Abstract
The complete mitochondrial genome (mitogenome) was determined for the longfin dragonfish Tactostoma macropus, which is the first for the genus and the third within the family Stomiidae. The mitogenome sequence is 17,690 bp in length containing 2 ribosomal RNA genes, 22 transfer RNA genes, 13 protein-coding genes, and a control region, as in most fishes. The gene order of T. macropus showed an unreported deviation from the typical vertebrate one. Phylogenetic reconstruction using the maximum likelihood method placed T. macropus in the monophyletic Stomiiformes. Three stomiid species were recovered as a moderately supported clade in the phylogenetic tree.
The barbeled dragonfishes of the family Stomiidae (Stomiiformes) are representative deep-sea pelagic predators (Kenaley Citation2012). Stomiid taxonomy has attracted considerable attention due to the rarity of these fishes. In addition, the remarkable phenotypical and ecological specialization of these species to life in dark waters includes the use of not only blue but also far-red bioluminescence to communicate (e.g. Kenaley et al. Citation2014). Phylogenetic analysis based on mitochondrial genome (mitogenome) data would improve our understanding of the evolutionary history of stomiids. However, stomiid mitogenomes are available for only 2 – Chauliodus sloani and Stomias atriventer – of the more than 280 species (Miya et al. Citation2001; Aguilar et al. Citation2018). In the current study, we determined the complete mitochondrial DNA sequence of the longfin dragonfish Tactostoma macropus; this is the first complete mitogenome for the genus and the fifth within the order Stomiiformes.
A beam-trawl haul yielded a single T. macropus at station KANO4 of the R/V Shinsei-maru cruise KS-17-6 (off Otsuchi, Iwate, Japan: 39°24.97′–39°25.00′N, 143°14.48′–143°19.58′E; depth, 2,161–2,414 m). The specimen was stored at −30 °C until dissection, and then fixed with 99% ethyl alcohol; vouchered DNA (171127_DNA1) was deposited at the Atmosphere and Ocean Research Institute, The University of Tokyo. Total genomic DNA was extracted from the photophore under the right eye, and paired-end sequencing (2 × 300 bp) was performed in an Illumina MiSeq sequencer. Bases with a quality score lower than 20 were removed from sequence reads, and then trimmed reads shorter than 127 bp were discarded using Sickle (Joshi and Fass Citation2011). Remaining reads were assembled using SPAdes version 3.10.1 (Bankevich et al. Citation2012). The assembled mitogenome sequence was annotated using the MitoFish website (Iwasaki et al. Citation2013); some annotations were corrected manually.
The complete mitogenome sequence of T. macropus (DDBJ/EMBL/GenBank accession no. LC377784) is 17,690 bp in length containing 2 ribosomal RNA (12S and 16S) genes, 22 transfer RNA genes (tRNAs), 13 protein-coding genes, and a control region (CR, D-loop). Tactostoma macropus shows a deviation from the typical vertebrate gene order in the Cyt b-tRNAThr-tRNAPro-CR region: gene rearrangement resulted in its specific order of tRNAPro-CR-Cyt b-tRNAThr (see Satoh et al. Citation2016). In addition, noncoding sequences are present between tRNAGlu and tRNAPro (217 bp) and between tRNAThr and tRNAPhe (151 bp). All protein-coding genes but COI (GTG) and ATP6 (ATT) use ATG as the start codon; TAA, TAG, TA–, T––, AGG, and AGA are found as stop codons. The ATT start codon of ATP6 does not occur in 250 previously determined fish mitogenomes (Satoh et al. Citation2016). The overall base composition is 25.1% for A, 24.5% for T, 31.4% for G, and 19.0% for C.
Partitioned maximum likelihood analysis placed T. macropus in the monophyletic Stomiiformes (bootstrap percentage [BS] = 100%; ). The family Stomiidae was recovered as a moderately supported clade (BS = 63%), although a recent mitogenomic phylogeny has suggested its non-monophyly (Aguilar et al. Citation2018). Further investigation with dense taxonomic sampling will shed new light on the phylogenetic status of the family.
