Abstract
The white-eye birds of the genus Zosterops have been recognized for their high speciation rates in the past, but the relationships of the East African populations are not yet fully resolved. We sequenced and annotated mitogenomes of four populations currently assigned to three East African white-eye species, Zosterops senegalensis, Z. abyssinicus and Z. poliogaster. For Z. senegalensis specimens from two distant populations were sequenced; for the other taxa we used samples collected at one site. The mitogenomes ranged between 17,827 and 17,974 bp, in size similar to previously published mitogenomes analyzed for this genus from other geographic regions. The mitogenomes contain the classical set of 13 coding genes, two structural rRNA genes and 22 tRNA genes. We constructed a phylogeny using all complete mitogenomes currently available for the genus. The phylogeny supports an Asian or Oceanic origin of the genus Zosterops. The East African species represent a monophyletic clade, but the two specimens of Zosterops senegalensis from different regions do not group together, supporting previous hypotheses of cryptic species within the genus. The new genetic resources provided here may help to further explore the relationships and evolution of the genus.
Introduction
Mitogenomes provide important information for phylogenetic and phylogeographic studies. They are characterized by functional genes with a variety of different evolutionary rates and hence can be used for analyses of taxa with different divergent times (Curole & Kocher Citation1999; Jacobsen et al. Citation2012). They have been shown to be highly informative to resolve the basic taxonomic relationships within the Passerine birds (Barker Citation2014).
The Passerine bird genus Zosterops is an interesting target for evolutionary biology as it is characterized by high rates of speciation and a large number of endemic species, especially island endemics (Gill Citation1971; Moyle et al. Citation2009; Milá et al. Citation2010; Cornetti et al. Citation2015). In East Africa, only three species have been recognized as valid species for a long time (Moreau Citation1957), but more recently it has become evident that local populations are strongly differentiated from each other (genetically, phenotypically, specifically in regard of plumage coloration and bioacoustic traits) and hence should be treated as distinct taxa (Habel et al. Citation2013, Citation2014, Citation2015; Cox et al. Citation2014; Husemann et al. Citation2014, Citation2015). However, genetic resources for the group are still limited reducing phylogenetic resolution in previous studies.
More recently, several mitogenomes of Zosterops species have become available (Zosterops lateralis – Gibb et al. Citation2015; Zosterops erythropleurus – Li et al. Citation2016; Zosterops japonicus – Yang et al. Citationin press). However, so far no (mito)genome of an East African species was sequenced. Hence, we used a hybrid approach of Sanger Sequencing and Illumina MiSeq to generate whole mitochondrial genomes of four populations identified as three species of East African Zosterops species. We annotated the genomes and provide a phylogeny based on these and all other published mitogenome sequences of Zosterops.
Material and methods
We included 17 individuals of Z. senegalensis collected at two sites, Kakamega Forest (0°17’ N; 34°50’ E) (1 individual for Sanger sequencing and 7 individuals for Illumina MiSeq), and Mt. Nyeri (2°05’ N, 36°42’ E) (1 individual for Sanger sequencing and 8 individuals for Illumina MiSeq); 5 individuals of Z. poliogaster were collected in the Chyulu Hills (2°36’ S; 37°50’ E) (2 individuals for Sanger sequencing, 3 individuals for Illumina MiSeq); 12 individuals of Z. abyssinicus were collected at the foothills of the Chyulu Hills (2°25’ S; 37°55’ E) (with 2 individuals for Sanger sequencing and 10 individuals for Illumina MiSeq). Birds were caught with mist nets and a blood sample was taken and stored in 99% ethanol. The birds were subsequently released unharmed.
DNA was extracted from blood using the Qiagen DNeasy blood and tissue kit (Qiagen, Hilden, Germany) using the manufacturers protocol for blood. DNA extracts are stored at the Technical University Munich under the following internal accession numbers: Z. senegalensis – Kakamega Forest: T41, T42, T49, T50-T54; Mt. Nyeri: T58, T71-T76, T82, T83; Z. poliogaster: CH1, CH2-1, CH2-2, CH2-3, CH2-4; Z. abyssinicus: T05-T12, T17-20. PCRs were performed with standard protocols.
