Abstract
We report the complete plastid genome of Cumathamnion serrulatum, also known as Delesseria serrulata. The plastid genome was 174,192 bp in size. Annotation showed there were 193 protein coding genes, three ribosomal RNAs, and 29 transfer RNAs. One intron was found, and the GC content was 27.2%. The maximum likelihood tree with the concatenated 177 plastid coding genes showed a strong monophyletic relationship to Membranoptera spp. within the Ceramiales.
Keywords:
Cumathamnion serrulatum (Harvey) M.J. Wynne & G.W. Saunders 2012, previously known as Delesseria serrulata, is a red algal species of the Ceramiales, Florideophyceae, Rhodophyta (Wynne and Saunders Citation2012). Cumathamnion serrulatum is widely distributed in Korea (Choi et al. Citation2002), Japan (Yoshida et al. Citation1990), Russia (Kozhenkova Citation2009), and North America (Wynne and Saunders Citation2012). The thallus is bright rosy-red in color and its primary frond produces branches irregularly from the midrib. Numerous secondary fronds emerge with similar shapes, followed by third and fourth leaflets in a similar way. All leaflets are linear-lanceolate, but they vary in degree of attenuation (Wynne and Saunders Citation2012). Despite its ecological and pharmacological importance (Güven et al. Citation2010; Kim et al. Citation2012), no genomic study has been reported yet. Here, we report the complete plastid genome of C. serrulatum.
Cumathamnion serrulatum was collected in Gonghyeonjin, Gangwon-do, Korea (38°21'29.1"N 128°30'37.7"E) on 28 March 2015. A voucher specimen was deposited at Sungkyunkwan University Herbarium (https://www.skku.edu, HSY, [email protected]) under accession number SKKU003380. Before DNA extraction from a leaflet of thallus, epiphytic contaminants were removed with brushes, forceps and sterile water. Genomic DNA was extracted using LaboPass™ Tissue Genomic DNA Isolation Kit Mini (COSMO Genetech, Seoul, Korea) following the manufacturer’s instructions. Total DNA was cleaned up again to remove impurities using DNeasy PowerClean Cleanup Kit (QIAGEN, Hilden, Germany). Sequencing libraries were prepared using an Ion Xpress Plus gDNA Fragment Library Preparation kit for the 400 bp libraries (Thermo Fisher Scientific, San Francisco, California, USA). Sequencing reads were generated by an Ion Personal Genome Machine (PGM) platform using the Ion PGM Hi-Q Template kit and the Ion PGM Hi-Q sequencing kit (Thermo Fisher Scientific, San Francisco, California, USA).
Raw reads were trimmed and assembled with CLC Genomics Workbench 5.5.1 (CLC Bio, Aarhus, Denmark) and MIRA3 Assembler (Chevreux et al. Citation1999). Plastid contigs were sorted out using tBLASTn. A consensus sequence was confirmed with a read-mapping tool in CLC Genomics Workbench to correct sequencing errors and fill gaps. Protein coding genes were manually annotated with the aid of NCBI’s BLASTx function. Then, these genes were confirmed with published red algal plastid genomes (see ). The rRNA sequences were identified using the RNAmmer 1.2 Server with the option of bacteria (Lagesen et al. Citation2007) and re-confirmed using BLASTn on NCBI. The tRNAs were checked using the ARAGORN web server (Laslett and Canback Citation2004). Lastly, ambiguous regions were re-sequenced using PCR amplification followed by Sanger sequencing of amplicons. The newly assembled plastid genome of C. serrulatum (GenBank accession number: MW292565) was 174,192 bp in size. The plastid genome contained 193 protein coding genes (CDSs), three rRNAs, 29 tRNAs, and one intron. GC content was 27.2%.
Figure 1. Maximum likelihood (ML) tree with 177 concatenated plastid proteins from 16 species of Ceramiales and three species of Gelidales (Outgroup).
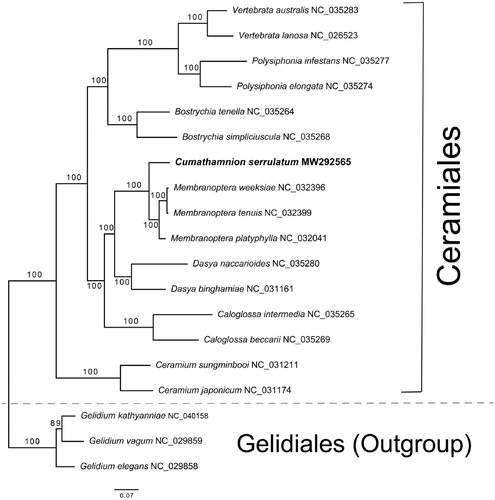
To construct a phylogeny with the concatenated plastid CDSs and to infer the phylogenetic position of C. serrulatum, 15 available plastid genomes of the Ceramiales were selected with an outgroup of three Gelidales species (Salomaki et al. Citation2015; Lee, Cho, et al. Citation2016a; Lee, Kim et al. Citation2016b; Hughey and Boo Citation2016; Tamayo and Hughey Citation2016; Díaz-Tapia et al. Citation2017; Hughey et al. Citation2017; Boo and Hughey Citation2019). The extraction of CDSs from plastid genomes was conducted following a method described in Lee et al. (Lee, Cho, et al. Citation2016a; Lee, Kim, et al. Citation2016b). A total of 177 gene-sets were aligned by MAFFT v7.310 (Katoh and Standley Citation2013). A maximum likelihood (ML) tree was constructed by IQ-tree v1.6.12 with 1000 ultrafast bootstrap replicates (Nguyen et al. Citation2015). The phylogeny showed a strong monophyletic relationship of C. serrulatum to three Membranoptera species, followed by Dasya and Calogrossa species (). Within the monophyly of 16 Ceramiales species, all internal nodes were fully resolved. Although its limited taxon sampling in this study, new plastid genome data of C. serrulatum may provide valuable information to resolve the phylogenetic relationships within the Ceramiales, the most species-rich order (2712 spp. www.algaebase.com) in Florideophyceae.
