Abstract
Pleurosigma inscriptura M. A. Harper Citation2009 is a marine diatom in Naviculales (Bacillariophyceae) order distributed in New Zealand, South America, Argentina, and Korea. We assembled the complete mitochondrial genome sequence of Pleurosigma inscriptura (38,013 bp), and annotated 34 protein-coding genes, 25 transfer RNAs, and 2 ribosomal RNAs. We analyzed a maximum-likelihood tree using conserved 34 mitochondrial genes from Bacillariophyta species. In the mitochondrial phylogeny, P. inscriptura showed a strong monophyletic relationship with Haslea nusantara and Navicula ramosissima.
Pleurosigma inscriptura M. A. Harper Citation2009, belongs to order Naviculales (Bacillariophyceae), is a kind of marine pennate diatom which is one of the important primary producers (Nelson et al. Citation1995; Harper et al. Citation2009) . Pleurosigma lives in a benthic environment, and includes epiphytic species with gliding movement over the surface area (Happey-Wood and Jones Citation1988; Sterrenburg Citation1991; Grossi et al. Citation2004; Al-Handal and Wulff Citation2008; Poulin et al. Citation2014). Pleurosigma was characterized by sigmoid raphe having a convex valve, and striated areola (Harper et al. Citation2009; Sar et al. Citation2012; Al-Handal et al. Citation2014; Poulin et al.Citation2014). The valve outline of P. inscriptura is lanceolate with a gently sigmoid raphe, and their raphe angle is smaller than other Pleurosigma species (Hasle and Syvertsen Citation1996; Harper et al. Citation2009). Pleurosigma inscriptura is distributed in New Zealand, South America, Argentina, and Korea (Sar et al. Citation2013; Harper et al. Citation2012; Lee and Park Citation2015). Since P. inscriptura was reported as a novel species (Harper et al. Citation2009), there is no report about molecular data in public databases (e.g. NCBI). In this study, we analyzed the mitochondrial genome of P. inscriptura (MW566731) isolated from Gijang, Busan, Korea (35°13′05.9″N, 129°13′47.3″E). The culture strain was deposited at Marine Ecological Genomics Lab. in Kyungpook National University (isolate MEG005; [email protected]).
To establish monoclonal strain of the target species, single cell isolation was performed by customized Pasteur pipettes (glass) under light microscope, and cultured under L1 medium (L1 media kit, https://ncma.bigelow.org/MKL150L). The isolate was identified by light microscopic (LM; Nikon ECLIPSE Ts2, Nikon, Tokyo, Japan) and scanning electron microscopic (SEM; Hitachi SU8220; Hitachi Ltd., Tokyo, Japan) observation based on previously described morphological characteristics (Harper et al. Citation2009; Lee and Park Citation2015). The morphological characteristics of P. inscriptura MEG005 are as follows: length of apical axis 104–115 μm (avg. 111 μm, n = 100), width of transapical axis 15–25 μm (avg. 21 μm, n = 100; Supplementary Figure S1(A)), lanceolate valves, 3.6° raphe angle (Supplementary Figure S1(B)), a small oval central nodule with no hyaline areas (Supplementary Figure S1(C)), 20 striae in 10 μm and small triangle of internal polar raphe ends ().
Figure 1. Maximum-likelihood (ML) tree using 34 concatenated mitochondrial proteins from eighteen Bacillariophyta and two Ochrophyta (outgroup) species.
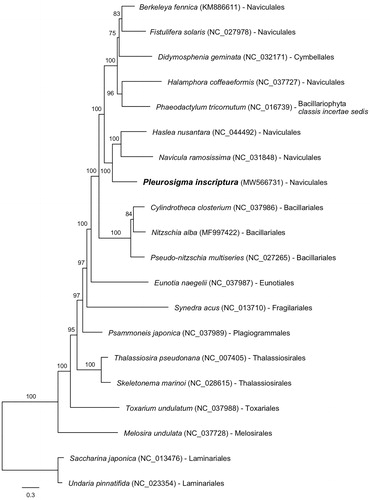
We have done DNA extraction using the DNeasy Plant Mini-DNA kit (Qiagen, Hilden, Germany). Genome sequencing of P. inscriptura (NCBI BioProject PRJNA717901; SRR14087012, 12.5 Gbp) and their de novo genome assembly were conducted by Illumina Novaseq6000 (Illumina, San Diego, CA; 150 bp paired-end library), and SPAdes assembler (v3.14.2; Bankevich et al. Citation2012), respectively. Mitochondrial genome contigs of P. inscriptura were sorted by local BLASTn search (e-value cutoff = 1.e − 05) with the mitochondrial genome of Berkeleya fennica (KM886611) as reference, and re-assembled as a circular mitochondrial genome from the sorted contigs. The circular form of mitochondrial genome (MW566731) was validated using the read-mapping method (bowtie2 v2.4.2; Langmead and Salzberg Citation2012).
