Abstract
Tetraena mongolica, an endangered shrub, is endemic to China’s western Inner Mongolia. Here we have reported its complete chloroplast genome with a length of 106,288 bp. The inverted repeat regions just retained 4315 bp. The genome encodes a total of 98 predicted genes, including 64 protein-coding genes, 30 transfer RNA genes and 4 ribosomal RNA genes. Fifteen genes, including infA, rps16, ycf1, ycf2 and all the 11 ndh genes, were probably lost from the genome. The clpP and rps12 lost one intron, respectively. All these characters show T. mongolica is a special taxon worth to be further studied.
Tetraena mongolica Maxim (Zygophyllaceae), which is the only species of the genus Tetraena, is endemic to China’s western Inner Mongolia (Beier et al. Citation2003; Ge et al. Citation2011). The accumulation of triacylglycerol makes it easily burnable, thus being used as firewood by the local people (Wang et al. Citation2007). Due to its narrow growth range and human overuse, T. mongolica has been listed as a nationally endangered plant (Qin et al. Citation2017). In DNA level, plastid genome (plastome) can provide remarkable information for its phylogeny and conservation genetics. However, Tetraena, even Zygophyllaceae or Zygophyllales, were poorly studied in this aspect.
The fresh leaves of T. mongolica were collected from in vitro seedlings in the Institute of Botany, Chinese Academy of Sciences (IBCAS), Beijing. The DNA was sheared to 500 bp and prepared in the DNA library. Total 3.3 Gb 100-bp pair-end reads were generated on Illumina HiSeq 2500 (Illumina, San Diego, USA). The raw reads were filtered for low-quality bases (PHRED < 20) by NGSQC Toolkit v2.3.3 (Patel and Jain Citation2012). De novo assembly was conducted in VELVET v1.2.10 (Zerbino and Birney Citation2008). Plastome sequences were picked up by blasting all contigs against confamilial species Larrea tridentata (accession number: NC_028023) using BLAST v2.4.0 (Camacho et al. Citation2009) with default parameter, then were assembled manually in Geneious R8 (Biomatters, Inc., Auckland, New Zealand; http://www.geneious.com). Gene annotation was determined by DOGMA (Wyman et al. Citation2004), and adjusted manually in Geneious R8. The genome sequence has been submitted to NCBI (https://www.ncbi.nlm.nih.gov) under accession number MK265246.
The complete plastome of T. mongolica is 106,288 bp in length, containing a large single-copy region of 80,458 bp and a small single-copy (SSC) region of 17,200 bp. The overall GC content is 33.6%. A total of 98 predicted genes were identified, including 64 protein-coding genes, 30 transfer RNA genes, and 4 ribosomal RNA (rRNA) genes.
Fifteen protein-coding genes were lost from the plastome, including infA, rps16, ycf1, ycf2, and all the 11 ndh complex genes. Except infA, which still had a pseudo leftover, the other three genes (rps16, ycf1, and ycf2) were totally deleted from the genome. Compared to 19,350 bp in L. tridentata, the inverted repeat regions (IRs) of T. mongolica were highly contracted with only 4315 bp each. All the four rRNA genes, rrn4.5, rrn5, rrn16, and rrn23, were shifted to the SSC region. Gene and intron losses and IR boundary changes were observed as well in the desert plant saguaro cactus (Carnegiea gigantea) (Sanderson et al. Citation2015). Besides heterotrophism (Barrett et al. Citation2014; Feng et al. Citation2016; Wicke et al. Citation2013), drought and heat could be another reason for plastome degradation.
We also used the coding sequences of T. mongolica and another 15 plastomes to reconstruct a Maximum Likelihood tree through RAxML (Stamatakis Citation2014) in CIPRES website (Miller et al. Citation2010). Tetraena mongolica and L. tridentata formed the clade corresponding to Zygophyllaceae (). This topology confirmed that Zygophyllales is the basal taxa of fabids (Stevens Citation2018). The new plastome sequence would provide a reliable conservation genetic and evolutionary resource for this endangered plant.
Figure 1. Phylogenetic tree based on the coding sequences of 16 plastomes. Bootstrap support values from 1000 replicates are shown above the branches. All the plastome sequences are available in GenBank, with the accession numbers listed right next to their scientific names. The new plastome obtained in this study is shown in bold.
