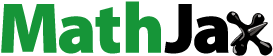
ABSTRACT
Scour is a significant cause of bridge failure, and resulting bridge closures are likely to generate significant disruption to infrastructure networks. The management of scour-susceptible bridges is a significant challenge for improving transport resilience, but tends to be heuristic and qualitative. Such assessments often suffer from insufficient knowledge of key factors and require assumptions, which may increase their estimation and relative uncertainty. Analysis of publicly available technical documents reveals that various definitions of “risk” are adopted, as well as multiple approaches are applied. This paper has three objectives: (i) to illustrate the concept of risk in bridge scour management; (ii) to propose a simple scoring system to analyse existing risk-based approaches to manage bridge scour; and (iii) to analyse and compare such approaches on the basis of the obtained scores. A sample of nine documents containing bridge scour risk assessment practices or approaches was analysed using the developed rating system.
1. Introduction
Bridges are vital infrastructure assets for transport functionality and are crucial for economic activity. Considering the 17 Sustainable Development Goals (SDGs), bridges play a critical role in enhancing road connectivity and building resilient infrastructure systems and lifelines (SDG9, Breuer et al., Citation2019), as well as improving the management of human settlements (SDG11, Breuer et al., Citation2019). Djalante et al. (Citation2013) and Werner et al. (Citation2021) highlighted that improving resilience of infrastructure systems to the effects of disasters and climate change is vital for long-term development of sustainable infrastructure. These efforts include improving long-term infrastructure management and related investment decisions (Tellman et al., Citation2018). In a context of more extreme flood events (and relative hydraulic actions), understanding current management of bridges at risk of scour is the pre-requisite for improving the safety and resilience of transport networks through better management, toward more resilient and sustainable infrastructure development. In this remit, this paper seeks to compare different risk-based methods to manage scour, and hence improve maintenance and reliability of bridges and the underpinned transport network.
Bridges are associated with high maintenance, repair, and (re)building costs, and have serious consequences in the event of failure (Wong et al., Citation2005). Aging bridges worldwide are becoming increasingly vulnerable due to the increased traffic loading and other external factors such as climate change (Nasr et al., Citation2020), thus reducing the resilience of transportation networks. Structures crossing or adjacent to watercourses are exposed to hydraulic actions, which could result in multiple hazards (e.g., overtopping, debris, scour). The scouring of foundations due to such actions is a major cause of bridge failures. Assessing scour hazard and risk is of upmost importance for bridge and transport managers (e.g., Briaud, Citation2015; Ding et al., Citation2016; Kerenyi & Flora, Citation2019; Maddison, Citation2012; Prendergast & Gavin, Citation2014; Whitbread et al., Citation1996).
Bridge scour is defined as the erosion of soil from around bridge foundations and abutments, which in turn can lead to a reduction in support capacity (e.g., Ettema et al., Citation2017; Hager, Citation2007; Maddison, Citation2012; Melville & Coleman, Citation2000; Whitbread et al., Citation1996; Whitbread et al., Citation2000); it is a process that may be exacerbated by high river and/or turbulent flows (e.g., due to flooding). The phenomenon of scour includes different types, namely general, contraction/constriction and local scour (Maddison, Citation2012; Melville & Coleman, Citation2000). A range of damage mechanisms and failure modes are triggered when scour holes reveal the bottom of or undermine shallow foundations, compromising the integrity and safety of the entire structure (Bento et al., Citation2018; Bento, Couto, et al., Citation2022; Mondoro & Frangopol, Citation2018; Wang et al., Citation2014). To maintain bridge resilience, owners and operators should consider how best to prevent, detect, and mitigate scour effects (Tubaldi et al., Citation2022).
In the context of scour, bridge integrity management is the process of identification and implementation of remedial actions to counter scour effects in a timely fashion, before they threaten structural integrity, cause damage (leading to repairs or rebuilding), or reduce asset functionality (e.g., Argyroudis et al., Citation2020; Gidaris et al., Citation2017; Hurt & Schrock, Citation2016). The main goal is to optimally keep the asset functional for a set reference period.
Bridge portfolios are often managed through bridge management systems, which typically rank structures according to their level of risk. This risk ranking supports decision-making and the subsequent allocation of resources (e.g., Bento et al., Citation2020; Flaig & Lark, Citation2000; Proske, Citation2018). However, the ranking is often based on ‘in-house’ (set by each owner/agency) definitions or perceptions of risk, that present noticeable differences (Pregnolato et al., Citation2022). Tubaldi et al. (Citation2022) discussed limitations in current risk assessment approaches, which usually give more weight to the contribution of the hazard, as compared to bridge vulnerability and potential failure consequences. Also, consequences are considered by means of simple heuristic coefficients (Tubaldi et al., Citation2022).
This paper analyzes existing methods to assess bridge scour risk using a scoring system. The objectives of the paper are: (i) to illustrate the concept of risk in bridge scour management; (ii) to propose a simple scoring system to analyse existing risk-based approaches to manage bridge scour; and (iii) to analyse and compare such approaches on the basis of the obtained scores.
2. Scour management approaches
Risk can be defined as the combination of hazard, vulnerability, and consequences (Grossi & Kunreuther, Citation2005). The scour hazard is often estimated using indicators that depend on the characteristics of the stream, the channel, and the floodplain, e.g., the floodwater level. In some cases, these intensity measures are qualitatively assessed via expert opinion; in other cases, more refined hydraulic modelling is implemented to determine the hazard for given return periods (Bento et al., Citation2021; Bento et al., Citation2022; Flint et al., Citation2017). The vulnerability is represented usually via fragility curves (e.g., Lamb et al., Citation2019) that describe the probability that the bridge is in a failure state at a given exposure. Similarly, to the hazard evaluation, vulnerability can be estimated using indicators; for example, of the foundation type and depth, or with higher accuracy, using detailed numerical models calibrated using results of tests and monitoring (e.g. see the monitoring methodology for scour presented in Anderson et al., (Citation2007)). Both hazard and vulnerability are needed to sensibly estimate the probability of bridge failure, and thereby the bridge reliability.
Reliability with respect to a given failure can be defined as the probability that in each timeframe the structure does not ‘fail’. The term ‘failure’ in this paper generally indicates limit conditions that must be avoided. The scour reliability can be written as the complement of the probability of failure (Ditlevsen & Madsen, Citation1996):
where is the probability that the bridge fails due to scour in a given time interval. An example of a reliability-based study for scour is provided by Alipour et al. (Citation2013).
However, reliability by itself does not enable accounting for the consequences related to damage which in turn may be a decisive factor in prioritizing maintenance interventions, especially under budget constraints (). Consequences are either direct or indirect (Chryssanthopoulos et al., Citation2011; Hackl et al., Citation2018; Imam & Chryssanthopoulos, Citation2012; JCSS, Citation2008; Lamb et al., Citation2019). Direct consequences result from physical damage of the structure or of its components (e.g., repair and replacement), and from fatalities and casualties. Direct consequences may relate to material, tangible values, that are quantifiable as economic losses, or intangible values such as human life, cultural values, loss of reputation, and quality of the environment (e.g., noise, pollution, loss of scenic beauty, biodiversity). Indirect consequences result from the loss of functionality of the bridge (e.g., re-routing, delays, increased pollution). Indirect consequences can be expressed in economic terms but should also account for social and environmental costs. For indirect consequences, it is an established norm to differentiate between tangible (e.g., traffic disruption) and intangible (e.g., damage to the environment or historical heritage) indirect consequences (e.g., Thoft-Christensen, Citation2012). Intangible consequences are difficult to express in monetary terms and often are not included in risk evaluations (Tubaldi et al., Citation2022).
Figure 1. Reliability and risk concepts in bridge integrity management. (Abbreviations: pf = probability of failure, ci = indirect consequences, cd = direct consequences).
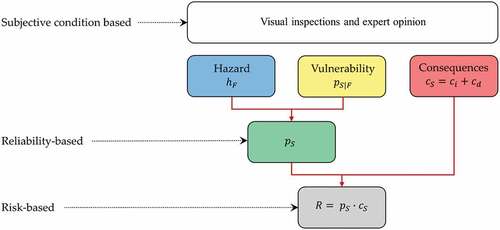
The estimation of the overall consequences is a complex multi-faceted problem. For example, the collapse of a bridge can lead to casualties and injuries (direct consequences) or traveling delays (indirect consequences) (c.f. HA Citation2012; Pregnolato, Vardanega, et al., Citation2021). Various methodologies have been proposed to quantify the total consequences (both direct and indirect) associated with bridge scour failure (e.g., NASEM, Citation2007; Pregnolato et al., Citation2021; Stein et al., Citation1999; Zhu & Frangopol, Citation2016). Reliability and risk are related; however, they lead to different approaches for bridge integrity management ().