CLC Main Workbench v20.0 (CLC Bio, Aarhus, Denmark) was used to annotate mitochondrial genes that manually predicted by BLASTx search (NCBI nr database; e-value cutoff = 1.e − 05) with codon table 4 (The Mold, Protozoan, and Coelenterate Mitochondrial Code). Ribosomal RNAs (rRNAs) were predicted by the RNAmmer 1.2 Server (Lagesen et al. Citation2007). Transfer RNAs (tRNAs) were analyzed by tRNA scan-SE 2.0 (Lowe and Chan Citation2016; Chan and Lowe Citation2019) and ARAGORN programs (Laslett and Canback Citation2004), respectively. A total 34 protein-coding genes, 25 transfer RNAs, 2 rRNAs is annotated in the mitochondrial genome of P. inscriptura (MW566731). Intron sequences of protein coding genes are present in rps3 (two introns), nad2 and nad11 (one intron).
To construct the concatenated alignment of mitochondrial genes, conserved 34 protein-coding genes from 18 Bacillariophyta and two outgroup species were used, and each homologous gene set was aligned by MAFFT (v7.313; Katoh and Toh Citation2008) with default options. The maximum-likelihood (ML) tree using the concatenated alignment was constructed by IQ-tree program (v1.6.12; Nguyen et al. Citation2015) with the options as follows: the gene partition information (-q), the model test (-m TEST), and ultrafast bootstrapping with 1000 replications (-bb 1000). Pleurosigma inscriptura is monophyly with Haslea nusantara and Navicula ramosissima (bootstrap support 100%; ), and this clade is clustered with the clade of Berkeleya fennica, Fistulifera solaris, Didymosphenia geminata, Halamphora coffeaeformis, and Phaeodactylum tricornutum (bootstrap support 100%). In the clade, almost all species belong to Naviculales order but D. geminate and P. tricornutum are Cymbellales and Bacillariophyta classis incertae sedis, respectively (Guiry and Guiry Citation2021). In previous studies, D. geminate and P. tricornutum show monophyletic relationship with Naviculales order that the phylogenetic tree was constructed by 27 mitochondrial protein-coding genes (Aunins et al. Citation2018). Therefore, we postulate that D. geminate and P. tricornutum could be regarded as the Naviculales order. More mitochondrial genome samples in Naviculales order will provide a better understanding of phylogenetic relationship between genera.
tmdn_a_1945970_sm7512.jpg
Download JPEG Image (252.1 KB)Acknowledgments
The authors thank Hyun Shik Cho (Marine Ecological Genomics Lab., Department of Oceanography, Kyungpook National University, Daegu) for technical support.
Disclosure statement
No potential conflict of interest was reported by the author(s).
Data availability statement
The data that support the findings of this study are openly available in GenBank at https://www.ncbi.nlm.nih.gov/genbank/, reference number ‘MW566731’. Raw reads of genome sequencing data in this study were uploaded to the NCBI Sequence Read Archive (SRA) database (BioProject PRJNA717901; SRR14087012).
Additional information
Funding
References
- Al-Handal AY, Wulff A. 2008. Marine benthic diatoms from Potter Cove, King George Island, Antarctica. Bot Marina. 51(1):51–68.
- Al-Handal AY, Dawood SA, Angela W, Mujtaba TA. 2014. Epiphytic diatoms of the Mesopotamian wetland, Huwaiza marsh, South Iraq. Diatom. 30:164–178.
- Aunins AW, Hamilton D, King TL. 2018. The complete mitochondrial genome of the stalk-forming diatom Didymosphenia Geminata. Mitochondrial DNA Part B. 3(2):676–677.
- Bankevich A, Nurk S, Antipov D, Gurevich AA, Dvorkin M, Kulikov AS, Lesin VM, Nikolenko SI, Pham S, Prjibelski AD, et al. 2012. SPAdes: a new genome assembly algorithm and its applications to single-cell sequencing. J Comput Biol. 19(5):455–477.