We initially sequenced 13 mitochondrial gene fragments using the primers provided by Amer et al. (Citation2013). Their primer set lacked primers for the Control Region and the primers for ND5, COII and COIII did not yield good results. The lack of primer binding was further confirmed by mapping all primers against a consensus sequence derived from 41 Passerine mitogenomes obtained from Genbank (, Accessions: KF509924, NC020429, JQ348398, NC015114, HQ690245, NC020427, JQ423933, NC015233, HQ896034, NC015196, HQ915865, NC015198, HQ915866, NC015232, HQ896033, NC015802, JN018411, NC015074, HQ690246, NC015898, JF937590, NC020423, JQ003191, JQ003192, NC015237, HQ896037, NC015613, JF810423, NC015195, HQ915864, NC020424, JQ083495, NC020426, JQ423932, NC015824, JN108020, KF509923, NC015810, JN018413, NC015200, HQ915867). Therefore, we designed a new set of 22 primer pairs based on the consensus sequence and the preliminary assemblies for Zosterops derived from the initial primer set (available from the authors upon request, ).
Figure 1. (A) Primer binding sites of all primers for Sanger sequencing used in this study (primer sequences available from the authors upon request) mapped against the consensus sequence of 41 published Passerine mitogenomes. (B) Annotated mitogenome of Zosterops poliogaster displaying gene order and composition; red - rRNA genes, pink - tRNA genes, yellow - coding genes, grey - Control Region.
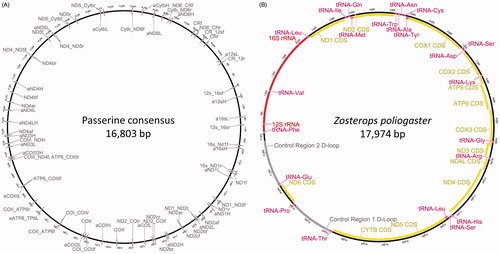
The newly generated sequences were trimmed and de-novo assembled using Geneious v. 6.1.8 (Kearse et al. Citation2012). For this, sequences from individuals belonging to the same populations were pooled. The resulting contigs were mapped against the published sequences of Z. lateralis (KC545407) to generate an initial assembly. The assemblies were further refined using Illumina MiSeq reads of specimens from identical populations (see above), which were generated for a different study with a new amplicon-based protocol that is described elsewhere (Meimberg et al. Citation2016). The final alignments were initially annotated using MitoAnnotator (Iwasaki et al. Citation2013) and Mitos (Bernt et al. Citation2013). The initial annotation was further refined by comparison to other published mitogenomes of Zosterops species (Z. lateralis – KC545407, Z. erythropleurus – KT194322, Z. japonicus – KT601061). Finally, we adjusted annotations of coding genes to implement stop codons, which were not implemented in the published mitogenomes for two of the genes.
We used the new mitogenomes together with published sequences to generate a phylogenetic tree with MrBayes v.3.2.6 (Ronquist et al. Citation2012). We included the newly generated sequences for Z. abyssinicus from the Chyulu Hills foothills, Z. poliogaster from the Chyulu Hills, and sequences for Z. senegalensis from Kakamega Forest and Mt. Nyeri. We further included all other available sequences for Zosterops species (Z. lateralis – KC545407, Z. erythropleurus – KT194322, Z. japonicus – KT601061) and used the consensus sequence of Passerines (see above) as outgroup. The best model of sequence evolution was determined as GTR + G by MrModeltest v.2 (Nylander Citation2004). We ran Mr Bayes for 10 million generations sampling every 1000 generations for a total of 10,000 sampled trees. Convergence was checked by the average split frequencies which were below 0.001. The tree was visualized using FigTree v.1.4.2 (http://tree.bio.ed.ac.uk/software/figtree/).
Results and discussion
We generated mitogenomic sequences for four populations of East African Zosterops: two populations of Z. senegalensis from Kakamega Forest and Mt. Nyeri, Z. poliogaster from the Chyulu Hills and Z. abyssinicus from the foothills of the Chyulu Hills. The four newly generated mitogenomes varied in size between 17,827 and 17,974 bp, similar to other mitogenomes published for this genus. They contain the classical set of 13 coding genes, two structural rRNA genes and 22 tRNA genes (). The tRNAs for Leucine and Serine were present in two copies. Similar to other Zosterops species we detected two Control Regions. The mitogenomic gene order was similar across all Zosterops which have been analyzed so far. The GC content varied between 44.5% for Z. senegalensis from Kakamega forest and 45.6% for Z. poliogaster. All newly generated sequences were deposited in NCBI GenBank (Accession Numbers: KX181885- KX181888).