The traditional reliability-based approach aims to maintain the load-carrying functionality by keeping the computed probability of failure below a target value. This approach implicitly reduces the risk of failure but does not explicitly account for the consequences of the bridge performance on the network ecosystem to which the bridge belongs. Two identical bridges under the same hazard have the same reliability; however, their failure may lead to consequences of different orders of magnitude. For example, the consequences (in terms of human life, loss of time for deviations, pollution, etc.) of the failure of an isolated bridge in a scarcely populated area are significantly different from the consequences of the failure of a bridge located on a highly congested highway. A complete risk-based approach would explicitly account for both direct and indirect consequences of the bridge failure. A risk-based method would be more suitable to prioritize maintenance interventions with respect to an approach that only accounts for safety through reliability. Following the definition from the JCSS (Citation2008), the risk of scour failure can be written as the product of the probability
that the bridge fails due to scour for a given time frame and the consequences
associated with this failure:
The probability depends on the hazard and the vulnerability. In this study, the hazard is modelled through the probability
that an event that can trigger scour, for example a flood, occurs within the given time frame. The vulnerability is represented by the conditional probability
that the bridge fails due to scour, given that the flood occurs. Hence, Eq. 2 can be written as:
EquationEquation (3)(3)
(3) does not refer to the full-life cycle of an asset since it considers one failure of the bridge only throughout its lifetime.
The traditional approach to bridge integrity assessment is generally based on the identification of condition scores derived from visual inspection data (Middleton Citation2004; Bennetts et al., Citation2018; Collins et al., Citation2019; Limongelli et al., Citation2018; Tűrksezer et al., Citation2021). Visual inspection efforts rely on engineering judgment to produce the condition metrics often used to assess bridge condition (e.g., Bennetts et al., Citation2020; Yuan et al., Citation2019).
The onset and evolution of scour and associated effects relate to variations in hydraulic parameters (e.g., flow rate, flow velocity, sediment type) and to the structural characteristics of the bridge (e.g., pier geometry, foundation shape) (e.g., Bao & Liu, Citation2017; Bento et al., Citation2020; Pizarro et al., Citation2020; Prendergast & Gavin, Citation2014; Prendergast et al., Citation2016). Thereby the management of the integrity of bridges prone to scour requires up-to-date information about key hydraulic and structural parameters (Moreno, Citation2016). The water height, water velocity, or flow rate are indicators of the potential hazard (scour). This information may be recorded using river gauges or sensors, or simulated via hydraulic models (Bento et al., Citation2022). The characteristics of the riverbed (e.g., material), of the catchment (e.g., steep/lowland), and the presence of debris may also be relevant indicators (e.g., Panici et al., Citation2020).
However, assessing the hydrodynamic actions alone is not sufficient to judge the overall condition of a bridge and the vulnerability to scour needs to be accounted for (Pregnolato, Citation2019). The foundation type, geometry (e.g., shape of piers), and foundation depth are fundamental indicators where scour risk management is concerned (Malekjafarian et al., Citation2020). Infrastructure operators and agencies have therefore developed various methods for identifying bridges at risk of scour with varying levels of complexity. This paper develops a new scoring system to assess this complexity and compares various existing published approaches to scour management.
Scour risk estimation and associated bridge management are often limited by: (i) insufficient knowledge about the structural configuration, the structural conditions, and the factors that influence the scour hazard (e.g., hydraulic parameters); (ii) a heuristic application of the concept of risk, with partial consideration of consequences; and (iii) the scarce (if any) integration of the information measured by monitoring systems.
3. A scoring system for risk-based scour assessment methods
To assess the level of refinement of a practical risk-based method, this paper proposes a scoring system () based upon the three elements of risk: hazard (), vulnerability (
) and consequences (indirect Ci, direct tangible
, and direct intangible
). Importantly, the scoring system differentiates between: (i) estimated factors, i.e., those parameters that are approximated using qualitative indicators or expert (subjective) judgement; and (ii) assessed factors, i.e., those parameters that are assessed via objective computation using numerical, empirical, or theoretical models. For each method, the resulting score is obtained by summing up the scores of the five factors (max score = 9) and indicates the level of refinement of the procedure (basic/intermediate/advanced), as shown in .
Table 1. Scoring system for risk-based methods. N/A means that the factor has not been considered. (Abbreviations: hf = scour probability; ps|f = failure probability, ci = indirect consequences, cd = direct consequences).
Table 2. Score ranking for risk-based methods; reliability-based methods not considered.
4. Survey of published methods for scour assessment
In this section, nine published approaches to scour management are scored using the framework presented in . The nine published approaches were selected due to their availability in the public domain. summarizes the results of the review. A given agency can offer a procedure at different levels of refinement (e.g., with successive, more refined stages of analysis); this work considers the most refined process reported in the reviewed publications and it is acknowledged that more sophisticated methods may be used in practice by the agencies reviewed in this paper (and unknown or unavailable to the authors).
Table 3. Summary of the review of scour management methods. Acronyms: Cerema - Centre d’études et d’expertise sur les risques, l’environnement, la mobilité et l’aménagement; MIT - Ministero delle Infrastrutture e dei Trasporti; MOTC - Directorate General of Highways; Mn DOT - Minnesota Department of Transport. Final scores (‘TOTAL SCORE’) were associated with the following ranking for risk-based methods (): 0–3 basic (BAS); 4–6 intermediate (INT); 7–9 advanced (ADV).
4.1. NR (2011) - UK
Network Rail (NR) operates and maintains most of the railway bridges in the UK. The bridge scour ‘risk’ model of NR (NR, Citation2011) is grounded on scour vulnerability via the Preliminary Priority Rating, based on the ratio between foundation depth and predicted scour depth. NR establishes a priority of interventions for bridges vulnerable to scour through a reliability-based three-step process; railway bridges are designed and assessed for a return period of 200-year (Dikanski et al., Citation2017). Stage 1 is a preliminary assessment and is based on the ‘EX2502 scour method’ (HR Wallingford, Citation1992); it assesses the potential level of ‘risk’ using a preliminary priority rating, computed by the ratio between the scour depth predicted and the assessed foundation depth. For high-rated structures, a Stage 2 assessment is undertaken, using hydraulic modelling to determine likely flows, water levels, discharges, and velocities at the structure for given return periods – based on the CIRIA method (Kirby et al., Citation2015). The results of these analyses are used to recalculate the priority score, similar to Stage 1. The results of Stage 2 normally reduce the number of structures rated as high risk; Stage 3 is undertaken for those that remain. Stage 3 includes the use of 3D models and requires specialist software, such as 3D hydraulic and 3D ground survey models. Readers are referred to the EX2502 (HR Wallingford, Citation1992), the CIRIA C742 manual (Kirby et al., Citation2015) and the Rail Safety and Standards Board standards (RSSB, Citation2004) for detailed guidance.
Reliability indicators include information from the stream (water height, water velocity), the channel (riverbed), the structure (foundation depth, pier geometry) and the catchment (floodplain). Likely intensity measures of the hazard (e.g., flood depth, velocity) are computed for the 1-in-a-200-year return period, so the hazard can be considered to score a 2 in the scoring system proposed in . The vulnerability is estimated using indicators related to the geometry of the piers, thus it scores a 1. Consequences are not considered in the process (cf. Lamb et al., Citation2019; Sasidharan et al., Citation2022). Therefore, the method cannot be considered risk-based (see Sec. 2) and it is scored as N/A in . Monitoring is not mandated to inform the assessment and/or reduce uncertainty in the evaluation; however, NR aims to develop safer and cheaper technology-based practices (compared to diving) to determine if a bridge is safe after a flood event (NR, Citation2019).
4.2. HA (Citation2012) - UK
HA (Citation2012) stated that ‘the ratio of calculated total scour depth to depth of foundations is the main indicator of risk’ (p4/15). Their scour risk rating includes two components: such a ratio (i.e., relative scour depth), and the Priority Factor. National Highways (NH; formerly Highways England – HE and Highways Agency – HA) assesses bridge risk using a two-stage risk-based method (HA, Citation2012). Stage 1 determines an initial risk rating for the bridge based on its geometry, an underwater examination, the history of scour, the presence of any scour protection, and floodplain information. Several assumptions are made such as the type and depth of the bridge foundations and the general hydrology of the river. Bridges with a scour-risk rating over a certain threshold proceed to a Level 2 assessment. Similar to Stage 3 of NR’s procedure, Stage 2 includes the calculation of: (i) the total scour depth (normalised by foundation depth), based on flood discharge for a 1-in-200-year flood event; and (ii) the Priority Factor, which includes multiple parameters that may influence the likelihood of damage, such as: past scour incidents, foundation type and construction material, type of river, and the importance of the bridge structure. Direct consequences are not considered in the input parameters. For the relevant equations, readers can refer to BD97/12, Chapter 5 Volume 3 Section 4 Part 21 (HA, Citation2012).
Reliability indicators include information about foundation depth, riverbed, floodplain, catchment properties, scour records, and protection. The hazard (flow depth and velocity) is determined from a hydraulic analysis based on the assessment flow. The assessment flow is determined at the site using statistical analysis of flood data available using the Flood Estimation Handbook and associated software (IH, Citation1999) with a return period of 200 years; therefore, the hazard is scored as 2. The vulnerability compares a potential depth of scour with the actual depth of the bridge foundation, estimated using indicators; this comparison gives the major parameter of the prioritization, thus scoring a 1. Consequences include indirect consequences only, in terms of bridge importance. The bridge importance is denoted by an Importance Factor given by the road type, as a proxy for traffic flow; this estimation scores a 1. Overall, the method obtains a score of 4 and qualifies as ‘intermediate’. Monitoring is not integrated into the method; however, this aspect is explicitly mentioned as eventual means of support for specialist appraisal and decision-making.
