Abstract
Phalaenopsis zhejiangensis is a rare and endangered plant species with extremely small populations. The complete chloroplast (cp) genome of P. zhejiangensis was assembled, its structural organization was described and comparative genomic analyses was carried out. The cp genome of P. zhejiangensis is 143,547 bp in length, with a GC content of 37.2%, which includes a pair of inverted repeats (IRs) of 24,464 bp separated by a small single-copy region of 10,764 bp and a large single-copy region of 83,856 bp. The cp genome contains 126 genes, consisting of 80 protein-coding genes, 38 transfer RNAs, and eight ribosomal RNAs. Six protein-coding genes, including ψndhB (two copies), ψndhD, ψndhG, ψndhK, and ψndhI, are identified as pseudogenes. Another six ndh genes, ndhA, ndhC, ndhE, ndhF, ndhH, and ndhJ, are missing from the plastid genome. A total of 41 cp simple sequence repeats (SSRs) were identified, including 40 mono-nucleotides and one di-nucleotides. Phylogenic analysis revealed P. zhejiangensis was nested inside the Phalaenopsis species and sister to P. wilsonii. The assembly and analysis of P. zhejiangensis cp genome will provide essential data for further study of taxonomy and systematics of Orchidaceae.
Introduction
Orchidaceae, a family of monocotyledonous plants, is one of the most successful and highly evolved monocots, comprising 763 genera with approximately 28,000 species (Hadi et al. Citation2015; Christenhusz and Byng Citation2016). The genus Phalaenopsis belongs to tribe Vandeae, which is commonly known as moth orchids, including more than 70 species widely distributed across Southeast Asia to northern Australia, with the majority in Indonesia and the Philippines (Ko et al. Citation2017; Gogoi and Rinya Citation2020; Lee et al. Citation2020). There are 12 Phalaenopsis species distributed in China, among which four species are endemics (Wu et al. Citation2009). Phalaenopsis zhejiangensis is a small epiphytic plant with slightly flattened roots, very short stems, basal leaves, and thinly textured flowers (Wu et al. Citation2009). P. zhejiangensis was transferred from Doritis zhejiangensis, while the latter was renamed from Nothodoritis zhejiangensis (Yukawa and Kita Citation2005), which was first found on a trunk of Podocarpus macrophyllus in 1970 at Xitianmu Mountain in Linan County, Zhejiang Province (Tsi Citation1989; Schuiteman Citation2012). P. zhejiangensis is a rare and endangered plant species in China, which is narrowly distributed in provinces of Zhejiang Province, Gansu, Anhui, and Shanxi, growing on the bark of trees or rock surfaces, with extremely small populations. As a result of habitat loss, P. zhejiangensis is listed as threatened with extinction. Fortunately, Zeng et al (Citation2011) have established an effective propagation protocol for large-scale propagation of this endangered orchid species. In recent years, several chloroplast (cp) genomes of Phalaenopsis plants, including P. aphrodite, Phalaenopsis ‘Tiny Star’, P. lowii, P. lobbii, and P. mannii, were sequenced and assembled (Chang et al. Citation2006; Kim et al. Citation2016; Wang et al. Citation2019; Chen et al. Citation2020; Zhang et al. Citation2020). However, the cp genome of P. zhejiangensis has not yet been assembled. In our study, the complete cp genome sequence of P. zhejiangensis was assembled to provide new insights into taxonomy and systematics of Orchidaceae.
Materials and methods
Plant material and DNA extraction
Fresh leaves were collected from a plant nursery (28°65.762′N, 121°46.976′E) in Jiaojiang, Zhejiang Province, China. A voucher specimen was deposited at the Molecular Biology Laboratory in Taizhou University (http://www.tzc.edu.cn, Dr. Huijuan Zhang, [email protected]) under a collection number of CHS2020029. Fresh leaves were ground into a fine powder in liquid nitrogen with a mortar and pestle, and genomic DNA was extracted following the CTAB-based protocol as described by Doyle and Doyle (Citation1987).
DNA sequencing and sequence assembly
Construction of a genomic DNA library was carried out according to the manufacturer’s instructions, and high-throughput sequencing was then performed using the Illumina Hiseq X Ten system (Illumina, San Diego, CA). Approximately, 3.64 Gb raw data of 150 bp paired-end Illumina reads were generated, and 3.63 Gb high-quality clean reads were harvested by using the NGS QC Toolkit v2.3.3 (Patel and Jain Citation2012). Geneious Prime 2019 (Biomatters, Auckland, New Zealand) was employed to assemble the complete cp genome.