Most relationships were strongly supported in our phylogenetic reconstruction (; posterior probabilities >0.95). Zosterops lateralis was the basal species in the phylogeny. Zosterops japonicus and Z. erythropleurus were sister taxa and represented the sister group to the East African species. This was the only relationship with no support (pp =0.53). This may be due to limited sampling or saturation effects. The Eastern African species were monophlyletic with maximum support. However, Zosterops senegalensis was polyphyletic with the population from Kakamega Forest being basal to all other species and the population from Mt. Nyeri being sister to Z. abyssinicus. Zosterops poliogaster was the sister taxon to this grouping.
Figure 2. Bayesian phylogenetic tree generated from all available Zosterops mitogenomes; black circles indicate posterior probabilities of 1.
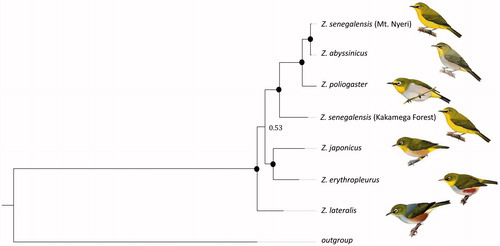
The phylogeny provides further support to previous findings in regard of the Asian or Oceanic origin of the genus (Moyle et al. Citation2009; Cox et al. Citation2014). Further, our findings support the non-monophyly of the currently established East African species of the genus, which had been suggested on the basis of morphological, acoustic, microsatellite and sequence data (Habel et al. Citation2013, Citation2014, Citation2015; Cox et al. Citation2014; Husemann et al. Citation2014, Citation2015).
Acknowledgement
The drawings of birds for were generously provided by the Handbook of the Birds of the World Alive del Hoyo et al. (Citation2014).
Disclosure statement
The authors declare no conflicts of interest. The authors alone are responsible for the content and writing of the paper.
Funding information
We would like to thank the DAAD for financial support to MH and JCH. The study was partially funded by a flexible pool grant to JCH and Wolfgang W. Weisser (TUM, Freising, Germany) of the German Centre of integrative Biodiversity Research (iDiv). This work was supported by the German Research Foundation (DFG) and the Technische Universität München within the funding programme Open Access Publishing.
References
- Amer SAM, Ahmed M, Shobrak M. 2013. Efficient newly designed primers for the amplification and sequencing of bird mitochondrial genomes. Biosci Biotechnol Biochem. 77:577–581.
- Barker FK. 2014. Mitogenomic data resolve basal relationships among passeriform and persseridan birds. Mol Phyl Evol. 79:313–324.
- Bernt M, Donath A, Jühling F, Externbrink F, Florentz C, Fritzsch G, Pütz J, Middendorf M, Stadler PF. 2013. MITOS: improved de novo Metazoan mitochondrial genome annotation. Mol Phylogenet Evol. 69:313–319.
- Cornetti L, Valente LM, Dunning LT, Quan X, Black RA, Hébert O, Savolainen V. 2015 . The genome of the “Great Speciator” provides insights into bird diversification. Genome Biol Evol. 7:2680–2691.
- Cox SC, Prys-Jones RP, Habel JC, Amakobe B, Day JJ. 2014. Niche divergence promotes rapid diversification of East African sky island white-eyes (Aves: Zosteropidae). Mol Ecol. 23:4103–4118.
- Curole J, Kocher T. 1999. Mitogenomics: digging deeper with complete mitochondrial genomes. Trends Ecol Evol (Amst.). 14:394–398.
- del Hoyo J, Elliott A, Sargatal J, Christie DA, de Juana E. 2014. Handbook of the birds of the world alive. Barcelona: Lynx Edicions. Retrieved from http://www.hbw.com/on
- Gibb GC, England R, Hartig G, McLenachan PA, Taylor Smith BL, McComish BJ, Cooper A, Penny D. 2015. New Zealand passerines help clarify the diversification of major songbird lineages during the oligocene. Genome Biol Evol. 7:2983–2995.
