Abstract
The western flower thrips, F. occidentalis is a vector of Tospoviruses and native to Western North America and Mexico. The present study is based on collected F. occidentalis specimens from Karnataka state in southern India and morphologically identified through available keys. The generated DNA barcode data show 99–100% similarity with the database sequences of F. occidentalis. The phylogenetic analysis (NJ, ML, and BA) shows three distinct clades of F. occidentalis in the present dataset with high bootstrap supports and posterior probabilities. The K2P genetic distances further depicted high similarity of the generated sequences from India and Netherlands. The Clade-1 (India + Netherlands) also shows a close relationship with Clade-2 (Kenya) rather than Clade-3 (Canada + USA). This study recorded the first genetic footprint of F. occidentalis in India and indicated the gene flow from the Netherlands to India. The similar molecular techniques may help to detect the invasion of many alien species in the near future and assists the quarantine regulations to protect the native ecosystem.
1. Introduction
The small, minute organism with fringed wing insects are known as thrips and belong to order Thysanoptera. Globally, near about 6154 species are reported, out of these, only 1% are reported as pests and vectors of Tospoviruses (ThripsWiki Citation2018). Among global thrips diversity, India shares 12% with 739 species under 5 families (Tyagi and Kumar Citation2016). Thrips are the sole transmitters of Tospoviruses and causing plant disease in several plant families across different zoogeography. Till now, only 15 species are known as vectors of plant Tospoviruses (family Bunyaviridae) (Riley et al. Citation2011, Zhou and Tzanetakis Citation2013). Thus, concerning their impact on the global agricultural economy, accurate species identification is the basic need for implementation of effective integrated pest management strategies.
The western flower thrips, F. occidentalis was native to Western North America and Mexico (Mound and Marullo Citation1996). In the recent past, the species has turned out to be one of the major pests worldwide by making a severe economic loss in many agricultural and horticultural crops (Wijkamp et al. Citation1995; Kirk Citation2002; Kirk and Terry Citation2003; Riley et al. Citation2011). This species can transmit five different Tospovirus strains, viz., Tomato spotted wilt virus, Impatiens necrotic spot virus, Groundnut ringspot virus, Chrysanthemum stem necrosis virus, and Tomato chlorotic spot virus (Whitfield et al. Citation2005). Globally, around $1 billion economic loss was estimated by a single Tospovirus, TSWV and $50 million loss from Netherland (Rugman-Jones et al. Citation2010). Further, the rigorous estimation revealed that a total of $326 million losses was detected by Tospoviruses from 1996 to 2006 in Georgia (Riley et al. Citation2011). Recently, F. occidentalis was morphologically detected from Karnataka state in southern India (Tyagi and Kumar Citation2015). This species exhibits color polymorphism, ranging from yellow, bicolored to brown which are morphologically similar but genetically different (Rugman-Jones et al. Citation2010). The cryptic behavior, sexual dimorphism, and polymorphism phenomenon are common in thrips species (Tyagi et al. Citation2017) that required molecular interventions for accurate species identification and delimitations. Further, DNA barcoding studies revealed multiple cryptic species in Frankliniella schultzei (Tyagi et al. Citation2017). Thus, the present study was aimed to determine the identity of F. occidentalis through molecular data to adjudicate the possible invasion.
2. Materials and methods
2.1. Sampling, vouchering and molecular assessment
Total 11 specimens of F. occidentalis were collected from three different localities (16°12′N 74°43′E, 14°21′N 76°03′E, 13°03′N 78°12′E) in Karnataka state of southern India (). The specimens were collected by standard beating methods from different host plants (bitter gourd, watermelon, cucumber, and pumpkin) and preserved in 70% ethanol and subsequently stored at −20 °C for DNA analysis. After non-destructive DNA isolation, the specimens were retrieved and mounted onto glass slides in Canada balsam for morphological identification. The specimens were identified by available morphological keys (Mound and Marullo Citation1996; Cavalleri and Mound Citation2012). The photographs were taken through a Leica Trinocular Microscope (Leica DM-1000) and using a Leica software application suite (LAS EZ 2.1.0). The voucher specimens were deposited in National Zoological Collections of Zoological Survey of India with the sample code and registration No. TH-2266 (9310/H17), TH-2275 (9314/H17), TH-2285 (9313/H17), TH-2318 (9315/H17), TH-2230 (9311/H17), TH-2314 (9312/H17), TH-2340 (9391/H17), TH-2339 (9392/H17), TH-2338 (9393/H17), TH-2336 (9422/H17), and TH-2337 (9433/H17).