4.3. Cerema (Citation2019) - France
In France, a methodology developed by Cerema (Centre d’études et d’expertise sur les risques, l’environnement, la mobilité et l’aménagement) (Cerema, Citation2019) proposes to assess scour risk to existing bridges and to identify solutions for extending their lifetime. They define risk as a combination of vulnerability, exposure, and hazard through qualitative and semi-qualitative approaches. Return periods are not specified. The methodology is organized in four successive stages. Stage 1 ‘Summary analysis’ provides a preliminary risk classification (Low, Medium, High) based on a qualitative approach that does not require calculations and is based on assessment grids. Three classes of hazard and vulnerability are defined, as well as five classes of strategic importance. The assessment grids contain different hazard (e.g., flow conditions, riverbed) and vulnerability indicators (e.g., shape of piers, construction period, foundation types); they define the partial scores to be assigned to each of them in relation to the characteristics of both the bridge and the river. The partial scores are assigned using engineering judgement. Empirical equations combine the different partial scores to obtain a global score, which allows definition of the level of hazard and the level of vulnerability.
Direct and indirect consequences of bridge failure are accounted for at this stage qualitatively. Direct consequences include replacement consequences (score 1); tangible indirect consequences relate to the strategic importance of the structure, the traffic level, and detours (score 1); intangible indirect consequences include historic value and the potential casualties due to the collapse of the bridge (score 1). The combination of the levels of hazard/vulnerability and the class of strategic importance allow definition of the preliminary classification of risk. For bridges that are classified as being at medium or high risk, Stage 2 ‘Simplified analysis’ requires more precise and exhaustive data on the bridges (e.g., riverbed composition and foundation geometry) and leads to a more realistic assessment of the level of risk (low, medium, high). In this case, the analysis concerns the combined use of theoretical equations and qualitative indices. Consequences are computed according to the methodology used during the first phase. Based on this information, direct and indirect consequences are scored 1. For those bridges which are still classified as at high risk, the Stage 3 ‘Detailed analysis’ is carried out by assessing scour depth with analytical (i.e., HEC-18 formulae, Arneson et al., Citation2012), numerical (e.g., software for hydraulic modelling) or empirical methods (e.g., laboratory tests). Therefore, a score of 2 is assigned to hazard and vulnerability. Stage 4 ‘Management of risk’ concerns risk management actions for those bridges that have shown criticalities in the previous stages, such as structural interventions, and modifications of hydraulic conditions. Cerema (Citation2019) mentions monitoring, but they do not provide specific information about its adoption. The final score obtained by this method is 7, thus it can be classified as an ‘advanced’ method.
4.4. CSLP (Citation2020) - Italy
The Italian Ministry of Infrastructure and Transport (MIT) recently issued an official guideline (CSLP, Citation2020) which proposes a multilevel procedure to manage any existing bridge. CSLP (Citation2020) defines risk as the combination of vulnerability, exposure, and hazard. Different types of risk are accounted for: structural and foundation failure, seismic, landslide, and hydraulic (which includes scour). The procedure is organized in six levels, with increasing degrees of complexity. Level 0 and Level 1 are devoted to the acquisition of data on the assets from existing documents (Level 0) and visual inspections (Level 1). Level 2 is the core of the approach and consists of the processing of the collected data and in the definition of the global level of risk (five levels: ‘low’, ‘medium-low’, ‘medium’, ‘medium-high’, and ‘high risk’). The analysis is carried out by combining principal and secondary parameters in assessment grids. Scour risk indicators relate to contraction scour and local scour, whose equations consider factors such as: riverbed, width of piers and abutments, floodplains, and ratio of scour to the depth of the foundation. For specific guidance on the analysis process, interested readers should refer to CSLP (Citation2020).
Consequences of bridge unavailability are considered in a qualitative way in the analysis of Level 2 only and by means of secondary parameters, e.g., presence of alternative routes, bridge strategic importance, and the value (social, economic, human, naturalistic) of the ‘entity’ under the bridge; human losses are included in relation to the bridge length (which is assumed as a proxy for the number of fatalities in case of collapse). The score for direct and indirect consequences is 1 in both cases. The additional point for intangible values is given since the method explicitly includes the ‘environmental, economic and social’ value of the bridge surroundings, which might be damaged by its collapse. Level 2 implies a detailed analysis of the hazard and a qualitative assessment of the vulnerability. So, a score of 2 and 1 is given to these factors, respectively. Regarding the hazard, the minimum clearance is “estimated by considering the distance between the bridge soffit and the water level”, in relation to a specified and different return periods (e.g., 10/50/200 years) (Pregnolato et al. (Citation2022): 5). The level of risk related to scour contributes to the definition of the global level of risk. If the global level of risk is assessed as being between medium and high, Levels 3 and 4 are then addressed. Level 3 includes additional investigations on the anomalies identified in Level 1, and preliminary studies on the load bearing capacity of the bridge. Level 4 concerns a careful assessment of the safety of the bridge according to current building code. Level 5 is with regard to the resilience of critical infrastructure, but it is not directly addressed in the guidelines. The level of risk determines surveillance and monitoring activities, including both visual inspections, the execution of occasional static and dynamic tests, and the installation of permanent or discrete monitoring systems. The final score of this method is 6, thus the MIT method is classified as ‘intermediate’.
4.5. Abé et al. (Citation2014) - Japan
Abé et al. (Citation2014) overviewed the monitoring and risk management for Japanese railway bridges under general hazards including wind, vehicle collisions and scour. Among monitoring sensors for scour, inclinometers can measure the change in rotation of a pier resulting from scour occurrence, while accelerometers can detect changes in natural frequency due to the loss of stiffness associated with scour (Prendergast & Gavin, Citation2014). Abé et al. (Citation2014) did not provide an explicit definition of risk, but it can be understood that risk management of Japanese railway bridges is based on risk curves defined as the number of accidents vs the number of deaths for post-derailment modes. In this methodology, data from inclinometer and accelerometer measurements is used as input for the development of a vulnerability indicator; so, vulnerability scores 2 according to the scoring system postulated in . In terms of hazard, geological and hydraulic conditions are used to evaluate scour potential, resulting in a score of 1 for hf (); return periods are not mentioned. The consequences of scour are considered as the potential suspension of railway operations. Considering that indirect consequences associated with bridge closure are qualitatively taken into consideration, a score of 1 can be given for Ci from .
Tangible and intangible direct consequences are not mentioned in the reviewed literature, resulting in scores of 0 for both items according to . The total score for Japanese Railways is 4 according to resulting in an ‘intermediate’ level of refinement and sophistication according to . On some bridges, natural frequency is monitored (using accelerometers) (Kim et al., Citation2020) as a method to infer scour presence, with excitation typically resulting from flow-induced vibration. Some scour-metrics including proportional changes in frequency have been put forward for monitoring purposes, whereby the frequency measured is normalized by the baseline undamaged frequency. In relation to the bridge network, identified priorities include enhancement of safety, reduction in disaster-related failures and effective data-utilization (such as local wind and structural performance) and there is a trend towards monitoring-based systems to assist with this (Abé et al., Citation2014). One method involving monitoring inclinations of pier elements assists decision-making related to when to close a bridge, determined as the point when the inclinations become excessive.
4.6. Cheng et al. (Citation2019) - Taiwan
Cheng et al. (Citation2019) described a risk-based approach for life-cycle maintenance for bridges (termed REMBMS) in Taiwan. The effects of scour and earthquakes, as well as deterioration, on bridge safety are evaluated using this model. In Cheng et al. (Citation2019), the concept of risk is based on a model that ‘considers the three main risk factors of component deterioration, scouring and earthquakes in order to minimise the expected life-cycle cost of bridge maintenance’ (p.334). The national bridge management database (Taiwan Bridge Management System (TBMS)) is used to store historical data related to bridge damage from the network. This data can be used with an ‘evolutionary support vector machine inference model (ESIM)’ (Cheng et al., Citation2019) to estimate consequences associated with scour-risk, facilitating a predictive approach to scour evaluation and maintenance. The TBMS was originally developed with a view to assisting government agencies in directing and prioritizing visual inspections for bridges.
Their method is underpinned by the following procedure. Firstly, REMBMS uses Monte Carlo simulations to estimate the probability of a bridge requiring maintenance based on historical data. For this reason, the hazard can be considered as being directly assessed (from historical data), which scores 2 in the criteria outlined in . Return periods (from 1- to 200-year) are used for calculating the scouring stability index. Then, a hybrid artificial intelligence (AI) model is employed to assess the impact of a variety of risk factors on financial consequences. Then, the probability of bridge maintenance is multiplied by the calculated consequences to obtain the expected cost. REMBMS employs a ‘Symbiotic Organism Search (SOS) algorithm’ for estimation of minimum maintenance costs. This process is a bridge maintenance strategy that can then be used to minimize life cycle maintenance consequences.