Chloroplast genome annotation
Annotation of the complete cp genome was performed with Geneious Prime 2019 by using P. lobbii as a reference genome (NCBI accession number: MT830847) (Wang et al. Citation2019), and the borders of each gene were manually inspected and corrected where necessary. The circular cp genome map of P. zhejiangensis was drawn using the online program OrganellarGenomeDRAW (OGDRAW, http://ogdraw.mpimp-golm.mpg.de) with default settings (Lohse et al. Citation2013).
Simple sequence repeat analysis
Perl scripts of MIcroSAtellite Identification Tool (MISA) were used to identify and locate the potential simple sequence repeats (SSRs) loci in complete cp genome sequence of P. zhejiangensis (Thiel et al. Citation2003). To determine the presence of SSRs, 1–6 bp nucleotide motifs were considered, and the minimum repeat numbers were set as 10 repeat units for mono-nucleotides, six for di-nucleotides, and five for tri-, tetra-, penta-, and hexa-nucleotides.
Phylogenetic analysis
The complete cp genome sequences of 39 Orchidaceae species downloaded from NCBI were used for phylogenetic analysis, which included eight Phalaenopsis plants, six Holcoglossum species, and five Cleisomeria plants. The complete cp genome of Coix lacryma-jobi (FJ261955) was applied as an outgroup. A total of 41 genome sequences were aligned with a multiple sequence alignment program MAFFT v7.388 plugin in Geneious Prime 2019 under default settings (Katoh and Standley Citation2013). The best-fit evolutionary model of DNA substitution for maximum-likelihood (ML) analysis was determined by using jModelTest 2.1.9 under the Akaike information criterion (AIC) (Darriba et al. Citation2012). A phylogenetic tree was generated by PhyML 3.1, with 1000 bootstrap replicates (Guindon et al. Citation2010).
Results
Genome sequencing and assembly
More than 12 million paired-end clean reads were obtained, and a circular cp genome was assembled with Geneious Prime. The complete cp plastome of P. zhejiangensis is 143,547 bp in length which includes a pair of inverted repeats (IRs) of 24,464 bp separated by a large single-copy (LSC) region of 83,855 bp and a small single-copy (SSC) region of 10,764 bp (). The overall guanine–cytosine (GC) content of P. zhejiangensis cp genome is 36.8%, while the corresponding values of LSC, SSC, and IR sequences are 34.0%, 28%, and 43.5%, respectively.
Genome features of Phalaenopsis zhejiangensis
The complete cp plastome of P. zhejiangensis is comprised of 126 genes, including 74 protein-coding genes, 38 tRNA genes, eight rRNA genes, and six pseudogenes. All the six pseudogenes are ndhs, including ψndhD, ψndhG, ψndhI, ψndhK, and two copies of ψndhB. Additionally, other six ndh genes have been lost in the plastome, these are ndhA, ndhC, ndhE, ndhF, ndhH, and ndhJ. Six tRNA genes (trnA-UGC, trnH-GUG, trnI-CAU, trnI-GAU, trnL-CAA, trnN-GUU, trnR-ACG, and trnV-GAC) and four rRNA genes (rrn4.5, rrn5, rrn16, and rrn23) are present in two copies. Among the protein-coding genes, eight genes including rpl16, rps16, rpoC1, petB, petD, atpF, and two copies of rpl2 contain a single intron, while three genes, rps12, ycf3, and clpP, contain two introns. Moreover, six tRNA genes, trnA-UGC, trnG-GCC, trnI-GAU, trnK-UUU, trnL-UAA, and trnV-UAC, harbored a single intron have been identified ().
Table 1. List of genes in the chloroplast genome of Phalaenopsis zhejiangensis.
IR expansion and contraction
The IR region of P. zhejiangensis is 24,464 bp in length, while the other eight Phalaenopsis IRs included in our study are longer than P. zhejiangensis, they range from 24,719 bp to 25,846 bp (). Comparisons of LSC, IRB, SSC, and IRA junction boundaries among nine Phalaenopsis cp genomes were performed and presented in . In Phalaenopsis plastome, LSC/IRB boundary junctions lie within rpl22 gene except P. mannii, which locates in the intergenic region between rpl22 and rps19. Eight rpl12 genes extend 31 bp into IRB regions, while the rpl12 in P. wilsonii extends only one base pair into its IRB region (). The IRB/SSC junctions of P. mannii, P. equestris, and P. lobbi locate within the ψycf1 pseudogenes; however, no ψycf1 is identified in other six Phalaenopsis cp genomes including P. zhejiangensis. The SSC/IRA junctions of P. wilsonii, P. mannii, P. equestris, and P. lobbii lie within ycf1 genes, which extend 0 bp, 132 bp, 9 bp, and 108 bp into IRA regions, respectively while the SSC/IRA junctions of P. zhejiangensis, P. lowii, P. japonica, P. aphrodite subsp. formosana, and the hybrid ‘Tiny Star’ locate between ycf1 and trnN-GUU.
