Abstract
The complete mitochondrial genome was sequenced from the calanoid copepod Eurytemora affinis. The sequenced total genome size was 18,553 bp. The mitochondrial genome of E. affinis has 13 protein-coding genes (PCGs), two rRNAs, and 22 tRNAs. Of 13 PCGs, ND1, ND5, and ATP6 genes had incomplete stop codons TA–, T—, and TA–, respectively. Furthermore, the stop codons of the remaining eleven PCGs were TAG or TAA while the start codon of 13 PCGs was ATG (Cytb, ATP8, ATP6, and CO3 genes), ATT (CO1, ND2, ND3, ND4L, ND5, and ND6 genes), and ATA (ND1, ND4, and CO2 genes), respectively. The ratio of A + T and G + C nucleotides of 13 PCGs of E. affinis mitogenome showed 63.9% and 36.1%, respectively while those ratio of the entire sequences were 65.5% and 34.5%, respectively.
To date, 12 species have been retrieved in the genus Eurytemora (http://v3.boldsystems.org/index.php/Taxbrowser_Taxonpage?taxid=5798); however, not a single complete mitochondrial genome has been reported from those species. Despite the limited mitochondrial genome information of these species, the population genetic analyses were examined to reveal how they invade freshwater ecosystem, using 16S rRNA and cytochrome oxidase 1 (CO1) gene (Lee, Citation1999, Citation2000, Citation2016; Winkler et al. Citation2008; Sukhikh et al. Citation2016). Also, environmental ecotoxicology using the RNA-seq (Legrand et al. Citation2016; Lee et al. Citation2018) of E. affinis have been widely reported (Lee et al. Citation2017; Legrand et al. Citation2017; Zidour et al. Citation2019), in addition to usefulness of E. affinis in studying the environmental adaptation (e.g. temperature) (Karlsson et al. Citation2018), but the taxonomical consideration of the copepod E. affinis based on complete mitogenome is still unavailable. Based on its wide distribution of the genus Eurytemora with ecological importance, complete mitochondrial genome will be useful for a better understanding on the phylogenetic divergence of E. affinis populations in the genus Eurytemora.
The calanoid copepod E. affinis was obtained from the laboratory cultures that originated from the sample copepods on September 2014 from the oligohaline zone of the Seine Estuary (49°45′34.38″N, 0°17′33.68″E) by Prof. Sami Souissi (Michalec et al. Citation2017) and maintained in Nagasaki University in Japan. The specimen was deposited in the copepod collection of the Fisheries Science Museum of Nagasaki University, Nagasaki University under the accession no. FFNU-Cr-00393.
We sequenced 300 bp paired-end library of E. affinis from the whole body genomic DNA using the Illumina HiSeq 2500 platform (GenomeAnalyzer, Illumina, San Diego, CA). De novo assembly was conducted using spades v3.13.0 (http://cab.spbu.ru/software/spades/) with K-mer auto. Of the assembled E. affinis 729,332 contigs (N50 = 2051 bp) with Newbler (version 2.9; identity 100) (http://www.454.com), one supercontig was obtained. After a manual curation of one supercontig with Consed (version 19.0) (http://www.phrap.org/consed/consed.html) with a gap closing, a single supercontig was mapped to the mitochondrial DNA of E. affinis. The total length of the complete mitochondrial genome of E. affinis was 18,553 bp (GenBank accession no. MN043905). The mitochondrial genome of E. affinis contained 13 protein-coding genes (PCGs), two rRNAs, and 22 tRNAs. The direction of 13 PCGs of E. affinis was mostly different to those of other copepods but the directions of the mitochondrial genome of the harpacticoid copepods Tigriopus japonicus and Tigriopus californicus were identical (). The ratio of A + T and G + C nucleotides of 13 PCGs of E. affinis mitogenome showed 63.9% and 36.1%, respectively, while those ratio of all the sequences were 65.5% and 34.5%, respectively.
Figure 1. Phylogenetic analysis. We conducted comparison of the mitochondrial genomes of nine copepods. Amino acids of 13 PCGs gene from nine copepods were aligned using MEGA software (ver. 10.0.1) with the ClustalW alignment algorithm. To establish the best-fit substitution model for phylogenetic analysis, the model with the lowest Bayesian Information Criterion (BIC) and Akaike Information Criterion (AIC) scores were estimated using a maximum-likelihood (ML) analysis. According to the results of model test, maximum likelihood phylogenetic analyses were performed with the LG + G + I model. Gray color represents the opposite direction of PCGs and the Black color represents the control regions; Upper and lower single letters indicate the direction of tRNA clockwise and anticlockwise, respectively. Modified from Hwang et al. (Citation2019).
