Abstract
The Ascaris roundworms (Ascaridida: Nematoda), one of the commonest soil-transmitted helminths (STHs), can cause ascariasis with significant socioeconomic and public health impact. In this study, the mitochondrial genome of Ascaris ovis, a representative of this genus from the sheep in Southwest China was determined using Illumina sequencing technology. The assembled genome was 14,205 bp in size and encoded 36 genes, including 12 protein-coding genes, 22 transfer RNA genes, and two ribosomal RNA genes. Phylogenetic analysis showed that A. ovis grouped with the congeneric Ascaris lumbricoides of humans, Ascaris spp. of non-human primates and Ascaris suum of pigs and together formed a monophyletic group relationship with either species of Baylisascaris/Toxascaris/Parascaris, species of Toxocara, species of Anisakis/Pseudoterranova or species of Ascaridia/Heterakis in the order Ascaridida, supporting its genetic similarity with A. lumbricoides, A. suum, and other congeneric species. The cumulative mitochondrial DNA data of this genus should contribute to a better understanding of the phylogenetic relationships among these roundworms.
The Ascaris roundworms (Ascaridida: Nematoda), one of the commonest soil-transmitted helminths (STHs), can cause the socioeconomically important disease ascariasis in various mammals (Dold and Holland Citation2011; Xie et al. Citation2013). However, owing to the sampling and survey biases, the current targeted species in this genus are mostly toward the roundworms that are of medical and veterinary importance, such as the human Ascaris lumbricoides and pig Ascaris suum (Dold and Holland Citation2011; Betson and Stothard Citation2016; Sadaow et al. Citation2018). Little or no attention is paid to roundworms infecting other hosts including domestic animals. Ascaris ovis Rudolphi, 1819, one common parasitic nematode of sheep and goats, represents a significant threat to both populations, especially the lambs and kids (Neveu-Lemaire Citation1923; Goodey Citation1926; Mozgovoi and Nosik Citation1951). The damage to the host body includes inflammation and scarring of the intestinal wall and parenchyma of the liver and lung (caused by larvae), as well as intestinal inflammation, obstruction, and even death (caused by adults) (Shcherbinin Citation1959; Niu et al. Citation2018). Although there has been substantial progress in studies on the morphology and biology of A. ovis so far, the knowledge gaps to understand this parasite at the molecular level, especially in genetics and molecular epidemiology, are still not sufficiently explored due to lacking suitable genetic markers (Niu et al. Citation2018). Moreover, given remarkable similarities in morphological characteristics and chromosome karyotypes between A. ovis and A. suum (Goodey Citation1926; Vassilev and Mutafova Citation1974), it is still unclear whether Ascaris species from sheep and goats represent A. suum or a different species. Under such context, we sequenced the mitochondrial genome of a representative A. ovis from the sheep using Illumina sequencing technology, because the mitochondrial DNA (mtDNA) has been not only proven be an important source for molecular markers but its complete data can also provide novel insights into the genome-wide comparative, phylogenetic-based analyses of Ascaris (Hu and Gasser Citation2006; Jex et al. Citation2010; Xie et al. Citation2013).
The parasite specimens (n = 2) were obtained from a naturally infected stray sheep housed in a mixed pastoral-farming area at Yajiang (30°03′N, 101°01′E), Sichuan Province of Southwest China, after treatment with pyrantel pamoate. After morphological identification, both worms were identified as the adult females of A. ovis according to the taxonomic key of Goodey (Citation1926). One specimen was used for DNA extraction and another was fixed in 5% formalin solution and archived in the Parasitological Museum of Sichuan Agricultural University (Ya'an, China) under collection numbers XY2018_19. Whole genomic DNA was extracted from a small portion (1.5 cm) of the worm specimen using the Universal Genomic DNA Extraction Kit Ver. 3.0 (TaKaRa, Dalian, China). After DNA yield and integrity assessment, a 300-bp paired-end (PE) genomic library was constructed according to manufacturer’s instructions (Illumina, San Diego, CA). The sequencing was carried out on HiSeq platform (BGI, Shenzhen, China) and reads were exported to the FASTQ format. The complete mtDNA was assembled using MITObim (Hahn et al. Citation2013) and annotated by MITOS (Bernt et al. Citation2013). The complete genome sequence was deposited in GenBank under accession number: MT993838.