Figure 1. (A) Map showing the collection localities of F. occidentalis in Karnataka states of southern India with latitude and longitude. (B) Bayesian (BA) phylogeny of the studied F. occidentalis species. The BA posterior probability, and bootstrap support resulted in NJ and ML tree were superimposed with each nodes. The collection localities of the studied and database sequences were incorporated with the voucher IDs and accession numbers in the phylogeny. Colour bars show the genetic variability and distinct clades of the F. occidentalis population.
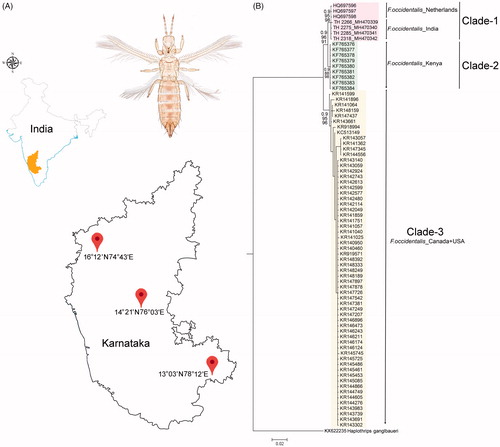
The genomic DNA was extracted using the QIAamp DNA Mini Kit (Qiagen, Valencia, CA). An intersegmental abdominal cut was made to only four specimens and lysed overnight at 56 °C in buffer ATL with proteinase-K. The polymerase chain reaction (PCR) was performed to amplify the mitochondrial DNA barcode gene segment (∼648 bp) for molecular-based species identification. The published primer pair, HCO-2198: 5′-TAAACTTCAGGGTGACCAAAAAATCA-3′ and LCO-1490: 5′-GGTCAACAAATCATAAAGATATTGG-3′ was used to amplify the targeted mitochondrial region (Folmer et al. Citation1994). The total volume of PCR reaction was 30 μl containing 20 Picomoles of each primer, 20 mM Tris-HCl (pH 8.0), 100 mM KCl, 0.1 mM EDTA, 1 mM DTT, 1.8 mM MgCl2, 0.25 mM of each dNTP, and 1U of Taq polymerase (Takara BIO Inc., Shiga, Japan) with the following thermal profile: 5 min at 94 °C; followed by 40 cycles of 30 s at 94 °C, 40 s at 49 °C, 1 min at 72 °C, and final extension for 5 min at 72 °C. The PCR products were checked in 1% agarose gel, stained with ethidium bromide (10μg/mL). The PCR products were purified using the QIAquick Gel Extraction Kit (Qiagen) following the manufacturer’s protocols. The cycle sequencing of the purified PCR products was performed with BigDye®Terminator ver. 3.1 Cycle Sequencing Kit (Applied Biosystems, Inc. California, US) by 48 capillary ABI 3730 Genetic analyzer, housed at the Zoological Survey of India, Kolkata.