In terms of scour-risk indicators, Scouring Stability Index (SSI) based on ‘Degree of deterioration’, ‘Extent of deterioration’, ‘Relationship between deterioration and safety’, and ‘Urgency of repair’ (DERU) is used (see Cheng et al., Citation2019). The DERU approach is used to inspect bridge components (e.g., left-hand abutment, pier, etc.) and rate them on a scale of 0–4 (where ‘0’ denotes the component does not exist, ‘1’ denotes a good condition, and ‘4’ denotes an extremely poor condition). Using the DERU approach, a Condition Index (CI) can be generated. For scour specifically, SSI is specified similarly to CI but only uses components in the DERU that can be affected by scour, i.e., waterway, abutment foundations, abutments, scour protection for piers, piers and columns themselves. As indicators based on the visual condition of the elements affected by scour are considered, this scores 1 for vulnerability using , since structural behaviour is not modelled. By combining data of the flood return period with the SSI slope index, calculation of ‘statistically declining SSI curves for each bridge’ can be carried out using Monte Carlo simulations. Maintenance probability can then be derived. The method takes scour-related maintenance and rebuilding consequences into consideration as direct consequences, therefore scoring 2 for tangible direct consequences in . These consequences need to be included in long-term bridge management budgets and are explicitly considered. The method does not consider indirect consequences, therefore scoring 0 in this category. Given intangible direct consequences are also not mentioned, this scores 0 according to . The total score for the method in Cheng et al. (Citation2019) is 5, resulting in an ‘intermediate’ risk-based ranking according to . In terms of using monitoring data, the method does not use real-time records, and instead uses historical records.
4.7. Kim et al. (Citation2018) - South Korea
Kim et al. (Citation2018) described a method for ‘risk-based maintenance prioritization’ which assesses 14 general risk-factors (including flood/rainfall and scour, among others) that may result in bridge damage. The study, while not scour-specific, provided a general overview of bridge risk-management. Risk is defined as ‘cause for damage to a bridge’ (p. 3721), and computed via a ‘Risk Factor Score’ as the product between the hazard probability and its relative impact. Probability and impact were quantitatively assessed using a five-point Likert scale (Likert, Citation1932) ranging from Very High to Very Low. This assessment results in a score of 2 from for hf as hazard is quantified. The study considered 14 ‘risk factors’ (e.g., flood/rainfall, strong wind, extreme heat wave, etc.; p. 3721); no specific return periods were provided. In terms of vulnerability, this would result in a score of 1 by applying the conditions in , as it implies that scour risk is estimated based on rudimentary elements such as geometry or otherwise.
In terms of the consequences, an Importance Coefficient considers consequences such as the detour distance in the event of bridge closure. As this coefficient is an assessed quantity, this scores 2 for Ci (), since detour distances are taken explicitly into account in the event a bridge should be closed by applying weights to annual average daily traffic assessments. In terms of tangible direct consequences, coefficients that quantify impact are adopted but consequences are not directly calculated, giving a score of 1. For intangible direct consequences, these are considered by being ranked on a five-point scale, where a high score implies high possibility of personal injury, therefore a score of 1 from is applied here. According to the ranking framework, the total score for the approach in Kim et al. (Citation2018) is 7, which falls into the ‘advanced’ category in . Monitoring is not mandated for this method.
4.8. Mn DOT (2009) - USA
Minnesota Mn DOT (Citation2009) requires bridges to be designed to survive predicted scour. Relative risk is discussed to some extent, but risk is not formally defined mathematically. For existing bridge structures, a four-step process is proposed. The first step ‘Primary Screening’ examines the ‘history of scour, foundation stability and protection from scour’ (Mn DOT, Citation2009, p. 2) to rate bridges as ‘low’, ‘unknown foundation’ and ‘scour susceptible’ (Mn DOT, Citation2009). For ‘scour susceptible’ bridges, steps 2 or 3 may be carried out. Step 2 ‘Secondary Screening’ considers the following parameters: ‘historical scour performance, scour resistant foundations, debris and blockage, geomorphic conditions, hydraulic conditions, structural conditions, and special low risk conditions’ (Mn DOT, Citation2009, p. 3). Step 3 is ‘Scour Analysis’ and requires four sub-tasks: (1) ‘office data collection’, i.e., examination of the bridge conditions such as past inspection reports, soil and geological conditions, and historical photographs; (2) ‘review and evaluation of collected data’; (3) ‘site visit and field assessment’, i.e., collection of further site-specific information including evidence of past scour events; and (4) ‘scour analysis’, which uses peak flood estimates, Mannings n values, and the field cross-section data (HEC-18 method) to estimate contraction and pier scour depths. In the final stage, scour depths are predicted and compared with the current design conditions. Finally, step 4 ‘Plan of Action’ determines what should happen in the event of a flood. Monitoring of at-risk bridges is part of the plans of action for the network, unless foundation conditions are well-known, the traffic is low or the bridge is scheduled for replacement.
During the ‘office data collection’ phase (step 3), peak flood discharges are calculated during the review of the collected data; return periods were not defined. For this reason, a score of 2 is given to the hazard. After the ‘scour analysis’ (step 3), bridge stability is assessed. A score of 2 is given for the vulnerability as in the scour rating codes, these are based on calculated scour depths. A score of 1 is given for the indirect consequences as the ADT (Average Daily Traffic) of the bridge is considered, e.g., to classify the road as ‘local’ (low volume). The overall score is 5 and this classifies the method as ‘intermediate’. While the method is risk-based, the consequences are not assessed in detail.
4.9. Coleman and Melville (Citation2001) – New Zealand
Coleman and Melville (Citation2001) published a proposed methodology that would allow for identification of ‘at-risk sites’, allowing for national ‘evaluation of scour risk’, nationally prioritizing ‘annual expenditure on scour-related works’, and comparing said expenditure against other expenditure. A formal definition of risk is not provided, but it can be understood that it is “based on the bridge significance and overall bridge vulnerability”(p.50). The proposed two-stage process includes a simple vulnerability ranking phase followed by a more advanced assessment for higher risk bridges. The first ‘office-review’ phase excludes bridges that are scheduled to be rebuilt and bridges that are not over a water course are removed from the advanced scour screening process. The second stage assesses bridge vulnerability and characteristics of the waterway using a four-level scale risk-rating methodology (‘unknown’, ‘high’, ‘medium’, and ‘low’). This assessment allows for bridges to be ranked on a susceptibility scale from 1 to 4 (4 is the lowest risk of scour).
The process is mainly subjective as it employs a simple risk-rating system to assess the bridge ‘significance’ and bridge ‘vulnerability’. The method notes that if a bridge is deemed ‘at-risk’ and remedial works are proposed (which may include monitoring, according to Coleman and Melville (Citation2001)), then an Economic Ranking Indicator (ERI) is applied to assess the cost-vs-benefits of any proposed remediation efforts. The document calls for bridges with higher ERI values to be ranked ‘on the basis of vulnerability, risk and economic justification’ (Coleman & Melville, Citation2001 p. 22).
For the initial screening process (which is reviewed in this paper) there are two stages: office review and vulnerability assessment. During the office review, the considered parameters included: bridge operational status, whether or not the bridge is riverine, channel characteristics (e.g., bed materials, sediment grading, etc.), construction typology, any incidences of historical scour at the bridge site, traffic volume, and an assessment of alternative route availability. If the bridge carries utilities, this is also noted. This evaluation means that for indirect consequences (Ci) a score of 1 is assigned, as the assessment is done qualitatively mainly via an assessment of traffic volume.
For the bridge vulnerability assessment, a series of parameters related to the following categories are rated using the four-level scale: ‘Catchment developments/conditions’, ‘Historical scour’, ‘Degradation and contraction’, ‘Aggradation’, ‘Waterway adequacy’, ‘Local scour at piers and abutments’. Therefore, the vulnerability is given a score of 1 due to the qualitative nature of the assessment (noting that many factors are considered).
No discussion of the likelihood of flooding (or return period) is included in the screening methodology. However, it is noted that whether or not the bridge is riverine and some hazard indicators are identified such as: ‘lateral channel movement’ (Coleman & Melville, Citation2001, p. 17), ‘flow depths in bends and confluences’ (Coleman & Melville, Citation2001, p. 7), incidences of historical scour and debris. Authors assumed that data is collected for assessment purposes, however how this assessment is carried out was not specified. For this reason, the hazard score is given as 1. The methodology is rated as scoring 3 and therefore is ‘basic’. This score is expected as this is an initial scour screening methodology. It is acknowledged more sophisticated approaches for specific sites based on the ERI indicator mentioned in Coleman and Melville (Citation2001) may also be employed in conjunction with the approach reviewed in this section.
5. Discussion and future directions
This study introduced a simple scoring system useful to compare approaches to bridge scour management and used it to analyse and compare multiple published risk-based approaches. Although the scoring system could be used in future studies, it was developed not to be universal or transferable, rather as a tool to allow for comparison of different methods within the scope of this paper.
This study was developed using publicly available reports and published literature for nine transport agencies; the authors accept that alternative documents could be in use (and not necessarily publicly available) by relevant authorities, or that independent studies could have proposed modifications to national methods (e.g., Bento et al. (Citation2020) for HA (Citation2012)’s method). Not all documents are available in English, e.g., the Danish code (O’Connor & Enevoldsen, Citation2009), as well as the Italian and the French codes. Also, interpreting information and formulating consistent judgments was among the challenges of this study, especially due to the inconsistency of terminology among agencies and approaches: the scores given in this paper are from the judgement and interpretation of the selected approaches by the present authors.
