Abstract
Mitochondria can produce the energy currency of the cell through respiration which would be affected by variation in mitochondrial DNA (mtDNA). We sequenced MT-ND3 and MT-ND4L genes in 51 Tibetan yaks, 59 Tibetan cattle, and 60 Holstein-Friesian. And the results showed that the SNPs (m.9893 A > G, m.9932 A > C, and m.10155 C > T) of MT-ND3 had negative associations with high-altitude adaptation (p < .003), while the SNP m.10073C > T was positively associated with high-altitude adaptation (p < .0006). Haplotypes H1 and H5 in MT-ND3 and Ha1 in MT-ND4L showed positive associations with high-altitude adaptability, while haplotype H3 and Ha3 negatively associated with this adaptability (p < .0014, p < .0017).
Introduction
Qinghai-Tibetan Plateau is the world’s highest plateau with altitude ranging from 3000 to 5000m and characterized by rigorous environmental features like coldness, strong UV, windiness, and hypoxia. Modern domestic yaks were firstly domesticated in Tibet and there are more than 14 million yaks, supplying food and transport means, inhabit there. Specifically, yak’s dung can be used as fuel. Except for Tibetan yak, there are nearly one million indigenous cattle called Tibetan cattle that can also adapt to the hypoxic environment (Yan-Iing et al. Citation2011). It has been suggested that cattle from other places are susceptible to hypertension and heart failure when exposed to high-altitudes environment (Weir et al. Citation1974), which attributed to cattle’s chromosomal composition (Newman et al. Citation2011).
Mitochondria, known as the ‘energy factory’ of all eukaryotic cells (Reed et al. Citation1998), provide more than 95% of cellular energy via oxidative phosphorylation (OXPHOS) and this process involves oxygen-consuming and adenosine triphosphate (ATP) production (Scott et al. Citation2011). However, mitochondrial damage can affect cell structure (Harri et al. 2002) and accelerate apoptosis (Leonard and Schapira Citation2000). Mitochondria could not function properly without adequate supply and enough intracellular partial-pressure of oxygen (Gnaiger Citation2003). And hypoxia-related changes will impair mitochondria function such as energy generation and consumption processes (Coskun et al. Citation2003), indicating that mitochondria play an important part in hypoxia-induced cell and tissue damage as well as hypoxia acclimatization (Mishmar et al. Citation2003). Mammalian mitochondrial genome include 37 genes, which encode subunits for OXPHOS, electron transport, and mitochondrial protein synthesis. Mitochondrial genes ND1-6 and ND4L encoding 7 of 46 polypeptides of complex I are necessary for cellular respiration and OXPHOS (Nakamaru-Ogiso et al. Citation2010; Consortium Citation2015). Specifically, ND3 subunits are required to catalyze NADH dehydrogenation and electron transfer to ubiquinone (coenzyme Q10) (Consortium Citation2015). Previous research reported that polymorphisms in mitochondrial DNA including MT-ND3 could result in neuro-psychiatric disorders, such as Alzheimer's disease (Ghezzi et al. Citation2005), mitochondrial encephalomyopathy, lactic acidosis, stroke-like episodes (MELAS) (Wang et al. Citation2014; Mukai et al. Citation2015), Leigh's syndrome (LS) (Sarzi et al. 2007; Nesbitt et al. Citation2012) and Leber's hereditary optic neuropathy (LHON) (Adam et al. Citation2003; La et al. Citation2014). MT-ND4L is also one of the seven mitochondrially encoded subunits of complex I. Likewise, ND4L also had an effect on LHON (Yu et al. Citation2005). Flaquer et al. (2014) found, for the first time, a significant association between MT-ND4L (mt 10550A-G) and BMI. Because the two genes are necessary for normal assembly of complex I, mutations in either MT-ND3 or MT-ND4L might affect normal respiration of animals. In order to better understand the mechanisms of hypoxia adaptability, we compared the nucleotide composition, genetic diversity, variations, and haplotypes of MT-ND3 and MT-ND4L between high-altitude animals (Tibetan yak and cattle) and low-altitude cattle (Holstein-Friesian).