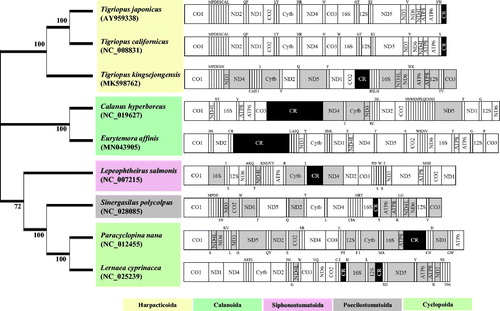
The phylogenetic placement of E. affinis was obtained using previously reported complete copepod mitogenomes (). The phylogenetic placement of the two calanoid copepods Calanus hyperboreus and E. affinis were in the same clade, but their orientations of 13 PGCs and tRNAs were different, indicating rearrangements of those particular components of mitochondrial DNA in the calanoid copepods over evolution. This information will be helpful for a better understanding of mitogenome evolution in the genus Eurytemora.
Disclosure statement
The authors report no conflicts of interest. The authors alone are responsible for the content and writing of the paper.
Additional information
Funding
References
- Hwang D-S, Choi B-S, Lee M-C, Han J, Kim S, Lee J-S. 2019. Complete mitochondrial genome of the Antarctic copepod Tigriopus kingsejongenesis (Harpacticoida, Harpacticidae). Mitochondrial DNA B. 4:1470–1471.
- Karlsson K, Puiac S, Winder M. 2018. Life-history responses to changing temperature and salinity of the Baltic Sea copepod Eurytemora affinis. Mar Biol. 165:30.
- Lee B-Y, Lee M-C, Jeong C-B, Kim H-J, Hagiwara A, Souissi S, Han J, Lee J-S. 2018. RNA-Seq-based transcriptome profiling and expression of 16 cytochrome P450 genes in the benzo[α]pyrene-exposed estuarine copepod Eurytemora affinis. Comp Biochem Physiol D Genomics Proteomics. 28:142–150.
- Lee CE, Remfert JL, Opgenorth T, Lee KM, Stanford E, Connolly JW, Kim J, Tomke S. 2017. Evolutionary responses to crude oil from the Deepwater Horizon oil spill by the copepod Eurytemora affinis. Evol Appl. 10:813–828.
- Lee CE. 1999. Rapid and repeated invasions of fresh water by the copepod Eurytemora affinis. Evolution. 53:1423–1434.
- Lee CE. 2000. Global phylogeography of a cryptic copepod species complex and reproductive isolation between genetically proximate “populations”. Evolution. 54:2014–2027.
- Lee CE. 2016. Evolutionary mechanisms of habitat invasions, using the copepod Eurytemora affinis as a model system. Evol Appl. 9:248–270.
- Legrand E, Boulangé-Lecomte C, Restoux G, Trémolet G, Duflot A, Forget-Leray J. 2017. Individual and mixture acute toxicity of model pesticides chlordecone and pyriproxyfen in the estuarine copepod Eurytemora affinis. Environ Sci Pollut Res Int. 24:5976–5984.
- Legrand E, Forget-Leray J, Duflot A, Olivier S, Thomé JP, Danger JM, Boulangé-Lecomte C. 2016. Transcriptome analysis of the copepod Eurytemora affinis upon exposure to endocrine disruptor pesticides: focus on reproduction and development. Aquat Toxicol. 176:64–75.
- Michalec F-G, Fouxon I, Souissi S, Holzner M. 2017. Zooplankton can actively adjust their motility to turbulent flow. Proc Natl Acad Sci USA. 114:E11199–E11207.
- Sukhikh N, Souissi A, Souissi S, Winkler G, Castric V, Holl AC, Alekseev V. 2016. Genetic and morphological heterogeneity among populations of Eurytemora affinis (Crustacea: Copepoda: Temoridae) in European waters. C R Biol. 339:197–206.
- Winkler G, Dodson JJ, Lee CE. 2008. Heterogeneity within the native range: population genetic analyses of sympatric invasive and noninvasive clades of the freshwater invading copepod Eurytemora affinis. Mol Ecol. 17:415–430.
- Zidour M, Boubechiche Z, Pan YJ, Bialais C, Cudennec B, Grard T, Drider D, Flahaut C, Ouddane B, Souissi S. 2019. Population response of the estuarine copepod Eurytemora affinis to its bioaccumulation of trace metals. Chemosphere. 220:505–513.