Water and foundation depth were the most common indicators identified in the examined documents as input to estimate the scour risk, while consequences assumed a mixed range of direct and indirect consequences, mostly including detours. Indirect consequences are included in almost all (7 of 9) analysed methods; direct tangible and intangible consequences are included by four methods and three methods, respectively. Direct consequences are more likely assessed for extreme shocks (e.g., earthquakes), rather than slower processes of degradation (such as scour); although bridges can collapse quite suddenly under specific single events, scour failure is often the result of an accumulation process (Tubaldi et al., Citation2017). Intangible consequences (human losses, cultural values, etc.) are seldom considered (possibly due to the difficulty of their assessment). Considering final scores, one approach was judged reliability-based since consequences were not accounted for in the method; one (risk-based) method scored as ‘basic’, five as ‘intermediate’ and two as ‘advanced’. Nevertheless, the level of sophistication of its implementation differs from one method to the other. This difference may depend on how significant a problem scour poses for the region for which the method was developed, and on the available budget for bridge management. offers an example of visualisation of the scores of the scoring system and a summary of the results obtained from its application to the nine selected methods.
Figure 2. Graphical summary of the results for the reviewed methods, as an example of visualisation of the scores of the scoring system. The total score is considered as follows: 0–3 basic (BAS); 4–6 intermediate (INT); 7–9 advanced (ADV). Abbreviations: hf = scour probability; ps|f = failure probability, ci = indirect consequences, cd = direct consequences.
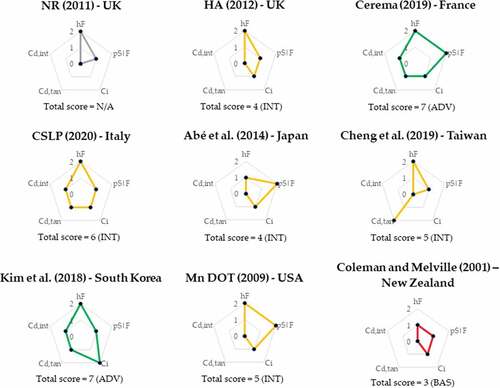
The results of this analysis could also be used to review the selected methods and reflect on potential improvements. For example, the HA (Citation2012)’s method in the UK and the Kim et al. (Citation2018)’s method in South Korea could reach a higher score by including direct consequences.
For six of the nine approaches reviewed, the evaluation of risk does not incorporate monitoring into their current methods of assessment. However, reliance on visual inspections and expert opinions may lead to inconsistencies in decision-making and management introduced by the subjectivity in the estimation of the structural condition (Nepomuceno et al., Citation2022). Therefore, in the last years there has been an effort to improve the knowledge on structural conditions by means of objective tools, such as monitoring technologies (Maroni et al., Citation2022). There are many recent publications reviewing technologies for scour monitoring (e.g., Prendergast & Gavin, Citation2014; Vardanega et al., Citation2021) and scour prediction (e.g., Austroads, Citation2018; Ettema, Constantinescu, & Melville, Citation2017; Kariyawasam et al., Citation2019a, Citation2019b, Citation2020; Pizarro et al., Citation2020) that may enable a more objective structural evaluation, and thereby of the scour vulnerability and risk. Monitoring sensors could provide a step-change in collecting data, locating damage and alerting for danger, especially if developed alongside AI (Artificial Intelligence, e.g., data-driven evaluation of structural conditions, automatic data processing of large databases, smart operation and effective maintenance). For example, in the case of the reviewed literature relating to Taiwan (Cheng et al., Citation2019), AI is integrated into optimization algorithms which help to estimate the economic implications associated with scour risk.
Further studies could increase the complexity of the framework developed in this paper and broaden the underpinning concept of risk (e.g., risk to communities, environment, etc.); for example, Panici et al. (Citation2020) demonstrated that an approach which includes debris effects would increase the estimated scour risk for debris-prone bridges with respect to approaches that do not account for this effect. Moreover, considering future strategies of asset adaptiveness, the system could be further developed by including the presence of scour countermeasures (such a rip-rap) (Martín-Vide et al., Citation2010). Finally, this initial version of the scoring system assigned equal weight to all the risk components, which is an assumption that should be verified in the future. Future scour assessment methods may consider pioneering the inclusion of additional factors (e.g., recovery) to move towards the adoption of a resilience-based approach in bridge scour management (Tubaldi et al., Citation2022). These suggested improvements could foster improved infrastructure system resilience especially with respect to disasters and effects of climate change by building system adaptiveness, towards long-term sustainability.
6. Summary and conclusions
Scour is a major cause of bridge failure, which could result in significant service disruptions, undermining the resilience of the underpinned infrastructure network. In practice, the management of bridges susceptible to scour tends to remain a heuristic process, which is mainly visual and reliant on engineering judgement. A risk-based approach is the preliminary condition to develop resilience-based approaches (e.g., accounting for recovery) in the future. However, the concept of ‘risk-based’ scour management differs depending on the publication reviewed. Considering the risk components (hazard, vulnerability and consequences), this paper has presented a simple scoring system as a tool to analyse and compare existing methods.
The developed scoring system has been applied to nine published methods. The analysis showed that the risk-based concept is applied at varying degrees of refinement across the samples studied. In fact, of the analysed nine approaches, one risk-based method scored as ‘basic’, five as ‘intermediate’ and two as ‘advanced’. (one was N/A). Monitoring is rarely integrated into the existing approaches and most of them do not define refined indicators of the environmental actions and of the asset conditions, nor the direct and indirect consequences of different maintenance interventions and policies.
This work proposes a new simple scoring system that allows the analysis and comparison of different risk-based methods for bridge scour assessment. The proposed scoring system enables the comparison of risk-based scour management policies as it can assist agencies with their in-house development of scour assessment methods, helping them to benchmark their approaches with respect to others, as well as sustaining the development of new risk-based methods. Future studies will also investigate the integration of information (e.g., from monitoring) and scour countermeasures in risk-based approaches, to assist practice and decision-making for transport resilience and adaptiveness, towards long-term sustainability.
Acknowledgments
Maria Pregnolato was supported by the Engineering and Physical Sciences Research Council (ESPRC) LWEC (Living With Environmental Change) Fellowship (EP/R00742X/2). Luke J. Prendergast acknowledges the Faculty of Engineering, University of Nottingham, for travel funding to facilitate this collaboration. This study was partially funded by the Italian Civil Protection Department within the project Accordo CSLLPP e ReLUIS 2021-2022 “WP3: Analisi, revisione e aggiornamento delle Linee Guida”.
Disclosure statement
No potential conflict of interest was reported by the authors.
Data availability statement
No data, models, or code were generated or used during the study.
Additional information
Funding
Notes on contributors
M. Pregnolato
Dr Maria Pregnolato is a Senior Lecturer in Infrastructure Resilience in Civil Engineering at the University of Bristol (UK). Her work focuses on infrastructure resilience and risk management from natural hazards, in particular the impact of flooding to road networks and bridges. She held an EPSRC (Engineering and Physical Sciences Research Council) Fellowship to investigate the impact of extreme flooding to bridges, including hydrodynamic modelling and scour risk management. In recent years, she has been focusing on structural health monitoring and Digital Twins, exploring the role of sensing and digitalisation within infrastructural assets exposed to flooding and natural hazards.
Pier Francesco Giordano
Dr. Pier Francesco Giordano is Assistant Professor (RTDa) at the Department of Architecture, Built Environment and Construction Engineering (DABC) of Politecnico di Milano, Italy, where he received his PhD cum laude, in 2021. His current research activities include the topics of bridge management, Value of Information from Bayesian decision theory, and Structural Health Monitoring. He graduated cum laude with the international Master’s Degree in Civil Engineering from University of Bologna, Italy, in 2016. Also, he graduated with the Erasmus Mundus Master’s Degree in Structural Analysis of Monuments and Historical Constructions (SAHC) from both University of Minho, Portugal, and Polytechnic University of Catalonia, Spain, in 2017.
L.J. Prendergast
Dr Luke J. Prendergast is Assistant Professor in Civil Engineering at University of Nottingham, UK. Prior to this, Luke worked as a Postdoctoral Researcher at the Faculty of Civil Engineering and Geosciences, Delft University of Technology in the Netherlands. Luke obtained his PhD at University College Dublin, Ireland (2015) and holds a Bachelor of Engineering (Civil) from University College Cork, Ireland (2011). Luke works as an Assistant Editor at the (Elsevier) Journal of Sound and Vibration (JSV), with focus on research related to damage identification in civil engineering structures. His current research interests include Structural Health Monitoring, Vibration-Based Bridge Scour Monitoring, Vehicle-Bridge Interaction, and Offshore Wind Foundation Engineering.
P.J. Vardanega
Dr Paul J. Vardanega is an Associate Professor in Civil Engineering at the University of Bristol. He has continuing research interests in fundamental soil behaviour, development of geotechnical databases, structural health monitoring, pavement engineering and bridge scour. He is a Graduate Member of the Institution of Civil Engineers, a Member of the American Society of Civil Engineers, a Member of the Institution of Engineers Australia and a Fellow of the Higher Education Academy. He is one of the co-authors of the 2016 book 'Bridge Monitoring: A practical guide' published by ICE Publishing.