The complete mitochondrial genome of A. ovis was 14,205 bp in size with 72.0% AT and encoded 12 protein-coding genes, 22 tRNA genes, and two rRNA genes. All genes were present on the same strand and unidirectionally transcribed, typical for other roundworms characterized so far (Park et al. Citation2011; Xie et al. Citation2011, Citation2013, Citation2019; Liu et al. Citation2012). Nine of the 12 protein-coding genes were predicted to use the TAA or TAG (atp6, cytb, cox2-3, nad1, nad4, and nad6) as the stop codons, while the remaining genes (cox1, nad2, and nad5) were deduced to end with an incomplete codon ‘T’ or ‘TA’. Twenty-two tRNA genes ranged in size from 51 bp (tRNA(AGN)-Ser and tRNA-Thr) to 61 bp (tRNA-Lys, tRNA-Ile, and tRNA-Met) and had distinctly different stem-loop structures when compared to those of other metazoan mtDNAs (Park et al. Citation2011; Liu et al. Citation2012; Xie et al. Citation2013; Xie et al. Citation2019). Two rRNAs, the small rRNA (rrnS; 692 bp) and large (rrnL; 960 bp) subunits, were located between tRNA-Glu and tRNA (UCN)-Ser and between tRNA-His and nad3, respectively. Two non-coding regions, namely NC1 (also known as AT-rich region; 868 bp) and NC2 (93 bp), were present between tRNA (UCN)-Ser and tRNA-Asn and between nad4 and cox1, respectively.
Based on a concatenated amino acid dataset of 12 protein-coding genes from 32 nematode parasites, a maximum-likelihood (ML) tree was reconstructed using Cucullanus robustus as the outgroup. As shown in , this tree topology clearly placed A. ovis together with the congeneric A. lumbricoides of humans, Ascaris spp. of non-human primates and A. suum of pigs and together formed a monophyletic group relationship with either species of Baylisascaris/Toxascaris/Parascaris in the family Ascarididae, species of Toxocara in the family Toxocaridae, species of Anisakis/Pseudoterranova in the family Anisakidae or species of Ascaridia/Heterakis in the family Ascaridiidae under the order Ascaridida, with high bootstrap values, supporting its genetic similarity with A. lumbricoides, A. suum, and other congeneric species (Niu et al. Citation2018). Further, a closer relationship was observed between A. ovis and A. lumbricoides than between A. ovis and A. suum, suggesting that A. ovis may share more genetic similarity to A. lumbricoides. Taken together, the sequenced mitochondrial genome of A. ovis in this study provides novel molecular evidence for its phylogenetic and taxonomic position in the genus Ascaris.
Figure 1. Maximum-likelihood tree inferred from concatenated amino-acid sequences of 12 mt protein-coding genes of T. leonine and other related nematodes, utilizing MtArt + I model and after 100,000 bootstrap replications (<50% support not shown). The solid black diamond represents the species in this study.
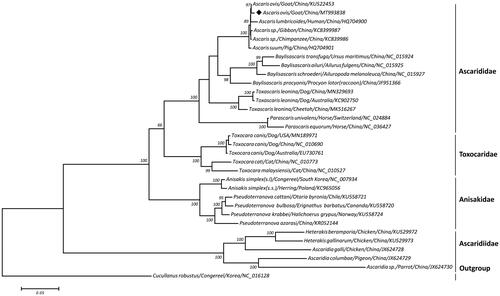
Disclosure statement
No potential conflict of interest was reported by the authors.
Data availability statement
The data that support the findings of this study are openly available in GenBank at https://www.ncbi.nlm.nih.gov/nuccore/MT993838. Associated BioProject, SRA, and BioSample accession numbers are PRJNA673292 (https://www.ncbi.nlm.nih.gov/bioproject/PRJNA673292), SRR12950074 (https://www.ncbi.nlm.nih.gov/sra/SRR12950074/), and SAMN16598390 (https://www.ncbi.nlm.nih.gov/biosample/SAMN16598390/), respectively.
