnson, A. (2013). Green infrastructure: A new strategy for stormwater management in downtown Wichita. http://hdl.handle.net/2097/15703
- Karki, R., Hasson, S., Schickhoff, U., Scholten, T., & Böhner, J. (2017). Rising precipitation extremes across Nepal. Climate, 5(1), 1–25. https://doi.org/10.3390/cli5010004
- Kaur, R., & Gupta, K. (2022). Blue-Green Infrastructure (BGI) network in urban areas for sustainable storm water management: A geospatial approach. City and Environment Interactions, 16, 100087. https://doi.org/10.1016/j.cacint.2022.100087
- Kharin, V. V., Zwiers, F. W., Zhang, X., & We’hner, M. (2013). Changes in temperature and precipitation extremes in the CMIP5 ensemble. Climatic Change, 119(2), 345–357. https://doi.org/10.1007/s10584-013-0705-8
- Li, Z., Zhang, X., Ma, Y., Feng, C., & Hajiyev, A. (2019). A multi-criteria decision making method for urban flood resilience evaluation with hybrid uncertainties. International Journal of Disaster Risk Reduction, 36, 101140. https://doi.org/10.1016/j.ijdrr.2019.101140
- Lourenço, I. B., Guimarães, L. F., Alves, M. B., & Miguez, M. G. (2020). Land as a sustainable resource in city planning: The use of open spaces and drainage systems to structure environmental and urban needs. Journal of Cleaner Production, 276, 123096. https://doi.org/10.1016/j.jclepro.2020.123096
- Lourenço, I. B., Oliveira, A. K. B., Marques, L. S., Barbosa, A. A. Q., Veról, A. P., Magalhães, P. C., & Miguez, M. G. (2020). A framework to support flood prevention and mitigation in the landscape and urban planning process regarding water dynamics. Journal of Cleaner Production, 277, 122983. https://doi.org/10.1016/j.jclepro.2020.122983
- Luo, P., Luo, M., Li, F., Qi, X., Huo, A., Wang, Z., He, B., Takara, K., Nover, D., & Wang, Y. (2022). Urban flood numerical simulation: Research, methods and future perspectives. Environmental Modelling & Software, 156, 105478. https://doi.org/10.1016/j.envsoft.2022.105478
- Mukherjee, S., Aadhar, S., Stone, D., & Mishra, V. (2018). Increase in extreme precipitation events under anthropogenic warming in India. Weather and Climate Extremes, 20, 45–53. https://doi.org/10.1016/j.wace.2018.03.005
- Mustafa, A., Bruwier, M., Archambeau, P., Erpicum, S., Pirotton, M., Dewals, B., & Teller, J. (2018). Effects of spatial planning on future flood risks in urban environments. Journal of Environmental Management, 225, 193–204. https://doi.org/10.1016/j.jenvman.2018.07.090
- Nigussie, T. A., & Altunkaynak, A. (2019). Modeling the effect of urbanization on flood risk in Ayamama Watershed, Istanbul, Turkey, using the MIKE 21 FM model. Natural Hazards, 99(2), 1031–1047. https://doi.org/10.1007/s11069-019-03794-y
- Nofal, O. M., & Van De Lindt, J. W. (2022). Understanding flood risk in the context of community resilience modeling for the built environment: Research needs and trends. Sustainable and Resilient Infrastructure, 7(3), 171–187. https://doi.org/10.1080/23789689.2020.1722546
- O’donnell, E., Thorne, C., Ahilan, S., Arthur, S., Birkinshaw, S., Butler, D., Dawson, D., Everett, G., Fenner, R., Glenis, V., Kapetas, L., Kilsby, C., Krivtsov, V., Lamond, J., Maskrey, S., O’donnell, G., Potter, K., Vercruysse, K., Vilcan, T., & Wright, N. (2020). The blue-green path to urban flood resilience. Blue-Green Systems, 2(1), 28–45. https://doi.org/10.2166/bgs.2019.199
- Ogden, F. L., Pradhan, N. R., Downer, C. W., & Zahner, J. A. (2011). Relative importance of impervious area, drainage density, width function, and subsurface storm drainage on flood runoff from an urbanized catchment. Water Resources Research, 47(12), 1–12. https://doi.org/10.1029/2011WR010550
- Oliveira, A. K. B. (2018). Drainage system and flood control as a preliminary structuring axis for urban planning: Case study of the Acari River Basin, in the Metropolitan region of Rio de Janeiro [ Master’s dissertation]. Universidade Federal do Rio de Janeiro. http://www.coc.ufrj.br/pt/dissertacoes-de-mestrado/602-msc-pt-2018/8991-antonio-krishnamurti-beleno-de-oliveira
- Oliveira, A. K. B., Rezende, O. M., de Sousa, M. M., Nardini, A., & Miguez, M. G. (2019). An alternative flood model calibration strategy for urban watersheds: The case study of Riohacha, Colombia. Water Science and Technology, 79(11), 2095–2105. https://doi.org/10.2166/wst.2019.211
- Oliveira, A. K. B., Sousa, M. M., Rezende, O. M., Magalhães, P. M. C., Jacob, A. C. P., Magalhães, P. C., & Miguez, M. G. (2022). Evaluating the role of urban drainage flaws in triggering cascading effects on critical infrastructure, affecting urban resilience. Infrastructures, 7(11), 153. https://doi.org/10.3390/infrastructures7110153
- Panteli, M., & Mancarella, P. (2015). The grid: Stronger, bigger, smarter?: Presenting a conceptual framework of power system resilience. IEEE Power and Energy Magazine, 13(3), 58–66. https://doi.org/10.1109/MPE.2015.2397334