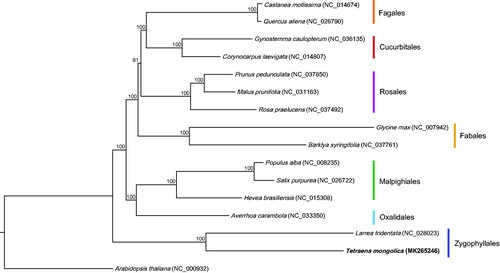
Disclosure statement
The authors report that they have no conflict of interest. The authors alone are responsible for the content and writing of the paper.
References
- Barrett CF, Freudenstein JV, Li J, Mayfield-Jones DR, Perez L, Pires JC, Santos C. 2014. Investigating the path of plastid genome degradation in an early-transitional clade of heterotrophic Orchids, and implications for heterotrophic angiosperms. Mol Biol Evol. 31:3095–3112.
- Beier BA, Chase MW, Thulin M. 2003. Phylogenetic relationships and taxonomy of subfamily Zygophylloideae (Zygophyllaceae) based on molecular and morphological data. Plant Syst Evol. 240:11–39.
- Camacho C, Coulouris G, Avagyan V, Ma N, Papadopoulos J, Bealer K, Madden TL. 2009. BLAST plus: architecture and applications. BMC Bioinformatics. 10:421.
- Feng YL, Wicke S, Li JW, Han Y, Lin CS, Li DZ, Zhou TT, Huang WC, Huang LQ, Jin XH. 2016. Lineage-specific reductions of plastid genomes in an Orchid tribe with partially and fully mycoheterotrophic species. Genome Biol Evol. 8:2164–2175.
- Ge XJ, Hwang CC, Liu ZH, Huang CC, Huang WH, Hung KH, Wang WK, Chiang TY. 2011. Conservation genetics and phylogeography of endangered and endemic shrub Tetraena mongolica (Zygophyllaceae) in Inner Mongolia, China. BMC Genet. 12:1.
- Miller MA, Pfeiffer W, Schwartz T. 2010. Creating the CIPRES Science Gateway for inference of large phylogenetic trees. In Proceedings of the Gateway Computing Environments Workshop (GCE), New Orleans, Louisiana, USA. 1–8.
- Patel RK, Jain M. 2012. NGS QC toolkit: a toolkit for quality control of next generation sequencing data. PLoS One. 7:e30619.
- Qin H, Zhao L, Yu S, Liu H, Liu B, Xia N, Peng H, Li Z, Zhang Z, He X. 2017. Evaluating the endangerment status of china’s angiosperms through the red list assessment. Biodiversity Sci. 25:745–757.
- Sanderson MJ, Copetti D, Búrquez A, Bustamante E, Charboneau JL, Eguiarte LE, Kumar S, Lee HO, Lee J, McMahon M, et al. 2015. Exceptional reduction of the plastid genome of saguaro cactus (Carnegiea gigantea): loss of the ndh gene suite and inverted repeat. Am J Bot. 102:1115–1127.
- Stamatakis A. 2014. RAxML version 8: a tool for phylogenetic analysis and post-analysis of large phylogenies. Bioinformatics. 30:1312–1313.
- Stevens PF. 2018. Angiosperm Phylogeny Website. Version 14. http://www.mobot.org/MOBOT/research/APweb/
- Wang G, Lin QQ, Xu Y. 2007. Tetraena mongolica Maxim can accumulate large amounts of triacylglycerol in phloem cells and xylem parenchyma of stems. Phytochemistry. 68:2112–2117.
- Wicke S, Muller KF, de Pamphilis CW, Quandt D, Wickett NJ, Zhang Y, Renner SS, Schneeweiss GM. 2013. Mechanisms of functional and physical genome reduction in photosynthetic and nonphotosynthetic parasitic plants of the broomrape Family. Plant Cell. 25:3711–3725.
- Wyman SK, Jansen RK, Boore JL. 2004. Automatic annotation of organellar genomes with DOGMA. Bioinformatics. 20:3252–3255.
- Zerbino DR, Birney E. 2008. Velvet: algorithms for de novo short read assembly using de Bruijn graphs. Genome Res. 18:821–829.