Maria Pina Limongelli
Prof. Maria Pina Limongelli received her PhD in Seismic Engineering at Politecnico di Milano. She is currently Associate Professor of Structural and Seismic Engineering at Politecnico di Milano. Her research interests in the field of Structural Health Monitoring of civil structures include vibration-based damage identification, value of Information of SHM, and SHM Standardization. She is an active member with leading roles in several committees and associations in the field of SHM and structural engineering (among others ISHMII, fib, IABSE, and JCSS). She serves on the editorial board of several international peer-reviewed journals including the Engineering Structures, ASCE Journal of Bridge Engineering, Journal of Civil SHM, Infrastructures, and Sustainable and Resilient Infrastructures. She participates in leading roles in several national and international funded projects on Seismic and Structural Health Monitoring and performance assessment of bridges.
References
- Abé, M., Shimamura, M., & Fujino, Y. (2014). Risk management and monitoring of Japanese railway bridges. Proceedings of the Institution of Civil Engineers - Forensic Engineering, 167(2), 88–98. https://doi.org/10.1680/feng.13.00022
- Alipour, A., Shafei, B., & Shinozuka, M. (2013). Reliability-based calibration of load and resistance factors for design of RC bridges under multiple extreme events: Scour and earthquake. Journal of Bridge Engineering (ASCE), 18(5), 362–371. https://doi.org/10.1061/(ASCE)BE.1943-5592.0000369
- Anderson, N. L., Ismael, A. M., & Thitimakorn, T. (2007). Ground-penetrating radar: A tool for monitoring bridge scour. Environmental & Engineering Geoscience, 13(1), 1–10. https://doi.org/10.2113/gseegeosci.13.1.1
- Argyroudis, S. A., Mitoulis, S. A., Hofer, L., Zanini, M. A., Tubaldi, E., & Frangopol, D. M. (2020). Resilience assessment framework for critical infrastructure in a multi-hazard environment. Science of Total Environment, 714, [136854]. https://doi.org/10.1016/j.scitotenv.2020.136854
- Arneson, L., Zevenbergen, L., Lagasse, P., & Clopper, P. (2012). HEC-18 evaluating scour at bridges. Report No. FHWA-HIF-12-003HEC-18. Federal Highway Administration. https://www.fhwa.dot.gov/engineering/hydraulics/pubs/hif12003.pdf [07/10/2022]
- Austroads. (2018). Guide to Bridge Technology Part 8. Hydraulic Design of Waterway Structures. Austroads Publication No. AGBT08-18, https://bit.ly/3DiXHCg [07/10/2022]
- Bao, T., & Liu, Z. (2017). Vibration-based bridge scour detection: A review. Structural Control Health Monitoring, 24(7), [e1937]. https://doi.org/10.1002/stc.1937
- Bennetts, J., Vardanega, P. J., Taylor, C. A., & Denton, S. R. (2020). Survey of the use of data in UK bridge asset management. Proceedings of the Institution of Civil Engineers – Bridge Engineering, 173(4), 211–222. https://doi.org/10.1680/jbren.18.00050
- Bennetts, J., Webb, G., Denton, S., Vardanega, P. J., & Loudon, N. (2018). Quantifying uncertainty in visual inspection data. In: Maintenance, Safety, Risk, Management and Life-Cycle Performance of Bridges: Proceedings of the Ninth International Conference on Bridge Maintenance, Safety and Management (IABMAS 2018),9-13 July 2018Melbourne, Australia. (N. Powers et al. (Eds.)) CRC Press/Balkema, Leiden, the Netherlands, pp. 2252–2259.
- Bento, A. M., Couto, L., Pêgo, J. P., & Viseu, T. (2018). Advanced characterization techniques of the scour hole around a bridge pier model. In: River Flow 2018 - Ninth International Conference on Fluvial Hydraulics, E3S Web of Conferences, 40: [05066]. https://doi.org/10.1051/e3sconf/20184005066
- Bento, A. M., Couto, L., Viseu, T., & Pêgo, J. P. (2022). Image-based techniques for the advanced characterization of scour around bridge piers in laboratory. Journal of Hydraulic Engineering, 148(6), [06022004]. https://doi.org/10.1061/(ASCE)HY.1943-7900.0001981
- Bento, A. M., Gomes, A., Pêgo, J. P., Viseu, T., & Couto, L. (2022). Improved assessment of maximum streamflow for risk management of hydraulic infrastructures. A case study. International Journal of River Basin Management, 1–11. ahead of print. https://doi.org/10.1080/15715124.2021.2016783
- Bento, A. M., Gomes, A., Viseu, T., Couto, L., & Pêgo, J. P. (2020). Risk-based methodology for scour analysis at bridge foundations. Engineering Structures, 223, [111115]. https://doi.org/10.1016/j.engstruct.2020.111115
- Bento, A. M., Gomes, A., Viseu, T., Couto, L., & Pêgo, J. P. (2022). Assessment of scour risk in hydraulic infrastructures. A bridge case study. In: International Association for Bridge and Structural Engineering (IABSE) Symposium Report Volume 118,25-27 May 2022 Prague, Czech Republic. (IABSE (Eds)) IABSE, pp 1366–1373.
- Bento, A. M., Viseu, T., Pêgo, J. P., & Couto, L. (2021). Experimental characterization of the flow field around oblong bridge piers. Fluids, 6(11), [370]. https://doi.org/10.3390/fluids6110370
- Breuer, A., Janetschek, H., & Malerba, D. (2019). Translating sustainable development goal (SDG) interdependencies into policy advice. Sustainability, 11(7), 2092. https://doi.org/10.3390/su11072092
- Briaud, J. -L. (2015). Scour depth at bridges: Method including soil Properties. I: Maximum Scour depth prediction. Journal of Geotechnical and Geoenvironmental Engineering (ASCE), 141(2), [04014104]. https://doi.org/10.1061/(ASCE)GT.1943-5606.0001222
- Cerema. (2019). Analyse de risque des ponts en site affouillable (in French). Collection: Références.
- Cheng, M. Y., Chiu, Y. F., Chiu, C. K., Prayogo, D., Wu, Y. W., Hsu, Z. H., & Lin, C. H. (2019). Risk-based maintenance strategy for deteriorating bridges using a hybrid computational intelligence technique: A case study. Structure and Infrastructure Engineering, 15(3), 334–350. https://doi.org/10.1080/15732479.2018.1547767
- Chryssanthopoulos, M. K., Janssens, V., & Imam, B. (2011). Modelling of failure consequences for robustness evaluation. In IABSE–IASS Symposium: Taller, Longer, Lighter, London, UK. https://core.ac.uk/download/pdf/8767199.pdf [07/10/2022]
- Coleman, S. E., & Melville, B. W. (2001). Bridge-scour screening methodology for New Zealand bridges. Transfund New Zealand Research Report No. 196. 108pp. https://www.nzta.govt.nz/assets/resources/research/reports/196/196-Bridge-scour-screening-methodology-for-New-Zealand-bridges.pdf [07/10/2022]
- Collins, J., Ashurst, D., Webb, J., Sparkes, P., & Ghose, A. (2019). Guidance on hidden defects in bridges in the UK and Ireland. Proceedings of the Institution of Civil Engineers - Bridge Engineering, 172(1), 41–53. https://doi.org/10.1680/jbren.18.00021
- CSLP. (2020). Linee Guida per la Classificazione e Gestione del Rischio, la Valutazione della Sicurezza ed il Monitoraggio dei Ponti Esistenti. Ministero delle Infrastrutture e dei Trasporti. Consiglio Superiore dei Lavori Pubblici In Italian https://www.mit.gov.it/sites/default/files/media/notizia/2020-05/1_Testo_Linee_Guida_ponti.pdf [07/10/2022].
- Dikanski, H., Hagen-Zanker, A., Imam, B., & Avery, K. (2017). Climate change impacts on railway structures: Bridge scour. Proceedings of the Institution of Civil Engineers: Engineering Sustainability, 170(5), 237–248. https://doi.org/10.1680/jensu.15.00021
- Ding, Y., Yan, T., Yao, Q., Dong, X., & Wang, X. (2016). A new type of temperature-based sensor for monitoring of bridge scour. Measurement, 78, 245–252. https://doi.org/10.1016/j.measurement.2015.10.009
- Ditlevsen, O., & Madsen, H. O. (1996). Structural reliability methods. John Wiley & Sons Inc.