2.2. Sequence analysis and dataset preparation
The bi-directional chromatograms of each specimen were checked in MEGA6 (Tamura et al. Citation2013) and the noisy parts were trimmed at both the ends. Each sequence was evaluated in the nucleotide BLAST (BLASTn) online program (https://blast.ncbi.nlm.nih.gov/Blast.cgi) and ORF finder (http://www.ncbi.nlm.nih.gov/gorf/gorf.html) to examine the complete alignment and stop codons. Primarily, the generated sequences were identified in the online identification system, in GenBank with ‘Highly similar sequences (megablast)’ and BOLD databases with ‘All Barcode Records on BOLD’. Based on the similarity search and considering the sequence length (>500bp), total 73 sequences of F. occidentalis were acquired from the GenBank database to make a final dataset. The final dataset of 77 sequences was aligned using ClustalX software and finally, 514 bp of mtCOI were adopted for estimating genetic divergence and phylogeny (Thompson et al. Citation1997). The sequence of Haplothrips ganglbaueri (KX622235) was further incorporated in the dataset as an out-group.
2.3. Genetic divergence, haplotypes, and phylogeny
The genetic divergence was calculated by Kimura 2 parameter (K2P) in MEGA6 (Tamura et al. Citation2013). The model selection was based on the Bayesian Information Criterion (BIC) computed by PartitionFinder version 1.1.1 (Lanfear et al. Citation2012). The best fit model GTR + I + G (NST = 6) was selected for both ML and BA phylogeny. The Neighbor-Joining (NJ), Maximum Likelihood (ML), and Bayesian analysis (BA) were implemented to test the reciprocal monophyletic criteria for species identification. The NJ and ML tree were performed under the optimality criteria by using PAUP 4.0b10 (Swofford Citation2002) with 1000 bootstrap support. For BA, Markov Chain Monte Carlo (MCMC) was performed with four chains for 1,000,000 generations, with trees sampled every 100 generations (the first 1000 trees were discarded as ‘burn-in’) using MrBayes 3.1 (Ronquist and Huelsenbeck Citation2003). MCMC analysis was stationary when the maximum standard deviation of split frequencies reached below 0.01 and potential scale reduction factor approached 1.0.
3. Results and discussion
The collected thrips specimens were identified as F. occidentalis (female) with the following diagnostic characters: body pale yellow with brown patches on abdominal tergites; head with three pairs of ocellar setae, pair III situated between fore and hind ocelli; postocular setae IV longer than others. Eyes without pigmented facets. Antenna with 8-segments with simple pedicle on segment III, segments III and IV each with forked sense cone. Pronotum with two to four small setae between the major anteromarginal setae. Mesonotal surface with faint transverse line, anteromedian campaniform sensilla present. Metanotal median setae situated at the anterior margin, with irregular reticulations postero-medially and paired campaniform sensilla. Fore wing first vein and second vein with complete rows of setae. Abdominal tergite VIII with an irregular comb of microtrichia at their posterior margin. Tergite IX S1 setae longer than tergite X.
DNA barcoding is evidenced as an effective molecular tool for (i) species identification (Hebert et al. Citation2003; Iftikhar et al. Citation2016; Laskar et al. Citation2018), (ii) recognition of the species complexes and cryptic diversity (Tyagi et al. Citation2017; Kundu et al. Citation2018), (iii) estimation of the phylogeny and evolutionary relationship of species (Buckman et al. Citation2013; Kundu et al. Citation2016; Tyagi et al. Citation2017; Laskar et al. Citation2018), and (iv) detection of invasive species and their probable route (Tyagi et al. Citation2015; Kundu et al. Citation2016) and resolve several biological questions in a wide variety of animal taxa. The generated DNA barcode sequences of the four specimens were annotated (616bp) and submitted into the global database (GenBank) to acquire the unique accession numbers (TH-2266: MH470339, TH-2275: MH470340, TH-2285: MH470341, and TH-2318: MH470342). The sequence similarity search in both BLASTn and BOLD-IDs revealed definitive identity matches (99–100%) for all four generated sequences with the database sequences of F. occidentalis. The estimated phylogenetic tree (NJ, ML, and BA) depicted cohesive clustering of the identified F. occidentalis sequences with the database sequences with high bootstrap support and posterior probabilities (). The phylogenetic analysis shows three distinct clades of F. occidentalis in the present dataset: Clade-1, Clade 2, and Clade-3 (). The Clade-1 shows the cohesive clustering of the F. occidentalis sequences, generated from India with the database sequences from the Netherlands. Further, the Clade-2 and Clade-3 represented by the database sequences of F. occidentalis generated from Kenya and Canada respectively. One database sequence, generated from the USA, shows cladding with the Clade-3. The present phylogeny also depicted the close relationship of Clade-1 (India + Netherlands) with Clade-2 (Kenya) rather than Clade-3 (Canada + USA) (). The overall mean genetic distance within the studied dataset of F. occidentalis was 1.5% ranging from 0% to 4.7%. The F. occidentalis sequences generated from Canada and India shows 0.4% and 0.1% conspecific genetic divergence respectively. Further, the F. occidentalis sequences generated from the Netherlands and Kenya shows 0% genetic divergence within the species and cannot be calculated for a single sequence generated from the USA. The generated sequences of F. occidentalis from India is identical in the genetic distance (0%) with the sequences generated from the Netherlands. Further, the Clade-1 (India + Netherlands) shows 1–3.9% genetic divergence with other F. occidentalis sequences generated from Kenya (Clade-2), and Canada + USA (Clade-3). ().