Materials and methods
Ear tissue samples collected from 51 Tibetan yak (Bos mutus) and 59 Tibetan cattle (Bos taurus) inhabiting in the Tibetan Plateau with an average elevation exceeding 4500 m and 60 Holstein-Friesian (inhabiting in Sichuan basin with average 250–750 m altitude) were immediately put into 1.5 ml EP tubes containing 1 ml of ethanol and stored at −20 °C. All experimental procedures were approved by the Institutional Animal Care and Use Committee of Sichuan Agricultural University, China. Primers (F: AACCACCACTTCGGCTTTC and R: CGGCGTTTAGGCGTTCT) for Cattle based on a complete mitochondrial genome sequence of B. taurus (GenBank Accession Number V00654). The two genes for Tibetan yak were amplified using the primer F: CATTTCACTTCTAACCACCAC and R: TGAGGCTATTGTCACCAA according to the sequence of B. mutus (GenBank Accession Number KM233417), which was then used as the reference sequence to determine the variation sites of cattle MT-ND3 and MT-ND4L genes. The PCR procedure was conducted according to a previous study in our lab (Shi et al. Citation2018). All PCR products were sequenced by Chengdu Qingke Biotechnology Company, Ltd. (Chengdu, Sichuan, China). All sequences were edited and aligned with the reference sequence by using DNASTAR SeqMan software (DNASTAR Inc. Madison, WI). Sequence variations were identified using the software MEGA 6.0 (http://www.megasoftware.net). Haplotype diversity (Hd), average number of differences (K), and nucleotide diversity (p) of the MT-ND3 and MT-ND4L genes was determined using the software DnaSP V5 (http://www.ub.edu/dnasp/). Median-joining network analysis of haplotypes was performed using the programme Network 4.611 (http://www.fluxus-engineering.com/sharenet.htm). The SNP, haplotype frequencies of the two genes in Tibetan yak, Tibetan cattle and Holstein-Friesian were compared by using Pearson’s chi-square tests or Fisher’s exact tests with Bonferroni’s correction. The odds ratios (OR) with 95% confidence intervals were analyzed utilizing the Mito Tool (http://www.mitotool.org/index.html). The software SMART (http://smart.embl-herdelberg.de/) was used to identify protein domain structures and the location of mutations of MT-ND3 and MT-ND4L.
Results and discussion
Tibetan yak and Tibetan cattle were susceptible to the high-altitude environment and they had developed a series of novel metabolic reactions that help maintain ATP synthesis under limited oxygen availability over an evolutionary time frame. Previous studies have implicated that depletion of mitochondrial DNA in cells during hypoxia resulted in abnormal translation of erythropoietin, which influences the transport of oxygen and the efficiency of metabolism (Chandel et al. Citation1998). In this study, we compared the diversities of MT-ND3 and MT-ND4L between high-altitude cattle and low-altitude cattle to figure out the molecular mechanism of high-altitude adaptability. Considering that there might be some original sequence differences between yak and cattle, thus, we introduce Tibetan cattle to minimize this effect and to find more accurate changes derived from altitude that differed in Tibetan cattle or Holstein-Friesian. As shown in , there were no significant differences in the nucleotide composition among three breeds. Adenine account for the highest percentage (30.9% in MT-ND3 and 32.2% in MT-ND4L), and the base with the lowest percentage was guanine (12.3% in MT-ND3 and 11.7% in MT-ND4L). The T + A percentage account for more than half of all bases for both genes in yak and cattle populations, which was consistent with the content of vertebrate mitochondrial genomes (Luo et al. Citation2008). A total of 17 missense mutations in MT-ND3 and nine missense mutations in MT-ND4L were identified ( and ). No mutation was found in Tibetan yak and thus we focus on the differences between Tibetan cattle and Holstein-Friesian. In detail, three SNPs m.9893 A > G, m.9932 A > C, and m.10155 C > T in MT-ND3 gene showed a negative association with high-altitude adaptation (p < .003), while SNP m.10073C > T was positively associated with high-altitude adaptation (p < .0006). Specifically, m.9893 A > G was predicted to be located in the transmembrane region where act as aisle for electron transfer. Although no obvious difference between the TC (Tibetan cattle) and HF (Holstein-Friesian) groups according to and , the non-synonymous mutation m.10349 A > G located at the transmembrane region differed haplotype Ha4 from Ha5 ( and ), indicating a negative association between m.10349 A > G and high-altitude adaptability. All Tibetan yaks were included by two haplotypes (H1 of MT-ND3 and Ha1 of MT-ND4L). This indicated that these two mitochondrial genes are strongly conserved in Tibetan yak. Comparing with Holstein-Friesian, more Tibetan cattle were included in the control haplotype (H1 and Ha1). Besides, in view of haplotype analysis on MT-ND3 and MT-ND4L ( and ), haplotypes H1 and H5 in MT-ND3 and Ha1 in MT-ND4L showed positive associations with high-altitude adaptability, while haplotype H3 and Ha3 negatively associated with this adaptability. We further constructed median-joining network for MT-ND3 and MT-ND4L. According to , Tibetan yak was the largest group in haplotype H1, followed by Tibetan cattle. Only one variable site (m.10073 C > T, a non-synonymous mutation) that differed between haplotype H2 and H3, although they had different dominant cattle group. Haplotype H4 and H7 are separated by a missense mutation 9908 T > C, which was located in the predicted transmembrane region ( and ). Although Ha3 was almost equally divided into two groups of cattle, Ha3 differed from Ha2 only by the missense mutation (m.10392 C > T) (). These data revealed that polymorphisms of MT-ND3 and MT-ND4L may affect the proper function of related enzymes, which is necessary for high-altitude adaptation.