Additional information
Funding
References
- Bernt M, Donath A, Jühling F, Externbrink F, Florentz C, Fritzsch G, Pütz J, Middendorf M, Stadler PF. 2013. MITOS: improved de novo metazoan mitochondrial genome annotation. Mol Phylogenet Evol. 69(2):313–319.
- Betson M, Stothard JR. 2016. Ascaris lumbricoides or Ascaris suum: what's in a name? J Infect Dis. 213(8):1355–1356.
- Dold C, Holland CV. 2011. Ascaris and ascariasis. Microbes Infect. 13(7):632–637.
- Goodey T. 1926. On the Ascaris from sheep. J Helminthol. 4(1):1–6.
- Hahn C, Bachmann L, Chevreux B. 2013. Reconstructing mitochondrial genomes directly from genomic next-generation sequencing reads—a baiting and iterative mapping approach. Nucleic Acids Res. 41(13):e129.
- Hu M, Gasser RB. 2006. Mitochondrial genomes of parasitic nematodes-progress and perspectives. Trends Parasitol. 22(2):78–84.
- Jex AR, Littlewood DT, Gasser RB. 2010. Toward next-generation sequencing of mitochondrial genomes—focus on parasitic worms of animals and biotechnological implications. Biotechnol Adv. 28(1):151–159.
- Liu GH, Wu CY, Song HQ, Wei SJ, Xu MJ, Lin RQ, Zhao GH, Huang SY, Zhu XQ. 2012. Comparative analyses of the complete mitochondrial genomes of Ascaris lumbricoides and Ascaris suum from humans and pigs. Gene. 492(1):110–116.
- Mozgovoi AA, Nosik AF. 1951. On the validity of Ascaris ovis, the Ascaris of small ruminants. Trudy Gelmint Lab. 5:23–27.
- Neveu-Lemaire M. 1923. Présence d’Ascaris ovis chez le chevreuil (Capreolus capreolus). Ann Parasitol Hum Comp. 1(3):265–268.
- Niu MR, Wang HB, Liu TL, Fan XC, Song JK, Zhao GH. 2018. Phylogenetic analysis of Ascaris ovis based on sequences of the mitochondrial genome. Chin J Vet Sci. 38(1):154–159.
- Park YC, Kim W, Park JK. 2011. The complete mitochondrial genome of human parasitic roundworm, Ascaris lumbricoides. Mitochondrial DNA. 22(4):91–93.
- Sadaow L, Sanpool O, Phosuk I, Rodpai R, Thanchomnang T, Wijit A, Anamnart W, Laymanivong S, Aung WPP, Janwan P, et al. 2018. Molecular identification of Ascaris lumbricoides and Ascaris suum recovered from humans and pigs in Thailand, Lao PDR, and Myanmar. Parasitol Res. 117(8):2427–2436.
- Shcherbinin IV. 1959. A rare case of ascariasis in a sheep. Zool Zh. 38(12):1888.
- Vassilev I, Mutafova T. 1974. Comparative studies on the karyotype of Ascaris suum and “Ascaris ovis”. Z Parasitenkd. 43(2):115–121.
- Xie Y, Li H, Wang C, Li Y, Liu Y, Meng X, Wang L, Zhou X, Zheng Y, Zuo Z, et al. 2019. Characterization of the complete mitochondrial genome sequence of the dog roundworm Toxascaris leonina (Nematoda, Ascarididae) from China. Mitochondrial DNA Part B. 4(2):3517–3519.
- Xie Y, Niu L, Zhao B, Wang Q, Nong X, Chen L, Zhou X, Gu X, Wang S, Peng X, et al. 2013. Complete mitochondrial genomes of chimpanzee- and gibbon-derived Ascaris isolated from a zoological garden in Southwest China. PLOS One. 8(12):e82795.
- Xie Y, Zhang Z, Wang C, Lan J, Li Y, Chen Z, Fu Y, Nie H, Yan N, Gu X, et al. 2011. Complete mitochondrial genomes of Baylisascaris schroederi, Baylisascaris ailuri and Baylisascaris transfuga from giant panda, red panda and polar bear. Gene. 482(1–2):59–67.