Table 1. The K2P genetic divergence over sequence pairs between and within groups of the studied F. occidentalis species.
Hence, based on the molecular data, the present study assumed the flow of genetic material, and the European country (Netherlands) may be a probable source of invasion of F. occidentalis to India (South Asian countries). The genetic distance and phylogeny revealed the close relationship of European and South Asian populations of F. occidentalis with the African populations. The invasion of biological taxa in the non-native ecosystem is well-known biotic threats for indigenous animal or plant species. Furthermore, the trading of agricultural resources within and between the different countries facilitates to maintain economic growth. However, a thorough inspection is necessitated while trading of any agricultural resources and possible associated organisms to mitigate the invasion of alien species and protect the ecosystem. In this circumstance, the concern quarantine agencies may adopt the molecular tools for detecting the organisms at any life stages or at any forms. In the recent past, scientists have evidenced the invasion of the serious pest species of Papaya, Thrips parvispinus from Indonesia to India (Tyagi et al. Citation2015). In this study, we recorded the genetic footprint of another serious pest and vector species, F. occidentalis from India. In conclusion, more molecular data of this serious pest species from different geographical regions and their population genetics may be helpful to trace the exact route of invasion.
Acknowledgements
The authors thank Director, Dr. Kailash Chandra, Zoological Survey of India, Ministry of Environment, Forest and Climate Change for providing necessary facilities.
Disclosure statement
No potential conflict of interest was reported by the authors.
Additional information
Funding
References
- Buckman RS, Mound LA, Whiting MF. 2013. Phylogeny of thrips (Insecta: Thysanoptera) based on five molecular loci. Syst Entomol. 38:123–133.
- Cavalleri A, Mound LA. 2012. Toward the identification of Frankliniella species in Brazil (Thysanoptera, Thripidae). Zootaxa. 3270:1–30.
- Folmer O, Black M, Hoeh W, Lutz R, Vrijenhoek R. 1994. DNA primers for amplification of mitochondrial cytochrome c oxidase subunit I from diverse metazoan invertebrates. Mol Mar Biol Biotech. 3:294–299.
- Hebert PD, Cywinska A, Ball SL, deWaard JR. 2003. Biological identifications through DNA barcodes. Philos Trans R Soc Lond B Biol Sci. 270:313–322.
- Iftikhar R, Ashfaq M, Rasool A, Hebert PDN. 2016. DNA barcode analysis of thrips (Thysanoptera) diversity in Pakistan reveals cryptic species complexes. PLoS One. 11:e0146014.
- Kirk WDJ, Terry LI. 2003. The spread of the Western Flower thrips Frankliniella occidentalis (Pergande). Agric Forest Ent. 5:301–310.
- Kirk WDJ. 2002. The pest and vector from the West: Frankliniella occidentalis. In: Marullo R, Mound LA, editors. Thrips and Tospoviruses: Proceedings of the 7th International Symposium on Thysanoptera. Canberra (Australia): Australian National Insect Collection; p. 33–44.