Figure 1. Median-joining network of MT-ND3 (A) and MT-ND4L (B) haplotypes. Black sector in H1 and Ha1 represents Tibetan cattle and Tibetan yak. In other haplotypes, black sector only represents Tibetan cattle. White sector represents Holstein-Friesian Cattle. The arrow indicates that the reference sequence belongs to haplotype H1 and Ha1.
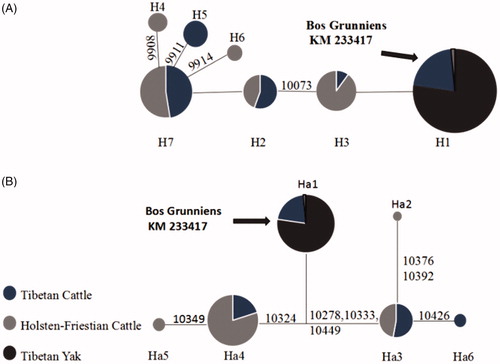
Figure 2. Diagram of the transmembrane structure of the MT-ND3 (A) and MT-ND4L (B) protein predicted using the SMART programme. The arrows indicate that the non-synonymous mutations were present in each of the transmembrane regions.
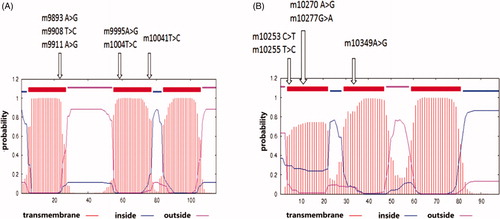
Table 1. Percentage of nucleotide composition of MT-ND3 and MT-ND4L gene sequences in Tibetan yaks (TY), Tibetan cattle (TC), and Holstein-Friesian (HF) populations.
Table 2. Mutations in MT-ND3 of Tibetan cattle (TC) and Holstein-Friesian (HF).
Table 3. Mutations in MT-ND4L of Tibetan cattle (TC) and Holstein-Friesian (HF).
Table 4. Haplotype distribution for MT- ND3 in Holstein-Friesian (HF) and Tibetan cattle (TC).
Table 5. Haplotype distribution for MT-ND4L in Holstein-Friesian (HF) cattle and Tibetan cattle (TC).
Disclosure statement
No potential conflict of interest was reported by the authors.
Additional information
Funding
References
- Adam MP, Ardinger HH, Pagon RA, Wallace SE, Ljh B, Stephens K, Amemiya A. 2003. Mitochondrial DNA-associated Leigh syndrome and NARP: PubMed.
- Chandel NS, Maltepe E, Goldwasser E, Mathieu CE, Simon MC, Schumacker PT. 1998. Mitochondrial reactive oxygen species trigger hypoxia-induced transcription. Proc Natl Acad Sci U S A. 95:11715–11720.
- Consortium UP. 2015. UniProt: a hub for protein information. Nucl Acids Res. 43:204–212.
- Coskun PE, Ruiz-Pesini E, Wallace DC. 2003. Control region mtDNA variants: longevity, climatic adaptation, and a forensic conundrum. Proc Natl Acad Sci U S A. 100:2174.
- Flaquer A, Baumbach C, Kriebel J, Meitinger T, Peters A, Waldenberger M, Grallert H, Strauch K. 2014. Mitochondrial genetic variants identified to be associated with BMI in adults. PLoS One. 9:e105116.
- Ghezzi D, Marelli C, Achilli A, Goldwurm S, Pezzoli G, Barone P, Pellecchia MT, Stanzione P, Brusa L, Bentivoglio AR, et al. 2005. Mitochondrial DNA haplogroup K is associated with a lower risk of Parkinson's disease in Italians. Eur J Hum Genet. 13:748.
- Gnaiger E. 2003. Oxygen conformance of cellular respiration. Hypoxia. Springer; p. 39–55.