Figure 2. Structural variations at inverted-repeat and single-copy borders in nine Phalaenopsis chloroplast genomes. The figure features are not to scale regarding sequence length.
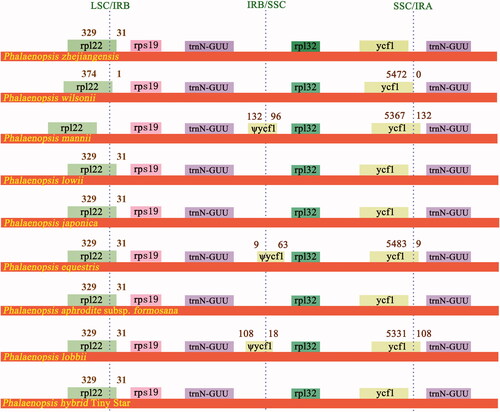
Table 2. Details of nine Phalaenopsis chloroplast genomes.
SSR analysis
Totally, P. zhejiangensis cp genome contains 41 SSRs (). Among the SSRs identified herein, A/T mononucleotide repeats account for the largest proportion of 97.56%. Only one di-nucleotide repeat was observed, and no tri-, tetra-, penta-, or hexa-nucleotides presented in the plastome. Most SSRs are distributed in LSC (70.73%), followed by SSC (24.39%), and the proportion in IRs is less than 5%. Among 41 SSRs, 25 loci lie in intergenic regions, four in introns, and 12 in coding regions or pseudogenes.
Table 3. Repeat sequences and their distribution within Phalaenopsis zhejiangensis chloroplast genome.
Phylogenetic analysis
Statistical selection of the best-fit model of nucleotide substitution was carried out by the jModelTest program, and GTR + G+I was turned out to be the best-fitted. To evaluate the phylogenetic relationship of P. zhejiangensis within Orchidaceae, a phylogenetic tree using the complete plastome sequences of 41 plant species were generated (). The resulting ML tree had very high bootstrap support for the majority of clades, and the phylogenetic tree was shown to be consistent with the traditional morphology-based taxonomy of Orchidaceae. Nine plants from the genus Phalaenopsis formed a well-supported monophyletic clade with 100% bootstrap value, and P. zhejiangensis was sister to P. wilsonii, with a support value of 97%.
Discussion
The classification of Orchidaceae is a very complex problem for its bewildering diversity and morphological parallelism, and also for botanical neglect (Dressler Citation1993). Orchidaceae were divided into five subfamilies, including Apostasioideae, Vanilloideae, Cypripedioideae, Orchidoideae and Epidendroideae, and Apostasioideae are monophyletic and sister to other subfamilies (Dixon et al. Citation2003; Tsai et al. Citation2013). The subfamily Orchidoideae is comprised of a large group of orchids, including four tribes (Diurideae, Cranichideae, Codonorchideae, and Orchideae) with around 3600 plant species that largely share terrestrial habits (Bateman et al. Citation2003; Serna-Sánchez et al. Citation2021). The genus Phalaenopsis belongs to tribe Orchideae, which consists of about 62 genera (Inda et al. Citation2012). The morphology of P. zhejiangensis is similar to both Doritis and P. lowii, with the characteristic feature of pollinia similar to that of Doritis, and its shapes of petals and columns similar to P. lowii (Tsi Citation1999; Christenson Citation2001). P. zhejiangensis was initially named N. zhejiangensis based on morphology, and then renamed as D. zhejiangensis (Yukawa and Kita Citation2005; Schuiteman Citation2012). Molecular analyses using ITS, trnL intron as well as trnL-F spacer prove that N. zhejiangensis is species nested in genus Phalaenopsis (Deng et al. Citation2015). Our molecular phylogenetic analyses confirm its systematic position, which indicates that P. zhejiangensis is sister to P. wilsonii, with a high support value.
