Abstract
Aldrichina grahami is a significant medical and forensic insect belonging to the family calliphoridae. In this study, we present the complete mitochondrial genome of A. grahami for the first time. The mitogenome was 14 903 bp in length, consisting of 22 transfer RNA genes, 2 ribosomal RNA genes and 13 protein-encoding genes. The overall base compositions of A, G, C and T were 39.14, 9.59, 13.65 and 37.62%, respectively. Phylogenetic analysis is conducted using 12 mitochondrial genomes from two families. The monophyletic branches of the phylogenetic tree reveal that the composition of the mitochondrial genome, particularly the gene lengths, is very similar to that of another Calliphoridae fly, C. vicina, but the interspecific variations between them was 5.4%, which could distinguish these two species explicitly. Phylogenetic analyses indicated that phylogenetic analysis of whole mt genome sequences resulted in much stronger support for the identification of A. grahami between other species. This article aimed to enrich the dipteran mitochondrial genomes and provides the complete mitochondrial genome and phylogenetic analyses of A. grahami for species identifications forensic purpose.
Accurate postmortem interval (PMI) estimation has been a focus and a difficulty in forensic practices, especially in putrefactive cases (Yan et al. Citation2014a). Aldrichina grahami (Aldrich, 1930), which belongs to the Aldrichina Townsend genus, Calliphoridae family and Diptera order, is widespread in China except Xinjiang Uygur Autonomous Region. A. grahami is the first wave of sarcosaphagous flies to arrive and oviposit into animal carcasses, and it has been an important essential in forensic entomology (Xu et al. Citation2014). Compared with published growth data from larva of the same species, investigators can approximately infer the age of the larva and estimate the PMI (Guo et al. Citation2011). Viewing it as a potential marker in forensic science, we report the complete mitochondrial genome sequence of A. grahami for species identification and phylogenetic analysis (GenBank accession number: KP872701).
The A. grahami samples were collected in June 2014 from Changsha city and cultured in the laboratory of Central South University. The entire genome was amplified in eight fragments (Nelson et al. Citation2012a, Citation2012b). All of the fragments were amplified by TaKaRa MightyAmp Taq (Takara, Dalian, China) and performed on an Eppendorf Mastercycler gradient (Eppendorf, Hamburg, Germany). PCR amplifications were performed in 20 µL reaction volumes containing 0.5 µL of DNA, 0.5 µL of each primer, 1.0 µL (1.25 U) of TaKaRa MightyAmp Taq polymerase, 10 µL of MightyAmp PCR Buffer and 7.5 µL of sterilized distilled water. The PCR thermal cycling conditions were as follows: denaturation for 2 min at 94 °C, followed by 35 cycles at 98 °C for 10 s, 50 °C for 30 s, 68 °C for 2.5 min and a final elongation at 68 °C for 10 min. DNA fragments were sequenced on both strands using Sanger dideoxy sequencing method by the commercial service (Transduction Bio Co. Ltd. Wuhan, China).The complete genome of A. grahami is circular 14 903 bp in length with 22 transfer RNA genes, 13 protein-coding genes, 2 ribosomal RNA genes and a control region as in other insects. The 89 bp misc_feature region of A. grahami was located between 12S rRNA and tRNA-Ile. The genome is particularly AT-biased, with 76.76% of the nucleotides being either A or T, which is slightly more AT-rich than that for Parasarcophaga similis (76.37%) (Yan et al. Citation2014b) and Sarcophaga africa (75.74%) (Fu et al. Citation2014), but less AT-rich than that for Ravinia pernix (77.17%) (Guo et al. Citation2014). Among the 13 protein-coding genes, 12 were identified with ATN as start codon coding for methionine except COI.
Phylogenetic analysis is conducted using the 12 complete mitochondria gene sequences from six species of Calliphoridae, along with two from family Sarcophagidae as outgroup species. Two of the Calliphoridae species, L. cuprina and L. sericata, include multiple specimens, allowing genomic variability within these species to be assessed. The sequence data from GenBank which are A. grahami, C. vicina, C. rufifacies, P. terraenovae, four L. cuprina, two L. sericata of Calliphoridae and S. crassipalpis, S. similis from family Sarcophagidae. Except A. grahami and S. crassipalpis, the other Calliphoridae genomes originally published in Nelson LA’s paper (Nelson et al. Citation2012b), S. similis published in Yan’s (Yan et al. Citation2014b) and S. crassipalpis published in Ramakodi MP’s (Ramakodi et al. Citation2015). Each of the 12 mt genomes was aligned separately using Vector NTI 9.0. (Lu & Moriyama Citation2004) and individually aligned gene data sets were translated into amino acid sequences using MEGA 5 (Tamura et al. Citation2011). The evolutionary history was deduced using the neighbor-joining method (Saitou & Nei Citation1987). The bootstrap consensus tree inferred from 500 replicates (Felsenstein, Citation1985) was conducted to represent the evolutionary history of the analyzed taxa (Felsenstein, Citation1985). Branches corresponding to partitions reproduced in <50% bootstrap replicates were collapsed. The percentage of replicate trees is shown next to the branches, in which the associated taxa clustered together in the bootstrap test (500 replicates). The maximum composite likelihood method was used to compute the evolutionary distances (Tamura et al. Citation2004). Codon positions included were first + second + third non-coding.
