Abstract
Tullbergia bisetosa is a springtail (Collembola) native to the sub-Antarctic Prince Edward Islands. Unlike most other springtails, it has acquired a euedaphic (living within the soil) life form. In the present study, the complete mitogenome of T. bisetosa was sequenced. It has a length of 15,204 bp and comprises 13 protein-coding genes, 22 tRNAs, and two rRNAs. The mitogenome has the following base composition: A = 35.5%, T = 32.3%, G = 10.6%, and C = 21.1%. A Bayesian phylogenetic tree shows that T. bisetosa is grouped with springtails that possess similar morphological features and similar life histories. Our results suggest a mitogenomic signal towards morphological traits that conforms to a euedaphic lifestyle.
Collembola (springtails) are a ubiquitous and widespread group of arthropods that inhabit a wide range of terrestrial ecosystems, although the choice of habitat varies between different species (Hopkin Citation1997). Springtails are macroscopic and wingless, and most species that are epidaphic (living in surface vegetation) possess a furca (springing organ) which facilitates their dispersal (Hopkin Citation1997). However, some springtails deviate from the norm and have adapted to different ecological niches. A case in hand is Tullbergia bisetosa Börner 1902 which has acquired a euedaphic life form (living deep within the soil). This species is blind, has reduced appendages, lacks a furca, and is likely parthenogenic during stable environmental conditions, which changes to sexual reproduction when conditions become unfavourable (Chahartaghi et al. Citation2009; Chernova et al. Citation2010). Here, we present the complete mitogenome of T. bisetosa and investigate its phylogenetic placement among other springtails with different morphological features and habitat preferences.
Genomic DNA was extracted from 16 individuals collected from the Prince Edward Islands (46.767°S, 37.850°E) using the DNeasy Blood and Tissue Kit (Qiagen®, Germany). DNA from one of these specimens has been deposited at the Iziko Museum, Cape Town, voucher number SAM-ENW-C010313. Genomic libraries were prepared and sequenced using the Illumina HiSeq4000 sequencer (Illumina, USA). The software package NOVOPlasty v2.7.2 (Dierckxsens et al. Citation2017) was used to assemble the mitogenome. The program’s default settings were used, except for the insert size, which was changed to 350 bp corresponding to the genomic library preparation pipeline. The starting seed for the assembly was the cytochrome c oxidase subunit I gene of T. bisetosa (Myburgh et al. Citation2007; Accession No. DQ147448). The MITOS WebServer (Bernt et al. Citation2013) was used for the initial annotation, and sequence boundaries of the protein-coding genes (PCGs) and ribosomal RNAs were confirmed using Exonerate v2.2 (Slater and Birney Citation2005) and Infernal v1.1 (Nawrocki and Eddy Citation2013).
The PCGs of T. bisetosa and nine other closely related species (sequence identity >69%) were independently aligned and a consensus Bayesian phylogenetic tree of all 13 PCGs was constructed in BEAST v2.5 (Bouckaert et al. Citation2014) selecting the HKY substitution model (Hasegawa et al. Citation1985), 300 million MCMC iterations, and a 33% burnin. The phylogenetic tree was visualized in Figtree v1.4.3 (Rambaut Citation2016) ().
Figure 1. A Bayesian phylogenetic tree of Tullbergia bisetosa and nine other species (six Collembola and three outgroup taxa). The following species were used for the phylogenetic analysis: Tullbergia bisetosa MK520870, Tetrodontophora bielanensis AF272824, Friesea grisea EU124719, Gomphiocephalus hodgsoni NC_005438, Cryptopygus antarcticus NC_010533, Cryptopygus terranovus NC_037610, Orchesella villosa EU016195, Anopheles funestus MG742199, Sabethes belisarioi NC_037498, and Zeugodacus tau MF966383. The posterior probabilities are at each node.
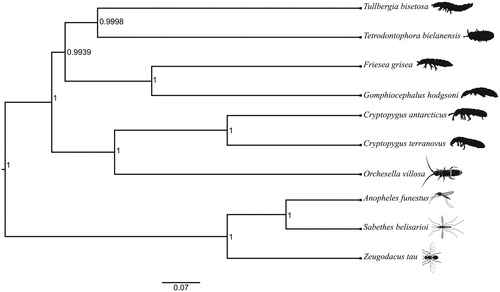
The circularized mitogenome consists of 15,204 bp with the base composition of A = 35.5%, T = 32.3%, G = 10.6%, and C = 21.1%. We observed several occurrences of non-canonical initiation and truncated stop codons (TNN), and a low GC content, both of which are common in invertebrates (Donath et al. Citationin press; Tikole and Sankararamakrishnan Citation2006).