- Djalante, R., Holley, C., & Thomalla, F. (2013). Pathways for adaptive and integrated disaster resilience. Natural Hazards, 69(3), 2105–2135. https://doi.org/10.1007/s11069-013-0797-5
- Ettema, R., Constantinescu, G., & Melville, B. (2017). Flow-field complexity and design estimation of pier-scour depth: Sixty years since Laursen and Toch. Journal of Hydraulic Engineering (ASCE), 143(9), [03117006]. https://doi.org/10.1061/(ASCE)HY.1943-7900.0001330
- Flaig, K. D., & Lark, R. J. (2000). The development of UK bridge management systems. Proceedings of the Institution of Civil Engineers – Transport, 141(2), 99–106. https://doi.org/10.1680/tran.2000.141.2.99
- Flint, M. M., Fringer, O., Billington, S. L., Freyberg, D., & Diffenbaugh, N. S. (2017). Historical analysis of hydraulic bridge collapses in the continental United States. J. of Infr Systems, 23(3), [04017005]. https://doi.org/10.1061/(asce)is.1943-555x.0000354
- Gidaris, I., Padgett, J. E., Barbosa, A. R., & Chen, S. (2017). Multiple-hazard fragility and restoration models of highway bridges for regional risk and resilience assessment in the United States: State-of-the-art review. Journal of Structural Engineering (ASCE), 143(3), [04016188]. https://doi.org/10.1061/(ASCE)ST.1943-541X.0001672
- Grossi, P., & Kunreuther, H.(2005).Catastrophe modeling: A new approach to managing risk. Huebner international series on risk, insurance and economic security. Springer https://www.springer.com/gp/book/9780387230825. [07/10/2022]
- Hackl, J., Adey, B. T., & Lethanh, N. (2018). Determination of near-optimal restoration programs for transportation networks followingnatural hazard events using simulated annealing. Computer-Aided Civil and Infrastructure Engineering, 33(8), 618–637. https://doi.org/10.1111/mice.12346
- Hager, W. H. (2007). Scour in hydraulic engineering. Proceedings of the Institution of Civil Engineers – Water Management, 160(3), 159–168. https://doi.org/10.1680/wama.2007.160.3.159
- Highways Agency (HA). (2012). The assessment of scour and other hydraulic actions at highway structures BD97/12. Design Manual for Roads & Bridges. HMSO, https://www.standardsforhighways.co.uk/prod/attachments/8ff7a31b-1ce0-4e34-9e94-b2372f125f34?inline=true [07/10/2022]
- HR Wallingford, R. (1992). Hydraulic aspects of bridges: Assessment of the risk of scour. Report EX2502, HR, Wallingford, https://eprints.hrwallingford.com/315/[07/10/2022]
- Hurt, M., & Schrock, D. S. (2016). Chapter 7 - Bridge Management. In: Highway bridge maintenance planning and scheduling (Hurt, M. & Schrock, D. S. (Eds.)). Butterworth-Heinemann pp. 289–310; https://doi.org/10.1016/B978-0-12-802069-2.00007-6.
- Imam, B., & Chryssanthopoulos, M. K. (2012). Causes and consequences of metallic bridge failures. Structural Engineering International, 22(1), 93–98. https://doi.org/10.2749/101686612X13216060213437
- Institute of Hydrology (IH). (1999). Flood Estimation Handbook (five volumes). Centre for Ecology & Hydrology.
- JCSS. (2008). Risk assessment in engineering. Principles, system representation and risk criteria. JCSS (Joint Committee on Structural Safety) report. In Faber M.H. (Ed.) ISBN 978-3-909386-78-9
- Kariyawasam, K., Fidler, P., Talbot, J., & Middleton, C. (2019a). Field assessment of ambient vibration-based bridge scour detection. In: Structural Health Monitoring 2019: Enabling Intelligent Life-cycle Health Management for Industry Internet of Things (IIOT): Proceedings of the 12thInternational Workshop on Structural Health Monitoring, September 10–12, 2019 (F. Chang, A. Güemes, & F. Kopsaftopoulos (Eds.)). Stanford, CA, USA, pp. 374–381. https://doi.org/10.12783/shm2019/32137
- Kariyawasam, K., Fidler, P., Talbot, J., & Middleton, C. (2019b). Field deployment of an ambient vibration-based scour monitoring system at Baildon Bridge, UK. In: Proceedings of the International Conference on Smart Infrastructure and Construction. Cambridge, UK: ICE Publishing UK, 711–719 https://doi.org/10.1680/icsic.64669.711
- Kariyawasam, K., Middleton, C., Madabhushi, G., Haigh, S., & Talbot, J. P. (2020). Assessment of bridge natural frequency as an indicator of scour using centrifuge modelling. Journal of Civil Structural Health Monitoring, 10(5), 861–881. https://doi.org/10.1007/s13349-020-00420-5
- Kerenyi, K., & Flora, K. (2019). A hybrid approach to forensic study of bridge scour. Proceedings of the Institution of Civil Engineers – Forensic Engineering, 172(1), 27–38. https://doi.org/10.1680/jfoen.19.00001
- Kim, D., Lee, Y., & Lee, M. J. (2018). Development of risk-based bridge maintenance prioritization methodology. KSCE Journal of Civil Engineering, 22(10), 3718–3725. https://doi.org/10.1007/s12205-018-2058-3
- Kim, C. W., Yoshitome, K., Qi, J., Kitagawa, S., & Hamada, Y. (2020). Vibration-based long-term scour monitoring of railway bridges. XI International Conference on Structural Dynamics, M. Papadrakakis, M. Fragiadakis, & C. Papadimitriou (Eds.), Athens, Greece 2020.
- Kirby, A. M., Roca, M., Kitchen, A., Escarameia, M., & Chesterton, O. J. (2015). Manual on scour at bridges and other hydraulic structures (2nd ed.). CIRIA.
- Lamb, R., Garside, P., Pant, R., & Hall, J. W. (2019). A probabilistic model of the economic risk to Britain’s Railway Network from bridge scour during floods. Risk Analysis, 39(11), 2457–2478. https://doi.org/10.1111/risa.13370
- Liao, K. -W., Lu, H. -J., & Wang, C. -Y. (2015). A probabilistic evaluation of pier-scour potential in the Gaoping River Basin of Taiwan. Journal of Civil Engineering and Management, 21(5), 637–653. https://doi.org/10.3846/13923730.2014.890650
- Likert, R. (1932). A technique for the measurement of attitudes. Archives of Psychology, 22(140), 5–55.
- Limongelli, M. P., Chatzi, E., & Anzlin, A. (2018). Condition assessment of roadway bridges: From performance parameters to performance goals. The Baltic Journal of Road and Bridge Engineering, 13(4), 345–356. https://doi.org/10.7250/bjrbe.2018-13.421
- Maddison, B. (2012). Scour failure of bridges. Proceedings of the Institution of Civil Engineering – Forensic Engineering, 165(1), 39–52. https://doi.org/10.1680/feng.2012.165.1.39
- Malekjafarian, A., Kim, C., Obrien, E. J., Prendergast, L. J., Fitzgerald, P. C., & Nakajima, S. (2020). Experimental demonstration of a mode shape-based scour monitoring method for multi-span bridges with shallow foundations. Journal of Bridge Engineering, 25(8), [04020050]. https://doi.org/10.1061/(ASCE)BE.1943-5592.0001586
- Maroni, A., Tubaldi, E., McDonald, H., & Zonta, D. (2022). An SHM-based classification system for risk management of bridge scour. Proceedings of the Institution of Civil Engineers - Smart Infrastructure and Construction, 175(2), 92–102. https://doi.org/10.1680/jsmic.21.00016
- Martín-Vide, J. P., Roca, M., & Alvarado-Ancieta, C. A. (2010). Bend scour protection using riprap. Proceedings of the Institution of Civil Engineers - Water Management, 163(10), 489–497. https://doi.org/10.1680/wama.2010.163.10.489
- Melville, B. W., & Coleman, S. E. (2000). Bridge scour (pp. 572). Highlands Ranch, Colorado, USA: Water resources publication.
- Middleton, C. R. (2004). Bridge management and assessment in the UK. In: Proceedings of Austroads, 5th Bridge Conference, Hobart, Tasmania, Australia, 19-21 May 2004. http://www.bridgeforum.org/files/pub/2004/austroads5/Middleton%20keynote.pdf [07/10/2022]
- Minnesota Department of Transportation (Mn DOT). (2009). Bridge scour evaluation procedure for Minnesota Bridges [on-line]. December 2009. https://www.dot.state.mn.us/bridge/pdf/hydraulics/ScourGuidelines_12-09.pdf [07/10/2022]
- Mondoro, A., & Frangopol, D. M. (2018). Risk-based cost-benefit analysis for the retrofit of bridges exposed to extreme hydrologic events considering multiple failure modes. Engineering Structures, 159, 310–319. https://doi.org/10.1016/j.engstruct.2017.12.029
- Moreno, M. E. C. (2016). Experimental study of local scour around complex bridge piers. Ph.D. Thesis. University of Porto, Porto, Portugal. http://hdl.handle.net/10216/85290 [06/10/2022.]
- Nasr, A., Kjellström, E., Björnsson, I., Honfi, D., Ivanov, O. L., & Johansson, J. (2020). Bridges in a changing climate: A study of the potential impacts of climate change on bridges and their possible adaptations. Structure and Infrastructure Engineering, 16(4), 738–749. https://doi.org/10.1080/15732479.2019.1670215
- National Academies of Sciences, Engineering , and Medicine (NASEM). 2007. Risk-Based Management Guidelines for Scour at Bridges with Unknown Foundations. The National Academies Press. https://doi.org/10.17226/23243
- Nepomuceno, D., Bennetts, J., Pregnolato, M., Tryfonas, T., & Vardanega, P. J. (2022). Development of a schema for the remote inspection of Bridges. Proceedings of the Institution of Civil Engineers – Bridge Engineering ( ahead of print). https://doi.org/10.1680/jbren.22.00027
- NR. (2019). Scour prevention and management. https://bit.ly/3tMdrWd [07/10/2022]
- NR (Network Rail). (2011). NR/L3/CIV/020 Design of Bridges. Issue 1, March 11.