- Radesca, F. D., Sousa, M. M. D., Veról, A. P., Oliveira, L. F. G. S., Fraga, J. P. R., Magalhães, P. C. D., & Miguez, M. G. (2021). Multilayer modelling as a support tool for flood diagnosis and drainage system design. In Proceedings of the Institution of Civil Engineers-Water Management (pp. 1–14). Thomas Telford Ltd. https://doi.org/10.1680/jwama.21.00041
- Rio-Águas. (2010). Technical instructions for the elaboration of hydrological studies and hydraulic dimensioning of urban drainage systems. [In Portuguese]. Subsecretariat of Hydrographic Basin Management -Rio-Águas, 1st version. http://www.rio.rj.gov.br/dlstatic/10112/8940582/4244719/InstrucaoTecnicaREVISAO1.pdf
- Romano, B., Fiorini, L., & Marucci, A. (2019). Italy without urban ‘sprinkling’. A uchronia for a country that needs a retrofit of its urban and landscape planning. Sustainability, 11(12), 3469. https://doi.org/10.3390/su11123469
- Sayers, P., Yuanyuan, L., Galloway, G., Penning-Rowsell, E., Fuxin, S., Kang, W., Yiwei, C., & Quesne, T. L. (2013). Flood risk management: A strategic approach. Asian Development Bank, GIWP, UNESCO and WWF-UK. http://hdl.handle.net/11540/81
- Scionti, F., Miguez, M. G., Barbaro, G., Sousa, M. M., Foti, G., & Canale, C. (2018). Integrated methodology for urban flood risk mitigation in Cittanova, Italy. Journal of Water Resources Planning and Management, 144(10), 05018013. https://doi.org/10.1061/(ASCE)WR.1943-5452.0000985
- Sheng, J., & Wilson, J. P. (2009). Watershed urbanization and changing flood behavior across the Los Angeles metropolitan region. Natural Hazards, 48(1), 41–57. https://doi.org/10.1007/s11069-008-9241-7
- Singh, P., Amekudzi-Kennedy, A., Ashuri, B., Chester, M., Labi, S., & Wall, T. A. (2022). Developing adaptive resilience in infrastructure systems: An approach to quantify long-term benefits. Sustainable and Resilient Infrastructure, 8(sup1), 1–22. https://doi.org/10.1080/23789689.2022.2126631
- Sousa, M. M., Oliveira, A. K. B., Rezende, O. M., Magalhães, P. M. C., Pitzer Jacob, A. C., de Magalhães, P. C., & Miguez, M. G. (2022). Highlighting the role of the model user and physical interpretation in urban flooding simulation. Journal of Hydroinformatics, 24(5), 976–991. https://doi.org/10.2166/hydro.2022.174
- Spencer, N., Strobl, E., & Campbell, A. (2022). Sea level rise under climate change: Implications for beach tourism in the Caribbean. Ocean & Coastal Management, 225, 106207. https://doi.org/10.1016/j.ocecoaman.2022.106207
- Tabari, H. (2020). Climate change impact on flood and extreme precipitation increases with water availability. Scientific Reports, 10(1), 1–10. https://doi.org/10.1038/s41598-020-70816-2
- Teng, J., Jakeman, A. J., Vaze, J., Croke, B. F., Dutta, D., & Kim, S. J. E. M. (2017). Flood inundation modelling: A review of methods, recent advances and uncertainty analysis. Environmental Modelling & Software, 90, 201–216. https://doi.org/10.1016/j.envsoft.2017.01.006
- Thorne, C., Lawson, E. C., Ozawa, C., Hamlin, S. L., & Smith, L. A. (2018). Uncertainties and barriers to adoption of BGI. Journal of Flood Risk Management, 11, S960–972. https://doi.org/10.1111/jfr3.12218
- United Nations. (2019). World population prospects 2019.
- Van Heerden, I. L. (2007). The failure of the New Orleans levee system following Hurricane Katrina and the pathway forward. Public Administration Review, 67, 24–35. https://doi.org/10.1111/j.1540-6210.2007.00810.x
- Yang, G., Bowling, L. C., Cherkauer, K. A., & Pijanowski, B. C. (2011). The impact of urban development on hydrologic regime from catchment to basin scales. Landscape and Urban Planning, 103(2), 237–247. https://doi.org/10.1016/j.landurbplan.2011.08.003
- Yang, W., Zhang, J., & Krebs, P. (2022). Low impact development practices mitigate urban flooding and non-point pollution under climate change. Journal of Cleaner Production, 347, 131320. https://doi.org/10.1016/j.jclepro.2022.131320
- Zevenbergen, C., Gersonius, B., & Radhakrishan, M. (2020). Flood resilience. Philosophical Transactions of the Royal Society, 378(2168), 20190212. https://doi.org/10.1098/rsta.2019.0212
- Zhang, X. Q. (2016). The trends, promises and challenges of urbanisation in the world. Habitat International, 54(13), 241–252. https://doi.org/10.1016/j.habitatint.2015.11.018
- Zhang, Y., Wang, Y., Chen, Y., Liang, F., & Liu, H. (2019). Assessment of future flash flood inundations in coastal regions under climate change scenarios—a case study of Hadahe River basin in northeastern China. The Science of the Total Environment, 693, 133550. https://doi.org/10.1016/j.scitotenv.2019.07.356
- Zilli, M. T., Carvalho, L. M. V., & Silva, M. A. (2016). A comprehensive analysis of trends in extreme precipitation over southeastern coast of Brazil. International Journal of Climatology, 37(5), 2269–2279. https://doi.org/10.1002/joc.4840