The Bayesian phylogenetic tree recovered T. bisetosa and Tetradontophora bielanensis as sister taxa within a monophyletic clade that comprises other euedaphic springtails, Friesea grisea and Gomphiocephalus hodgsoni. The current phylogeny supports a single origin for the euedaphic lifestyle in Collembola. The epidaphic springtails (Cryptopygus antarcticus, Cryptopygus terranovus, and Orchesella villosa) form a separate clade (see also Monsanto et al. Citation2019). Since mitochondrial DNA is involved in thermal and anaerobic respiratory adaptations (Silva et al. Citation2014), the current study emphasises that mitogenomes may be under selection for traits that are critical for a euedaphic lifestyle.
Acknowledgements
Specimens were collected under Prince Edward research permits granted by the Department of Environmental Affairs (DEA) (permit number 1/2013). The DEA is acknowledged for logistical support. We acknowledge the Centre for High Performance Computing (CHPC) in Cape Town and the University of Johannesburg’s IT services for bioinformatics support.
Disclosure statement
The authors have reported no conflict of interest, and are responsible for the content and writing of this article.
Additional information
Funding
References
- Bernt M, Donath A, Jühling F, Externbrink F, Florentz C, Fritzsch G, Pütz J, Middendorf M, Stadler PF. 2013. MITOS: improved de novo metazoan mitochondrial genome annotation. Mol Phylogenet Evol. 69:313–319.
- Bouckaert R, Heled J, Kühnert D, Vaughan T, Wu C-H, Xie D, Suchard MA, Rambaut A, Drummond AJ. 2014. BEAST 2: a software platform for bayesian evolutionary analysis. PLOS Comput Biol. 10:e1003537.
- Chahartaghi M, Maraun M, Scheu S, Domes K. 2009. Resource depletion and colonization: a comparison between parthenogenetic and sexual Collembola species. Pedobiologia. 52:181–189.
- Chernova NM, Potapov MB, Savenkova YY, Bokova AI. 2010. Ecological significance of parthenogenesis in collembola. Entomol Rev. 90:23–38.
- Dierckxsens N, Mardulyn P, Smits G. 2017. NOVOPlasty: de novo assembly of organelle genomes from whole genome data. Nucleic Acids Res. 45:1–9.
- Donath A, Juhling F, Al-Arab M, Stadler PF, Middendorf M, Bernt M. In press. Improved annotation of protein-coding genes boundaries in metazoan mitochondrial genomes. Nucleic Acids Res. 1–10.
- Hasegawa M, Kishino H, Yano T. 1985. Dating of the human-ape splitting by a molecular clock of mitochondrial DNA. J Mol Evol. 22:160–174.
- Hopkin SP. 1997. Biology of the springtails (Insecta: Collembola). New York: Oxford University Press.
- Monsanto DM, Jansen van Vuuren B, Jagatap H, Jooste CM, Janion-Scheepers C, Teske PR, Emami-Khoyi A. 2019. The complete mitogenome of the springtail Cryptopygus antarcticus travei provides evidence for speciation in the Sub-Antarctic region. Mitochondrial DNA Part B. 4:1195–1197.
- Myburgh M, Chown SL, Daniels SR, Jansen van Vuuren B. 2007. Population structure, propagule pressure, and conservation biogeography in the sub-Antarctic: lessons from indigenous and invasive springtails: biodiversity research. Divers Distrib. 13:143–154.
- Nawrocki EP, Eddy SR. 2013. Infernal 1.1: 100-fold faster RNA homology searches. Bioinformatics. 29:2933–2935.
- Rambaut A. 2016. FigTree v1.4.3. [accessed 2019 February 1]. Available from: http://tree.bio.ed.ac.uk/software/figtree/
- Silva G, Lima FP, Martel P, Castilho R. 2014. Thermal adaptation and clinal mitochondrial DNA variation of European anchovy. Proc R Soc B. 281:20141093.
- Slater GS, Birney E. 2005. Automated generation of heuristics for biological sequence comparison. BMC Bioinform. 6:31–42.
- Tikole S, Sankararamakrishnan R. 2006. A survey of mRNA sequences with a non-AUG start codon in refseq database. J Biomol Struct Dyn. 24:33–41.