The sizes of previously published Phalaenopsis cp genomes ranged from 144,607 bp (P. lobbii) to 148,964 bp (P. phalaenopsis aphrodite subsp. formosana) (Chang et al. Citation2006; Kim et al. Citation2016; Wang et al. Citation2019; Chen et al. Citation2020; Zhang et al. Citation2020). In our present study, the complete plastid genome of P. zhejiangensis is only 143,574 bp in length, which turns out to be the smallest among all known cp genome sequences in genus Phalaenopsis. The cp genomes of most flowering plants range from 120 kb to 160 kb, and the difference in genome size is mainly caused by contraction and expansion of the IR region (Goulding et al. Citation1996; Ingvarsson et al. Citation2003; Yao et al. Citation2020). The IR sizes of published Phalaenopsis plastid genomes are 24,719 (P. lobbii) (Wang et al. Citation2019) to 25,846 bp (P. equestris) (Jheng et al. Citation2012). In our present study, the length of P. zhejiangensis IR sequence is 24,464 bp, which is smaller than that of P. lobbii.
Plastid gene loss and pseudogenization are common phenomena in parasitic, semi-parasitic, and saprophytic plants (Molina et al. Citation2014; Li and Zheng Citation2018; Nie et al. Citation2019). In semi-parasitic plants of Macrosolen cochinchinensis, M. tricolor, and M. bibracteolatus, the infA and all the ndh genes were lost among the three species, and two genes, ycf1 and rpl2, were found to be pseudogenes (Nie et al. Citation2019). Gastrodia elata is a saprophytic plant with extremely small cp genome in which many genes related to photosynthesis are missing, and it contains only 28 genes, including 20 protein-coding genes, three rRNAs, and five tRNAs (Park et al. Citation2020). However, cp gene loss and pseudogenization are rarer in photosynthetic species, because the plastid gene cannot simply be discarded (Magee et al. Citation2010; Wicke et al. Citation2011; Daniell et al. Citation2016). Interestingly, pseudogenization, truncation, and deletion of ndh genes in cp genomes are common in some cp genomes of photosynthetic orchid plants (Kim et al. Citation2015). Orchids like Apostasia odorata, Sobralia callosa, Paphiopedilum armeniacum, and Phragmipedium longifolium retain the complete set of ndh genes, whereas most of the ndh genes in P. equestris, Dendrobium officinale, and D. catenatum were found to be lost in their plastids (Kim et al. Citation2015; Lin et al. Citation2017). In this study, six ndh genes are pseudogenized, and another six ndh genes are missing from the plastid genome of Phalaenopsis zhejiangensis.
Authors contributions
Conceived and designed the experiments: MJ and HJZ. Performed the experiments: MJ, WQ, and ZY. Analyzed the data: HZW and LQP. Contributed to reagents/materials/analysis tools: MJ and WQ. Wrote the paper: MJ and HJZ.
Disclosure statement
The authors declare that they have no competing financial interests or personal relationships that could have appeared to influence the work reported in this paper.
Data availability statement
The data that support the findings of this study are openly available in GenBank of NCBI at https://www.ncbi.nlm.nih.gov/nuccore/MZ326749. The associated BioProject, SRA, and Bio-Sample numbers are PRJNA734444, SRR14710950, and SAMN19491762, respectively.
Additional information
Funding
References
- Bateman RM, Hollingsworth PM, Preston J, Yi-Bo LUO, Pridgeon AM, Chase MW. 2003. Molecular phylogenetics and evolution of Orchidinae and selected Habenariinae (Orchidaceae). Bot J Linn Soc. 142(1):1–40.
- Chang CC, Lin HC, Lin IP, Chow TY, Chen HH, Chen WH, Cheng CH, Lin CY, Liu SM, Chang CC, et al. 2006. The chloroplast genome of Phalaenopsis aphrodite (Orchidaceae): comparative analysis of evolutionary rate with that of grasses and its phylogenetic implications. Mol Biol Evol. 23(2):279–291.
- Chen B, Zhang Y, Cao Y, Zheng Y, Wei Z, Zhao K, Peng D, Zhou Y. 2020. Chloroplast characterizations of a Phalaenopsis native to China, Phalaenopsis mannii (Orchidaceae). Mitochondrial DNA Part B. 5(3):3725–3726.
- Christenhusz MJM, Byng JW. 2016. The number of known plants species in the world and its annual increase. Phytotaxa. 261(3):201–217.
- Christenson EA. 2001. Phalaenopsis—a monograph. Portland: Timber Press.
- Daniell H, Lin CS, Yu M, Chang WJ. 2016. Chloroplast genomes: diversity, evolution, and applications in genetic engineering. Genome Biol. 17(1):134.
- Darriba D, Taboada GL, Doallo R, Posada D. 2012. jModelTest 2: more models, new heuristics and parallel computing. Nat Methods. 9(8):772.