Figure 1. Maximum likelihood (ML) phylogeny of 10 teleost fishes according to the concatenated amino-acid sequences of 13 protein-coding genes (3784 positions). Tactostoma macropus is shown in bold. Sequences were aligned separately for each gene using MAFFT version 7.047 (Katoh and Standley Citation2013) with default parameters. Ambiguously aligned positions were removed using Gblocks Server version 0.91b (Castresana Citation2000), with all options for less stringent selection. ML analysis was performed in RAxML version 7.2.6 (Stamatakis Citation2006) using mtREV + G model; nodal support was estimated by 1000 bootstrap replicates. DDBJ/EMBL/GenBank accession numbers are shown for published sequences.
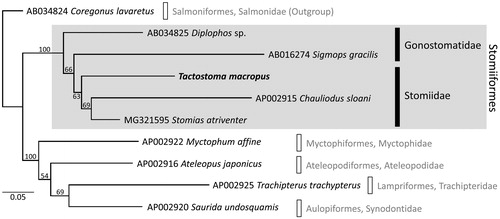
Acknowledgements
We thank the captain, crew, and researchers of the R/V Shinsei-maru cruise KS-17-6, which is part of the research project Tohoku Ecosystem-Associated Marine Sciences (TEAMS) funded by the Ministry of Education, Culture, Sports, Science, and Technology. Sequencing was contributed by Bioengineering Lab. Co., Ltd., Japan. We also thank Dr. H. Fukumori (AORI, UT) for providing invaluable comments, which improved the manuscript.
Disclosure statement
No potential conflict of interest was reported by the authors.
References
- Aguilar A, Truong BR, Gutierrez F. 2018. Complete mitochondrial DNA genomes for two northeast Pacific mesopelagic fishes, the Mexican lampfish (Triphoturus mexicanus) and black-belly dragonfish (Stomias atriventer). Mitochondrial DNA B Resour. 3:21–23.
- Bankevich A, Nurk S, Antipov D, Gurevich A, Dvorkin M, Kulikov AS, Lesin V, Nikolenko S, Pham S, Prjibelski A, et al. 2012. SPAdes: a new genome assembly algorithm and its applications to single-cell sequencing. J Comput Biol. 19:455–477.
- Castresana J. 2000. Selection of conserved blocks from multiple alignments for their use in phylogenetic analysis. Mol Biol Evol. 17:540–552.
- Iwasaki W, Fukunaga T, Isagozawa R, Yamada K, Maeda Y, Satoh TP, Sado T, Mabuchi K, Takeshima H, Miya M, et al. 2013. MitoFish and MitoAnnotator: a mitochondrial genome database of fish with an accurate and automatic annotation pipeline. Mol Biol Evol. 30:2531–2540.
- Joshi NA, Fass JN. 2011. Sickle: a sliding-window, adaptive, quality-based trimming tool for FastQ files (Version 1.33) [Software]. https://github.com/najoshi/sickle.
- Katoh K, Standley DM. 2013. MAFFT multiple sequence alignment software version 7: improvements in performance and usability. Mol Biol Evol. 30:772–780.
- Kenaley CP. 2012. Exploring feeding behaviour in deep-sea dragonfishes (Teleostei: Stomiidae): jaw biomechanics and functional significance of a loosejaw. Biol J Linn Soc. 106:224–240.
- Kenaley CP, DeVaney SC, Fjeran TT. 2014. The complex evolutionary history of seeing red: molecular phylogeny and the evolution of an adaptive visual system in deep-sea dragonfishes (Stomiiformes: Stomiidae). Evolution. 68:996–1013.
- Miya M, Kawaguchi A, Nishida M. 2001. Mitogenomic exploration of higher teleostean phylogenies: a case study for moderate-scale evolutionary genomics with 38 newly determined complete mitochondrial DNA sequences. Mol Biol Evol. 18:1993–2009.
- Satoh TP, Miya M, Mabuchi K, Nishida M. 2016. Structure and variation of the mitochondrial genome of fishes. BMC Genomics. 17:719.
- Stamatakis A. 2006. RAxML-VI-HPC: maximum likelihood-based phylogenetic analyses with thousands of taxa and mixed models. Bioinformatics. 22:2688–2690.