Acknowledgements
We thank to Robert A. Andersen for the valuable comments.
Disclosure statement
No potential conflict of interest was reported by the author(s).
Data availability statement
The plastid genome and raw data of C. serrulatum is available in the NCBI GenBank under accession number of MW292565 (https://www.ncbi.nlm.nih.gov/nuccore/MW292565) and the NCBI Sequence Read Archive (SRA) under the accession number of SRX9855090 with the BioProject accession number PRJNA692315 (https://www.ncbi.nlm.nih.gov/bioproject/PRJNA692315).
Additional information
Funding
References
- Boo GH, Hughey JR. 2019. Phylogenomics and multigene phylogenies decipher two new cryptic marine algae from California, Gelidium gabrielsonii and G. kathyanniae (Gelidiales, Rhodophyta). J Phycol. 55(1):160–172.
- Chevreux B, Wetter T, Suhai S. 1999. Genome sequence assembly using trace signals and additional sequence information. Proc German Conf Bioinformatics. 99:45–56.
- Choi HG, Kraft G, Lee IK, Saunders G. 2002. Phylogenetic analyses of anatomical and nuclear SSU rDNA sequence data indicate that the Dasyaceae and Delesseriaceae (Ceramiales, Rhodophyta) are polyphyletic. Eur J Phycol. 37(4):551–569.
- Díaz-Tapia P, Maggs CA, West JA, Verbruggen H. 2017. Analysis of chloroplast genomes and a supermatrix inform reclassification of the Rhodomelaceae (Rhodophyta). J Phycol. 53(5):920–937.
- Güven KC, Percot A, Sezik E. 2010. Alkaloids in marine algae. Mar Drugs. 8(2):269–284.
- Hughey JR, Boo GH. 2016. Genomic and phylogenetic analysis of Ceramium cimbricum (Ceramiales, Rhodophyta) from the Atlantic and Pacific Oceans supports the naming of a new invasive Pacific entity Ceramium sungminbooi sp. nov. Bot Mar. 59(4):211–222.
- Hughey JR, Hommersand MH, Gabrielson PW, Miller KA, Fuller T. 2017. Analysis of the complete plastomes of three species of Membranoptera (Ceramiales, Rhodophyta) from Pacific North America. J Phycol. 53:32–43.
- Katoh K, Standley DM. 2013. MAFFT multiple sequence alignment software version 7: improvements in performance and usability. Mol Biol E. 30(4):772–780.
- Kim YD, Park MS, Yoo HI, Min BH, Jin HJ. 2012. Seasonal variations of seaweed community structure at the subtidal zone of Bihwa on the East Coast of Korea. Kor J Fish Aquat Sci. 45(3):262–270.
- Kozhenkova SI. 2009. Retrospective analysis of the marine flora of Vostok Bay, Sea of Japan. Russ J Mar Biol. 35(4):263–278.
- Lagesen K, Hallin P, Rødland EA, Staerfeldt HH, Rognes T, Ussery DW. 2007. RNAmmer: consistent and rapid annotation of ribosomal RNA genes. Nucleic Acids Res. 35(9):3100–3108.
- Laslett D, Canback B. 2004. ARAGORN, a program to detect tRNA genes and tmRNA genes in nucleotide sequences. Nucleic Acids Res. 32(1):11–16.
- Lee JM, Cho CH, Park SI, Choi JW, Song HS, West JA, Bhattacharya D, Yoon HS. 2016. Parallel evolution of highly conserved plastid genome architecture in red seaweeds and seed plants. BMC Biol. 14(1):1–16.
- Lee JM, Kim KM, Yang EC, Miller KA, Boo SM, Bhattacharya D, Yoon HS. 2016b. Reconstructing the complex evolutionary history of mobile plasmids in red algal genomes. Sci Rep. 6:23744.
- Nguyen LT, Schmidt HA, von Haeseler A, Minh BQ. 2015. IQ-TREE: a fast and effective stochastic algorithm for estimating maximum-likelihood phylogenies. Mol Biol E. 32(1):268–274.
- Salomaki ED, Nickles KR, Lane CE. 2015. The ghost plastid of Choreocolax polysiphoniae. J. Phycol. 51:217–221.
- Tamayo DA, Hughey JR. 2016. Organellar genome analysis of the marine red alga Dasya binghamiae (Dasyaceae, Rhodophyta) reveals an uncharacteristic florideophyte mitogenome structure. Mitochondrial DNA Part B. 1(1):510–511.
- Wynne MJ, Saunders GW. 2012. Taxonomic assessment of North American species of the genera Cumathamnion, Delesseria, Membranoptera and Pantoneura (Delesseriaceae, Rhodophyta) using molecular data. Algae. 27(3):155–173.
- Yoshida T, Nakajima YT, Nakata Y. 1990. Check-list of marine algae of Japan (Revised in 1990). Jpn J Phycol. 38:269–320.