- O’Connor, A., & Enevoldsen, I. (2009). Probability-based assessment of highway bridges according to the new Danish guideline. Structure and Infrastructure Engineering, 5(2), 157–168. https://doi.org/10.1080/15732470601022955
- Panici, D., Kripakaran, P., Djordjević, S., & Dentith, K. (2020). A practical method to assess risks from large wood debris accumulations at bridge piers. Science of Total Environment, 728, [138575]. https://doi.org/10.1016/j.scitotenv.2020.138575
- Pizarro, A., Manfreda, S., & Tubaldi, E. The science behind Scour at Bridge foundations: A review. (2020). Water, 12(2), 374. 374. https://doi.org/10.3390/w12020374
- Pregnolato, M. (2019). Bridge safety is not for granted – a novel approach for bridge management. Engineering Structures, 196, [109193]. https://doi.org/10.1016/j.engstruct.2019.05.035
- Pregnolato, M., Bates, P., Winter, A. O., Mascarenas, D., Sen, A. D., & Motley, M. R. (2021). An integrated impact analysis for riverine bridges subjected to high river flows. In: Bridge Maintenance, Safety, Management, Life-Cycle Sustainability and Innovations: Proceedings of the Tenth International Conference on Bridge Maintenance, Safety and Management (IABMAS 2020), Sapporo, Japan, 11-15 April 2021 (H. Yokota & D. M. Frangopol (Eds.)). CRC Press/Balkema Taylor & Francis Group, The Netherlands, pp. 1258–1264. https://doi.org/10.1201/9780429279119-170
- Pregnolato, M., Giordano, P. F., Panici, D., Prendergast, L. J., & Limongelli, M. P. (2022). A comparison of the UK and Italian national risk-based guidelines for assessing hydraulic actions on bridges. Structure and Infrastructure Engineering, 1–14. ahead of print. https://doi.org/10.1080/15732479.2022.2081709
- Pregnolato, M., Vardanega, P. J., Limongelli, M. P., Giordano, P. F., & Prendergast, L. J. (2021). Risk-based scour management: A survey. In: Bridge Maintenance, Safety, Management, Life-Cycle Sustainability and Innovations: Proceedings of the Tenth International Conference on Bridge Maintenance, Safety and Management (IABMAS 2020), Sapporo, Japan, 11-15 April 2021 (H. Yokota & D. M. Frangopol (Eds.)). CRC Press/Balkema Taylor & Francis Group, The Netherlands, pp. 693–701. https://doi.org/10.1201/9780429279119-91
- Prendergast, L. J., & Gavin, K. (2014). A review of bridge scour monitoring techniques. Journal of Rock Mechanics and Geotechnical Engineering, 6(2), 138–149. https://doi.org/10.1016/j.jrmge.2014.01.007
- Prendergast, L. J., Hester, D., & Gavin, K. (2016). Determining the presence of scour around bridge foundations using vehicle-induced vibrations. Journal of Bridge Engineering (ASCE), 21(10), [04016065]. https://doi.org/10.1061/(ASCE)BE.1943-5592.0000931
- Proske, D. (2018). Bridge collapse frequencies versus failure probabilities. In Risk Engineering. Springer International Publishing. https://doi.org/10.1007/978-3-319-73833-8
- Rail Safety and Standards Board (RSSB). (2004). Impact of scour and flood risk on railway structures, Infrastructure Integrity (4) Research Theme: Report Number T112. https://www.rssb.co.uk/en/research-catalogue/CatalogueItem/T554 [07/10/2022]
- Sasidharan, M., Parlikad, A. K., & Schooling, J. (2022). Risk-informed asset management to tackle scouring on bridges across transport networks. Structure and Infrastructure Engineering, 18(9), 1300–1316. https://doi.org/10.1080/15732479.2021.1899249
- Stein, S. M., Young, G. K., Trent, R. E., & Pearson, D. R. (1999). Prioritizing scour vulnerable bridges using risk. Journal of Infrastructure Systems (ASCE), 5(3), 95–101. https://doi.org/10.1061/(ASCE)1076-0342(1999)5:3(95)
- Suzuki, O., Masui, Y., Abe, M., Samizo, M., & Shimamura, M. (2008). Development of multifunction Scour monitoring device for Railway Bridge Piers part 1: Background and concept. Fourth International Conference on Scour and Erosion 2008, Japanese Geotechnical Society, Tokyo, Japan, pp. 263–266. https://henry.baw.de/bitstream/handle/20.500.11970/100127/b_1.pdf?sequence=1&isAllowed=y [07/10/2022]
- Tellman, B., Bausch, J. C., Eakin, H., Anderies, J. M., Mazari-Hiriart, M., Manuel-Navarrete, D., & Redman, C. L. (2018). Adaptive pathways and coupled infrastructure: Seven centuries of adaptation to water risk and the production of vulnerability in Mexico City. Ecology and Society, 23(1), [1]. https://doi.org/10.5751/ES-09712-230101
- Thoft-Christensen, P. (2012). Infrastructures and life-cycle cost-benefit analysis. Structure & Infrastructure Engineering, 8(5), 507–516. https://doi.org/10.1080/15732479.2010.539070
- Tubaldi, E., Macorini, L., Izzuddin, B. A., Manes, C., & Laio, F. (2017). A framework for probabilistic assessment of clear-water scour around bridge piers. Structural Safety, 69, 11–22. https://doi.org/10.1016/j.strusafe.2017.07.001
- Tubaldi, E., White, C. J., Patelli, E., Mitoulis, S. A., De Almeida, G., Brown, J., Cranston, M., Hardman, M., Koursari, E., Lamb, R., McDonald, H., Mathews, R., Newell, R., Pizarro, A., Roca, M., & Zonta, D. (2022). Invited perspectives: Challenges and future directions in improving bridge flood resilience. Natural Hazards and Earth System Sciences, 22(3), 795–812. https://doi.org/10.5194/nhess-22-795-2022
- Tűrksezer, Z. I., Iacovino, C., Giordano, P. F., & Limongelli, M. P. (2021). Development and implementation of indicators to assess bridge inspection practices. Journal of Construction Engineering and Management (ASCE), 147(12), [04021165]. https://doi.org/10.1061/(ASCE)CO.1943-7862.0002195
- Vardanega, P. J., Gavriel, G., & Pregnolato, M. (2021). Assessing the suitability of bridge-scour-monitoring devices. Proceedings of the Institution of Civil Engineers – Forensic Engineering, 174(4), 105–117. https://doi.org/10.1680/jfoen.20.00022
- Wang, H., Hsieh, S. C., Li, N. C., & Wang, C. Y. (2014). Forensic diagnosis on flood-induced bridge failure. I: Determination of the possible causes of failure. Journal of Performance of Constructed Facilities (ASCE), 28(1), 76–84. https://doi.org/10.1061/(ASCE)CF.1943-5509.0000419
- Werner, E. S., Wise, R. M., Butler, R. A. J., Totin, E. J., & Vincent, K. (2021). Adaptation pathways: A review of approaches and a learning framework. Environmental Science & Policy, 116(2), 266–275. https://doi.org/10.1016/j.envsci.2020.11.003
- Whitbread, J. E., Benn, J. R., & Hailes, J. M. (2000). Cost-effective management of scour-prone bridges. Proceedings of the Institution of Civil Engineers -Transport, 141(2), 79–86. https://doi.org/10.1680/tran.2000.141.2.79
- Whitbread, J. E., Bettess, R., Riddell, J., & Hey, R. D. (1996). Bridge Scour. Proceedings of the Institution of Civil Engineers - Transport, 117(1), 67–69. https://doi.org/10.1680/itran.1996.28146
- Wong, S. M., Onof, C. J., & Hobbs, R. E. (2005). Models for evaluating the consequences of bridge failure. Proceedings of the Institution of Civil Engineers - Bridge Engineering, 158(3), 117–128. https://doi.org/10.1680/bren.2005.158.3.117
- Yuan, V., Argyroudis, S., Tubaldi, E., Pregnolato, M., & Mitoulis, S. (2019). Fragility of bridges exposed to multiple hazards and impact on transport network resilience. Proceedings of the 2019 Society for Earthquake and Civil Engineering Dynamics conference (SECED 2019): Greenwich, London, UK 9-10 Sep 2019.https://openresearch.surrey.ac.uk/esploro/outputs/conferencePresentation/Fragility-of-bridges-exposed-to-multiple/99511698602346 [07/10/2022]
- Zhu, B., & Frangopol, D. M. (2016). Time-variant risk assessment of bridges with partially and fully closed lanes due to traffic loading and Scour. Journal of Bridge Engineering (ASCE), 21(6), [04016021]. https://doi.org/10.1061/(ASCE)BE.1943-5592.0000817