- Chan PP, Lowe TM. 2019. tRNAscan-SE: searching for tRNA genes in genomic sequences. In: Kollmar M, editor. Gene prediction. Methods in molecular biology. New York: Humana; p. 1–14.
- Grossi V, Beker B, Geenevasen JA, Schouten S, Raphel D, Fontaine MF, Damsté JSS. 2004. C(25) highly branched isoprenoid alkenes from the marine benthic diatom Pleurosigma strigosum. Phytochemistry. 65(22):3049–3055.
- Guiry MD, Guiry GM. 2021. AlgaeBase. Galway: National University of Ireland. https://www.algaebase.org.
- Happey-Wood CM, Jones P. 1988. Rhythms of vertical migration and motility in intertidal benthic diatoms with particular reference to Pleurosigma angulatum. Diatom Res. 3(1):83–93.
- Harper MA, Cassie Cooper V, Chang FH, Nelson WA, Broady PA. 2012. Phylum Ochrophyta: brown and golden-brown algae, diatoms, silicioflagellates, and kin. In: Gordon DP, editor. New Zealand inventory of biodiversity. Vol. 3. Kingdoms bacteria, protozoa, chromista, plantae, fungi canterbury. Christchurch: University Press; p. 114–163.
- Harper MA, Patterson JE, Harper JF. 2009. New diatom taxa from the world’s first marine Bioblitz held in New Zealand: Skeletomastus a new genus, Skeletomastus coelatus nov. comb. and Pleurosigma inscriptura a new species. Acta Bot Croat. 68(2):339–349.
- Hasle GR, Syvertsen EE. 1996. Marine diatoms. In: Tomas CR, editor. Identifying marine diatoms and dinoflagellates. Academic Press. San Diego; p. 5–385.
- Katoh K, Toh H. 2008. Recent developments in the MAFFT multiple sequence alignment program. Brief Bioinform. 9(4):286–298.
- Lagesen K, Hallin P, Rødland EA, Staerfeldt HH, Rognes T, Ussery DW. 2007. RNAmmer: consistent and rapid annotation of ribosomal RNA genes. Nucleic Acids Res. 35(9):3100–3108.
- Laslett D, Canback B. 2004. ARAGORN, a program to detect tRNA genes and tmRNA genes in nucleotide sequences. Nucleic Acids Res. 32(1):11–16.
- Langmead B, Salzberg SL. 2012. Fast gapped-read alignment with Bowtie 2. Nat Methods. 9(4):357–359.
- Lee JH, Park JS. 2015. Newly recorded diatom species in marine and fresh water of Korea. J Ecol Environ. 38(4):545–562.
- Lowe TM, Chan PP. 2016. tRNAscan-SE On-line: integrating search and context for analysis of transfer RNA genes. Nucleic Acids Res. 44(W1):W54–W57.
- Nelson DM, Tréguer P, Brzezinski MA, Leynaert A, Quéguiner B. 1995. Production and dissolution of biogenic silica in the ocean: revised global estimates, comparison with regional data and relationship to biogenic sedimentation. Global Biogeochem Cycles. 9(3):359–372.
- Nguyen LT, Schmidt HA, von Haeseler A, Minh BQ. 2015. IQ-TREE: a fast and effective stochastic algorithm for estimating maximum-likelihood phylogenies. Mol Biol Evol. 32(1):268–274.
- Poulin M, Underwood GJ, Michel C. 2014. Sub-ice colonial Melosira arctica in Arctic first-year ice. Diatom Res. 29(2):213–221.
- Sar EA, Hinz F, Sterrenburg FAS, Lavigne AS, Lofeudo S, Sunesen I. 2012. Species of Pleurosigma (Pleurosigmataceae) with lanceolate or slightly sigmoid valve outlines: analysis of type material. Diatom Res. 27(4):237–253.
- Sar EA, Sterrenburg FA, Lavigne AS, Sunesen I. 2013. Diatomeas de ambientes marinos costeros de Argentina: Especies del género Pleurosigma (Pleurosigmataceae). Bol Soc Argent Bot. 48(1):17–51.
- Sterrenburg FAS. 1991. Studies on the genera Gyrosigma and Pleurosigma (Bacillario-phyceae) light microscopical criteria for taxonomy. Diatom Res. 6(2):367–389.