Abstract
Toxascaris leonina is a polyxenical parasite and commonly found in canids and felids. In this study, we used the Illumina high throughput sequencing and assembly to determine the complete mitogenome of a representative of this parasite from the Siberian tiger (Panthera tigris altaica). The genome was 14,248 bp in size and encoded 12 protein-coding genes, 22 transfer RNAs, and two ribosomal RNAs. Phylogeny showed that two canid (dog)-originated T. leonina were phylogenetically distinct from two felid-originated T. leonina (tiger isolate and cheetah isolate), suggesting at least two distinct subclades of T. leonina infecting these hosts and supporting once again that T. leonina represents a species complex. Furthermore, four isolates of T. leonina grouped together and were more closely related to other species from the family Ascarididae than species of families Toxocaridae, Anisakidae and Ascaridiidae, demonstrating phylogenetic stability of these paraphyletic groups characterized in this study. These cumulative mitochondrial DNA data provide a better understanding of phylogenetic relationships of this polyxenical and zoonotic roundworm species.
The roundworm Toxascaris leonina is a polyxenical parasite and its host range includes the domestic dogs and cats as well as wild canids and felids, such as wolves, foxes, tigers, and lions (Okulewicz et al. Citation2012; Fogt-Wyrwas et al. Citation2019; Jin et al. Citation2019). Unlike other roundworms, T. leonina lifecycle is simple and infections often follow by oral ingestion of the infective eggs (Sprent Citation1959). Then, the egg-hatched larvae penetrate the mucosal lining of the small intestine and settle down there for development. After several molts, these larvae return to the intestinal lumen where they mature and mate, and the females release eggs into the feces. The clinical symptoms contain diarrhea, emesis, abdominal discomfort, and even life-threatening blockage of intestinal obstruction (Lee et al. Citation2010). Humans can also be infected through interaction with dogs or cats, or ingestion of eggs in contaminated food. Until now there have been several clinic cases of human infections with T. leonina characterized and often involved to aberrant larva migrans in the eye (ocular larva migrans, OLM) and the viscera (visceral larva migrans, VLM) (Robertson and Thompson Citation2002; Okulewicz et al. Citation2012). Moreover, increased epidemiological evidences suggest that T. leonina is emerging as an underestimated zoonotic agent because of close relationships between humans and their pets (e.g. dogs and cats) and increased interactions between people and wildlife hosts in zoos (Robertson and Thompson Citation2002; Li et al. Citation2007; Li et al. Citation2008; Okulewicz et al. Citation2012; Xie et al. Citation2020). Such situations highlight the significance and necessity for diagnosis and identification of T. leonina. Unfortunately, current diagnosis and identification of this parasite still rely on morphological characteristics, host sources and geographical distributions although these criteria are not always sufficient to distinguish the morphologically similar species (Gasser Citation2006; Chen et al. Citation2012; Fogt-Wyrwas et al. Citation2019; Xie et al. Citation2020). Under such context, obtaining a more efficient approach to identify T. leonina infections has become urgent for clinical diagnosis and epidemiological investigation, and achieving this goal is foreseeable only through utilization of molecular methodologies. Numerous studies have demonstrated that the mitogenomic DNA is an informative marker and has been widely used for species-specific identification in many zoonotic nematodes (Hu and Gasser Citation2006; Hu et al. Citation2004). Herein, we reported the complete mitogenome of a representative T. leonina isolated from its wildlife host, the Siberian tiger.
In November 2018, four roundworm samples were collected from a naturally infected Siberian tiger (Panthera tigris altaica) provided by the Veterinary Hospital, Chengdu Zoo (30°42′N, 104°06′E), Sichuan of China, after treatment with pyrantel pamoate. Following morphological identification (Sprent Citation1959) and molecular sequencing (Zhu et al. Citation1999), these parasite specimens were determined as T. leonina adults with one male and three females. One female was subsequently used for extraction of genomic DNA and the remaining were formalin-fixed and archived in the Parasitological Museum of Sichuan Agricultural University (https://dop.sicau.edu.cn/; [email protected] (Yue Xie)) under collection numbers XY2018_23-25. Total genomic DNA was extracted using the Universal Genomic DNA Extraction Kit (TaKaRa, Dalian, China). After quality and quantity assessment, ∼5 µg genomic DNA was sheared into c. 350 bp fragments to construct a paired-end (PE) library, followed by sequencing on the Illumina HiSeq X Ten platform (BerryGenomics, Beijing, China). The clean reads (∼3.5 Gb) were assembled with MITObim (Hahn et al. Citation2013) using the mitogenome of cheetah T. leonina as the reference. The completeness and accuracy of this assembly was validated by multiple whole-genome alignment at the nucleotide level using species of Ascaris and Baylisascaris for which complete mitogenome sequences are available. Annotation was performed using MITOS (Bernt et al. Citation2013) with manual adjustment on the basis of whole genome-guided gene alignments and online BLAST against Nt and Nr databases. The complete genome sequence was deposited in GenBank under accession number: MW560284.