The phylogeny of Calliphoridae flies based on the complete mitochondria gene sequences was separated into several genetic clades (). As outgroup, the two Sarcophagidae samples were clustered together and clearly separated from the Calliphoridaid mitotypes. The monophyletic branches of the phylogenetic tree reveal that the composition of the A. grahami mitochondrial genome, particularly the gene lengths, is very similar to that of another Calliphoridae fly, C. vicina, but the interspecific variations between them was 5.4%, which could distinguish these two species explicitly. The interspecific and intraspecific percentage genetic divergences were calculated. All values for maximum intraspecific variations of the Calliphoridae species were no more than 2%. The interspecific variations between species were larger than 5% except for L. cuprina and L. sericata, which were no more than 2%, the results is consistent with the previous study (Nelson et al. Citation2012a, Citation2012b), which indicated that phylogenetic analysis of whole mt genome sequences resulted in much stronger support for discrimination between A. grahami and other five species, but week for discrimination between the L. cuprina and L. sericata.
Figure 1. Neighbor-joining (NJ) tree of maximum composite likelihood method for the complete mitochondria gene sequences from six species of Calliphoridae. Voucher ID, City, morphological species identification, and accession number are given in specimen label. Numbers on branches indicate the support value. Two from family Sarcophagidae are included as outgroup. Evolutionary distance divergence scale bar is 0.01.
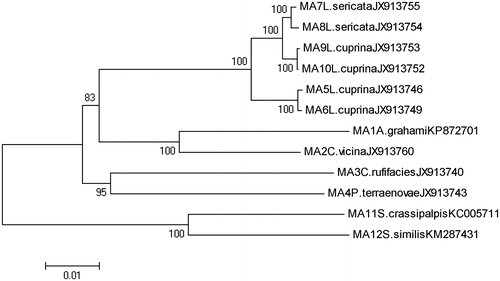
Species identification of sarcosaphagous flies using morphological methods is a difficult task, especially for spawn and larva. Forensic scientists have to wait for the adult emergence (Wang et al. Citation2002). Now species identification techniques of molecular biology including molecular taxonomy serve as an effective supplement for morphological identification. We hope the complete mitochondrial genome and phylogenetic analysis of A. grahami can be valuable for species identification and will be instrumental for implementation of the Calliphoridae database.
Disclosure statement
The authors report no conflicts of interest. The authors alone are responsible for the content and writing of the paper.
Funding information
This study was supported by the National Natural Science Foundation of China (Nos. 81302615, 81201486, 81373249), Grants from the Project of Innovation-driven Plan in Central South University (2015CXS021).
References
- Felsenstein J. 1985. Confidence limits on phylogenies: an approach using the bootstrap. Evolution. 39:783–791.
- Fu XL, Che KX, Zhu ZY, Liu J, Guo YD. 2014. The complete mitochondria genome of Sarcophaga africa (Diptera: Sarcophagidae). Mitochondrial DNA. 24:1–2. [Epub ahead of print].
- Guo JJ, Xie K, Che KX, Hu ZY, Guo YD. 2014. The complete mitochondria genome of Ravinia pernix (Diptera: Sarcophagidae). Mitochondrial DNA. 24:1–2. [Epub ahead of print].
- Guo YD, Cai JF, Tang ZC, Feng X, Lin Z, Yong F, Li JB, Chen YQ, Meng FM, Wen JF. 2011. Application of Aldrichina grahami (Diptera, Calliphoridae) for forensic investigation in central-south China. Rom J Leg Med. 19:55–58.
- Lu G, Moriyama EN. 2004. Vector NTI, a balanced all-in-one sequence analysis suite. Brief Bioinformatics.5:378–388.
- Nelson LA, Cameron SL, Yeates DK. 2012a. The complete mitochondrial genome of the flesh fly, Sarcophaga impatiens Walker (Diptera:Sarcophagidae). Mitochondrial DNA. 23:42–43.
- Nelson LA, Lambkin CL, Batterham P, Wallman JF, Dowton M, Whiting MF, Yeates DK, Cameron SL. 2012b. Beyond barcoding: a mitochondrial genomics approach to molecular phylogenetics and diagnostics of blowflies (Diptera: Calliphoridae). Gene. 511:131–142.
- Ramakodi MP, Singh B, Wells JD, Guerrero F, Ray DA. 2015. A 454 sequencing approach to dipteran mitochondrial genome research. Genomics. 105:53–60.
- Saitou N, Nei M. 1987. The neighbor-joining method: a new method for reconstructing phylogenetic trees. Mol Biol Evol. 4:406–425.
- Tamura K, Dudley J, Nei M, Kumar S. 2011. MEGA 5: Molecular evolutionary genetics analysis using maximum likelihood, evolutionary distance, and maximum parsimony methods. Mol Biol Evol. 28:2731–2739.
- Tamura K, Nei M, Kumar S. 2004. Prospects for inferring very large phylogenies by using the neighbor-joining method. Proc Natl Acad Sci USA. 101:11030–11035.
- Wang JF, Hu C, Chen YC, Min JX, Li JT. 2002. Pupal stage with Aldrichina gtahami development form to deduce death time. Acta Ent Sin. 45:696–699.
- Xu H, Ye GY, Xu Y, Hu C, Zhu GH. 2014. Age-dependent changes in cuticular hydrocarbons of larvae in Aldrichina grahami (Diptera: Calliphoridae). Forensic Sci Int. 242:236–241.
- Yan J, Liao H, Xie K, Cai J. 2014a. The complete mitochondria genome of Chrysomya pinguis (Diptera: Calliphoridae). Mitochondrial DNA. 18:1–3. [Epub ahead of print].
- Yan J, Liao H, Zhu Z, Xie K, Guo Y, Cai J. 2014b. The complete mitochondria genome of Parasarcophaga similis (Diptera: Sarcophagidae). Mitochondrial DNA. 18:1–3. [Epub ahead of print].