- Harri R, Johanna A, Heli YO, Aino L, Juha P, Hassinen IE, Kari M. 2002. Cytoskeletal structure of myoblasts with the mitochondrial DNA 3243Asn Italiansisease in Italiansame> <fname > E.</fname> <sname > Ruiz-Pesini<. Cell Motil Cytoskeleton. 53:231–238.
- La MC, Caporali L, Gandini F, Olivieri A, Toni F, Nassetti S, Brunetto D, Stipa C, Scaduto C, Parmeggiani A. 2014. Association of the mtDNA m.4171C > A/MT-ND1 mutation with both optic neuropathy and bilateral brainstem lesions. BMC Neurol. 14:1–5.
- Leonard JV, Schapira A. 2000. Mitochondrial respiratory chain disorders II: neurodegenerative disorders and nuclear gene defects. Lancet. 355:389.
- Luo Y, Gao W, Gao Y, Tang S, Huang Q, Tan X, Chen J, Huang T. 2008. Mitochondrial genome analysis of Ochotona curzoniae and implication of cytochrome c oxidase in hypoxic adaptation. Mitochondrion. 8:352–357.
- Mishmar D, Ruiz-Pesini E, Golik P, Macaulay V, Clark AG, Hosseini S, Brandon M, Easley K, Chen E, Brown MD, et al. 2003. Natural selection shaped regional mtDNA variation in humans. Proc Natl Acad Sci USA. 100:171–176.
- Mukai M, Sugaya K, Ozawa T, Goto YI, Yagishita A, Matsubara S, Bokuda K, Miyakoshi A, Nakano I. 2015. Isolated mitochondrial stroke‐like episodes in an elderly patient with the MT‐ND3 gene mutation. Neurol Clin Neurosci. 3:153–156.
- Nakamaru-Ogiso E, Han H, Matsuno-Yagi A, Keinan E, Sinha SC, Yagi T, Ohnishi T. 2010. The ND2 subunit is labeled by a photoaffinity analogue of asimicin, a potent complex I inhibitor. FEBS Lett. 584:883–888.
- Nesbitt V, Morrison PJ, Crushell E, Donnelly DE, Alston CL, He L, McFarland R, Taylor RW. 2012. The clinical spectrum of the m.10191T > C mutation in complex I-deficient Leigh syndrome. Dev Med Child Neurol. 54:500–506.
- Newman JH, Holt TN, Hedges LK, Womack B, Memon SS, Willers ED, Wheeler L, Phillips JA, Hamid R. 2011. High-altitude pulmonary hypertension in cattle (brisket disease): candidate genes and gene expression profiling of peripheral blood mononuclear cells. Pulmonary Circulation. 1:462–469.
- Reed KL, Blaeser LL, Dantzer V, Green ML, Simmen RC. 1998. Control of secretory leukocyte protease inhibitor gene expression in the porcine periimplantation endometrium: a case of maternal-embryo communication. Biol Reprod. 58:448.
- Sarzi E, Brown MD, Lebon S, Chretien D, Munnich A, Rotig A, Procaccio V. 2007. A novel recurrent mitochondrial DNA mutation in ND3 gene is associated with isolated complex I deficiency causing Leigh syndrome and dystonia. Am J Med Genet A. 143A:33–41.
- Scott GR, Schulte PM, Egginton S, Scott ALM, Richards JG, Milsom WK. 2011. Molecular evolution of cytochrome c oxidase underlies high-altitude adaptation in the bar-headed goose. molecular. Biol Evol. 28:351.
- Shi Y, Hu Y, Wang J, Elzo MA, Yang X, Lai S. 2018. Genetic diversities of MT-ND1 and MT-ND2 genes are associated with high-altitude adaptation in yak. Mitochondrial DNA Part A. 29:485–494.
- Wang HN, Chen HD, Chen KY, Xiao JF, He K, Xiang GA, Xie X. 2014. Highly expressed MT-ND3 positively associated with histological severity of hepatic steatosis. APMIS. 122:443–451.
- Weir EK, Tucker A, Reeves JT, Will DH, Grover RF. 1974. The genetic factor influencing pulmonary hypertension in cattle at high altitude. Cardiovasc Res. 8:745–749.
- Yan-Iing Zhao, Jian-Zhou Wang, Yang-Zom Y-X. 2011. Isolation and culture of fetal fibroblasts of Tibetan yellow cattle. Animal Husbandry Feed Sci. 10:3–5.
- Yu WMP, Turnbull DM, Chinnery PF. 2005. Leber hereditary optic neuropathy. Berlin, Heidelberg: Springer.