- Gill FB. 1971. Ecology and evolution of the sympatric Mascarene white-eyes, Zosterops borbonica and Zosterops olivacea. Auk. 88:35–60.
- Habel JC, Cox S, Gassert F, Mulwa RK, Meyer J, Lens L. 2013. Population genetics of the East African white-eye species complex. Conserv Genet. 14:1019–1028.
- Habel JC, Mulwa RK, Gassert F, Rödder D, Ulrich W, Borghesio L, Husemann M, Lens L. 2014. Population signatures of large-scale, long-term disjunction and small-scale, short-term habitat fragmentation in an Afromontane forest bird. Heredity. 113:205–214.
- Habel JC, Borghesio L, Newmark WD, Day JJ, Lens L, Husemann M, Ulrich W. 2015. Evolution along the Great Rift Valley: phenotypic and genetic differentiation of East African white-eyes (Aves, Zosteropidae). Ecol Evol. 5:4849–4862.
- Husemann M, Ulrich W, Habel JC. 2014. The evolution of contact calls in isolated and overlapping populations of two white-eye congeners in East Africa (Aves, Zosterops). BMC Evol Biol. 14:115.
- Husemann M, Cousseau L, Borghesio L, Lens L, Habel JC. 2015. Effects of population size and isolation on the genetic structure of the East African mountain white-eye Zosterops poliogaster (Aves). Biol J Linn Soc. 114:828–836.
- Iwasaki W, Fukunaga T, Isagozawa R, Yamada K, Maeda Y, Satoh TP, Sado T, Mabuchi K, Takeshima H, Miya M, Nishida M. 2013. MitoFish and MitoAnnotator: a mitochondrial genome database of fish with an accurate and automatic annotation pipeline. Mol Biol Evol. 30:2531–2540.
- Jacobsen MW, Hansen MM, Orlando L, Bekkevold D, Bernatchez L, Willerslev E, Gilbert TP. 2012. Mitogenome sequencing reveals shallow evolutionary histories and recent divergence time between morphologically and ecologically distinct European whitefish (Coregonus spp.). Mol Ecol. 21:2727–2742.
- Kearse M, Moir R, Wilson A, Stones-Havas S, Cheung M, Sturrock S, Buxton S, Cooper A, Markowitz S, Duran C, et al. 2012. Geneious basic: an integrated and extendable desktop software platform for the organization and analysis of sequence data. Bioinformatics. 28:1647–1649.
- Li YM, Yao JY, Zhao X, Li LY, Yan SQ. 2016. Complete mitochondrial genome sequence of Chestnut-flanked white-eye (Zosterops erythropleurus). Mitochondrial DNA A DNA Mapp Seq Anal. 27:3529–3530.
- Meimberg H, Schachtler C, Curto M, Husemann M, Habel JC. 2016. A new amplicon based approach of whole genome sequencing for phylogenetic and phylogeographic analysis: an example of East African white-eyes (Aves: Zosteropidae). Mol Phylogenet Evol. 102:74–85. dx.doi.org/10.1016/j.ympev.2016.05.023
- Milá B, Warren BH, Heeb P, Thébaud C. 2010. The geographic scale of diversification on islands: genetic and morphological divergence at a very small spatial scale in the Mascarene grey white-eye (Aves: Zosterops borbonicus). BMC Evol Biol. 10:158.
- Moreau RE. 1957. Variation in the western Zosteropidae (Aves). Bull Br Mus. 4:318–433.
- Moyle RG, Filardi CE, Smith CE, Diamond J. 2009. Explosive pleistocene diversification and hemispheric expansion of a ‘great speciator’. Proc Nat Acad Sci Am. 106:1863–1868.
- Nylander JAA. 2004. MrModeltest version 2. Program distributed by the author. Uppsala: Evolutionary Biology Centre, Uppsala University.
- Ronquist F, Teslenko M, van der Mark P, Ayres DL, Darling A, Höhna S, Larget B, Liu L, Suchard MA, Huelsenbeck JP. 2012. MrBayes 3.2: efficient Bayesian phylogenetic inference and model choice across a large model space. Syst Biol. 61:1–4.
- Yang D-C, Sun Y, Lu C-H. in press. The complete mitochondrial genome of Zosterops japonicas. Mitochondrial DNA. DOI: 10.3109/19401736.2015.1101581.