- Kundu S, Kumar V, Laskar BA, Chandra K, Tyagi K. 2016. Mitochondrial DNA effectively detects non-native Testudines: invisible wildlife trade in northeast India. Gene Reports. 4:10–15.
- Kundu S, Kumar V, Laskar BA, Tyagi K, Chandra K. 2018. Investigating genetic divergence in subspecies of Manouria emys: distinct lineages or plastron anomalies?. Mitochondrial DNA B Resour. 3:166–170.
- Lanfear R, Calcott B, Simon Y, Ho W, Stephane GS. 2012. PartitionFinder: combined selection of partitioning schemes and substitution models for phylogenetic analyses. Mol Biol Evol. 29:1695–1701.
- Laskar BA, Kumar V, Kundu S, Tyagi K, Chandra K. 2018. Taxonomic quest: validating two mahseer fishes (Actinopteri: Cyprinidae) through molecular and morphological data from biodiversity hotspots in India. Hydrobiologia. 815:113–124.
- Mound LA, Marullo R. 1996. The thrips of Central and South America: an introduction. Mem Am Entomol Inst. 6:1–486.
- Riley DG, Joseph SV, Srinivasan R, Dife S. 2011. Trips vectors of tospoviruses. J Int Pest Manage. 1:1–10.
- Ronquist F, Huelsenbeck JP. 2003. MrBayes3: Bayesian phylogenetic inference under mixed models. Bioinformatics. 19:1572–1574.
- Rugman-Jones PF, Hoddle MS, Stouthamer R. 2010. Nuclear-mitochondrial barcoding exposes the global pest western flower thrips (Tysanoptera: Tripidae) as two sympatric cryptic species in its native California. J Econ Entomol. 103:877–886.
- Swofford DL. 2002. PAUP*. Phylogenetic Analysis Using Parsimony (*and Other Methods). Version 4. Sunderland (MA): Sinauer Associates.
- Tamura K, Stecher K, Peterson D, Filipski K, Kumar S. 2013. MEGA6: Molecular Evolutionary Genetics Analysis version 6.0. Mol Biol Evol. 30:2725–2729.
- Thompson JD, Gibson TJ, Plewniak F, Jeanmougin F, Higgins DG. 1997. The CLUSTAL_X windows interface: flexible strategies for multiple sequence alignment aided by quality analysis tools. Nucleic Acids Res. 25:4876–4882.
- ThripsWiki. 2018. Thrips Wiki - providing information on the World’s thrips; [accessed May 2018]. Available from: http://thrips.info/wiki.
- Tyagi K, Kumar V. 2015. First report of Western Flower Thrips, Frankliniella occidentalis (Pergande) (Thripidae: Thysanoptera) from India-potential havoc to Indian agriculture. Halteres. 6:1–3.
- Tyagi K, Kumar V. 2016. Thrips (Insecta: Thysanoptera) of India: an updated checklist. Halteres. 7:64–98.
- Tyagi K, Kumar V, Singha D, Chakraborty R. 2015. Morphological and DNA barcoding evidence for invasive pest Trips, Trips parvispinus (Tripidae: Tysanoptera), newly recorded from India. J Insect Sci. 15:105.
- Tyagi K, Kumar V, Singha D, Chandra K, Laskar BA, Kundu S, Chakraborty R, Chatterjee S. 2017. DNA barcoding studies on thrips in India: cryptic species and species complexes. Sci Rep. 7:4898.
- Whitfield AE, Ullman DE, German TL. 2005. Tospovirus-thrips interactions. Annu Rev Phytopathol. 43:459–489.
- Wijkamp I, Almarza R, Goldbach R, Peters D. 1995. Distinct levels of specificity in thrips transmission of tospoviruses. Phytopathology. 85:1069–1074.
- Zhou J, Tzanetakis IE. 2013. Epidemiology of soybean vein necrosis-associated virus. Phytopathology. 103:966–971.