The mitogenome of the tiger T. leonina was 14,248 bp in size and encoded 12 protein-coding genes (PCGs), 22 tRNAs, and two rRNAs. Similar to congeneric species including those from the dog and cheetah (Liu et al. Citation2014; Jin et al. Citation2019; Xie et al. Citation2019), all of these genes were located on the same strand and transcribed in one direction. Twelve PCGs, except nad2 and nad5 genes deduced to use an incomplete stop codon ‘T’, were predicted to use the typical TAG as the stop codons. Twenty-two tRNAs ranged from 52 bp (tRNA(AGN)-Ser) to 62 bp (tRNA-Lys) in size, the same as those of the dog T. leonina (Xie et al. Citation2019). Two rRNAs, the small rRNA (rrnS; 700 bp) and large (rrnL; 959 bp) subunits, presented between tRNA-Glu and tRNA (UCN)-Ser and between tRNA-His and nad3, respectively. In addition, two non-coding regions, namely NC1 (also known as AT-rich region; 1000 bp) and NC2 (100 bp), were located between tRNA (UCN)-Ser and tRNA-Asn and between nad4 and cox1, respectively, in accordance with other roundworm species, suggesting their conservation and function in regulation of transcription and DNA replication (Clayton Citation1991).
On the basis of the available complete mitogenomes of Ascaridida species retrieved from GenBank, an alignment of 3,445 amino acid loci was yielded from the concatenated 12 PCGs of 33 nematode parasites using MAFFT v7.271 (Katoh Citation2002) and GBLOCKS v0.91b (Castresana Citation2000) for phylogeny. A maximum-likelihood (ML) tree was subsequently constructed with PHYML 3.0 (Guindon and Gascuel Citation2003) using Cucullanus robustus as the outgroup and edited for display using FigTree v1.4.3 (http://tree.bio.ed.ac.uk/software/figtree/). As shown in , this phylogenic tree clearly showed that two dog-originated T. leonina (Chinese isolate and Australian isolate) were phylogenetically distinct from two felid-originated T. leonina (tiger isolate and cheetah isolate), consistent with recent molecular studies (Jin et al. Citation2019; Fogt-Wyrwas et al. Citation2019; Xie et al. Citation2020), suggesting at least two distinct subclades of T. leonina infecting these hosts and supporting that T. leonina represents a species complex. Furthermore, four T. leonina isolates of grouped together, regardless of isolate origins and hosts, and were more closely related to other species from the family Ascarididae than species of families Toxocaridae, Anisakidae, and Ascaridiidae, demonstrating phylogenetic stability of these paraphyletic groups characterized in this study. Taken together, these cumulative mitochondrial DNA data provide a better understanding of classification and phylogenetic relationships of this polyxenical and zoonotic roundworm species.
Figure 1. Phylogenetic relationships among roundworm nematodes. The maximum likelihood phylogenetic tree was inferred from concatenated amino-acid sequences of 12 mitochondrial protein-coding genes of T. leonina and other related nematodes, utilizing MtArt + I model selected by TREEFINDER under the corrected Akaike's Information Criterion and 1,000,000 bootstrap replications (<50% support not shown). The solid black diamond represents the species in this study.
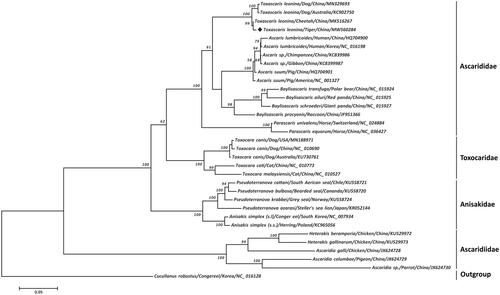
Disclosure statement
No potential conflict of interest was reported by the authors.
Data availability statement
The data that support the findings of this study are openly available in GenBank of NCBI at https://www.ncbi.nlm.nih.gov, under the accession number MW560284. Associated BioProject, SRA, and BioSample numbers are PRJNA673292, SRR12950074, and SAMN16598390, respectively.
Additional information
Funding
References
- Bernt M, Donath A, Jühling F, Externbrink F, Florentz C, Fritzsch G, Pütz J, Middendorf M, Stadler PF. 2013. MITOS: improved de novo metazoan mitochondrial genome annotation. Mol Phylogenet Evol. 69(2):313–319.
- Castresana J. 2000. Selection of conserved blocks from multiple alignments for their use in phylogenetic analysis. Mol Biol Evol. 17(4):540–552.
- Chen J, Zhou DH, Nisbet AJ, Xu MJ, Huang SY, Li MW, Wang CR, Zhu XQ. 2012. Advances in molecular identification, taxonomy, genetic variation and diagnosis of Toxocara spp. Infect Genet Evol. 12(7):1344–1348.
- Clayton DA. 1991. Replication and transcription of vertebrate mitochondrial DNA. Annu Rev Cell Biol. 7:453–478.
- Fogt-Wyrwas R, Dabert M, Jarosz W, Rząd I, Pilarczyk B, Mizgajska-Wiktor H. 2019. Molecular data reveal cryptic speciation and host specificity in Toxascaris leonina (Nematoda: Ascarididae). Vet Parasitol. 266:80–83.
- Gasser RB. 2006. Molecular tools-advances, opportunities and prospects. Vet Parasitol. 136(2):69–89.
- Guindon S, Gascuel O. 2003. A simple, fast, and accurate algorithm to estimate large phylogenies by maximum likelihood. Syst Biol. 52(5):696–704.
- Hahn C, Bachmann L, Chevreux B. 2013. Reconstructing mitochondrial genomes directly from genomic next-generation sequencing reads-a baiting and iterative mapping approach. Nucleic Acids Res. 41(13):e129–e129.
- Hu M, Chilton NB, Gasser RB. 2004. The mitochondrial genomics of parasitic nematodes of socio-economic importance: recent progress, and implications for population genetics and systematics. Adv Parasitol. 56:134–213.
- Hu M, Gasser RB. 2006. Mitochondrial genomes of parasitic nematodes-progress and perspectives. Trends Parasitol. 22(2):78–84.
- Jin YC, Li XY, Liu JH, Zhu XQ, Liu GH. 2019. Comparative analysis of mitochondrial DNA datasets indicates that Toxascaris leonina represents a species complex. Parasit Vectors. 12:194.
- Katoh K. 2002. MAFFT: a novel method for rapid multiple sequence alignment based on fast Fourier transform. Nucleic Acids Research. 30(14):3059–3066.
- Lee AC, Schantz PM, Kazacos KR, Montgomery SP, Bowman DD. 2010. Epidemiologic and zoonotic aspects of ascarid infections in dogs and cats. Trends Parasitol. 26(4):155–161.
- Li MW, Lin RQ, Chen HH, Sani RA, Song HQ, Zhu XQ. 2007. PCR tools for the verification of the specific identity of ascaridoid nematodes from dogs and cats. Mol Cell Probes. 21(5–6):349–354.
- Li MW, Lin RQ, Song HQ, Sani RA, Wu XY, Zhu XQ. 2008. Electrophoretic analysis of sequence variability in three mitochondrial DNA regions for ascaridoid parasites of human and animal health significance. Electrophoresis. 29(13):2912–2917.
- Liu GH, Zhou DH, Zhao L, Xiong RC, Liang JY, Zhu XQ. 2014. The complete mitochondrial genome of Toxascaris leonina: comparison with other closely related species and phylogenetic implications. Infect Genet Evol. 21:329–333.
- Okulewicz A, Perec-Matysiak A, Buńkowska K, Hildebrand J. 2012. Toxocara canis, Toxocara cati and Toxascaris leonina in wild and domestic carnivores. Helminthologia. 49(1):3–10.
- Robertson ID, Thompson RC. 2002. Enteric parasitic zoonoses of domesticated dogs and cats. Microbes Infect. 4(8):867–873.
- Sprent JFA. 1959. The life history and development of Toxascaris leonina (von Linstow 1902) in the dog and cat. Parasitology. 49:330–371.
- Xie Y, Li H, Wang C, Li Y, Liu Y, Meng X, Wang L, Zhou X, Zheng Y, Zuo Z, et al. 2019. Characterization of the complete mitochondrial genome sequence of the dog roundworm Toxascaris leonina (Nematoda, Ascarididae) from China. Mitochondrial DNA Part B. 4(2):3517–3519.
- Xie Y, Li Y, Gu X, Liu Y, Zhou X, Wang L, He R, Peng X, Yang G. 2020. Molecular characterization of ascaridoid parasites from captive wild carnivores in China using ribosomal and mitochondrial sequences. Parasit Vectors. 13:382.
- Zhu X, Chilton NB, Jacobs DE, Boes J, Gasser RB. 1999. Characterisation of Ascaris from human and pig hosts by nuclear ribosomal DNA sequences. Int J Parasitol. 29(3):469–478.