- Deng H, Zhang G-Q, Liu Z-J, Wang Y. 2015. A new species and a new combination of Phalaenopsis (Orchidaceae: Epidendroideae: Aeridinae): evidence from morphological and DNA analysis. Phytotaxa. 238(3):243–254.doi:https://doi.org/10.11646/phytotaxa.238.3.3.
- Dixon KW, Kell SP, Barrett RL, Cribb PJ. 2003. Orchid conservation. Kota Kinabalu: Sabah Natural History Publications.
- Doyle JJ, Doyle JL. 1987. A rapid DNA isolation procedure for small quantities of fresh leaf tissue. Phytochem Bull. 19:11–15.
- Dressler RL. 1993. Phylogeny and classification of the orchid family. Cambridge: Cambridge University Press.
- Gogoi K, Rinya K. 2020. Phalaenopsis arunachalensis sp. nov. (Orchidaceae: Epidendroideae: Aeridinae) A new epiphytic orchid from Arunachal Pradesh, Northeast India. Lankesteriana. 20(3):275–280.
- Goulding SE, Olmstead RG, Morden CW, Wolfe KH. 1996. Ebb and flow of the chloroplast inverted repeat. Mol Gen Genet. 252(1–2):195–206.
- Guindon S, Dufayard JF, Lefort V, Anisimova M, Hordijk W, Gascuel O. 2010. New algorithms and methods to estimate maximum-likelihood phylogenies: assessing the performance of PhyML 3.0. Syst Biol. 59(3):307–321.
- Hadi H, Razali SN, Awadh AI. 2015. A comprehensive review of the cosmeceutical benefits of Vanda species (Orchidaceae). Nat Prod Commun. 10(8):1483–1488.
- Inda LA, Pimentel M, Chase MW. 2012. Phylogenetics of tribe Orchideae (Orchidaceae: Orchidoideae) based on combined DNA matrices: inferences regarding timing of diversification and evolution of pollination syndromes. Ann Bot. 110(1):71–90.
- Ingvarsson PK, Sarah R, Taylor DR. 2003. Molecular evolution of insertions and deletion in the chloroplast genome of silene. Mol Biol Evol. 20(11):1737–1740.
- Jheng CF, Chen TC, Lin JY, Chen TC, Wu WL, Chang CC. 2012. The comparative chloroplast genomic analysis of photosynthetic orchids and developing DNA markers to distinguish Phalaenopsis orchids. Plant Sci. 190:62–73.
- Katoh K, Standley DM. 2013. MAFFT multiple sequence alignment software version 7: improvements in performance and usability. Mol Biol Evol. 30(4):772–780.
- Kim HT, Kim JS, Moore MJ, Neubig KM, Williams NH, Whitten WM, Kim JH. 2015. Seven new complete plastome sequences reveal rampant independent loss of the ndh gene family across orchids and associated instability of the inverted repeat/small single-copy region boundaries. PLOS One. 10(11):e0142215.
- Kim GB, Kwon Y, Yu HJ, Lim KB, Seo JH, Mun JH. 2016. The complete chloroplast genome of Phalaenopsis “Tiny Star”. Mitochondrial DNA Part A. 27(2):1300–1302.
- Ko YZ, Shih HC, Tsai CC, Ho HH, Liao PC, Chiang YC. 2017. Screening transferable microsatellite markers across genus Phalaenopsis (Orchidaceae). Bot Stud. 58(1):48.
- Lee YI, Tseng YF, Lee YC, Chung MC. 2020. Chromosome constitution and nuclear DNA content of Phalaenopsis hybrids. Sci Hortic. 262:109089.
- Li B, Zheng Y. 2018. Dynamic evolution and phylogenomic analysis of the chloroplast genome in Schisandraceae. Sci Rep. 8(1):9285.
- Lin CS, Chen JJW, Chiu CC, Hsiao HCW, Yang CJ, Jin XH, Leebens-Mack J, de Pamphilis CW, Huang YT, Yang LH, et al. 2017. Concomitant loss of NDH complex-related genes within chloroplast and nuclear genomes in some orchids. Plant J. 90(5):994–1006.
- Lohse M, Drechsel O, Kahlau S, Bock R. 2013. OrganellarGenomeDRAW—a suite of tools for generating physical maps of plastid and mitochondrial genomes and visualizing expression data sets. Nucleic Acids Res. 41(Web Server issue):W575–W581.
- Magee AM, Aspinall S, Rice DW, Cusack BP, Sémon M, Perry AS, Stefanović S, Milbourne D, Barth S, Palmer JD, et al. 2010. Localized hypermutation and associated gene losses in legume chloroplast genomes. Genome Res. 20(12):1700–1710.
- Molina J, Hazzouri KM, Nickrent D, Geisler M, Meyer RS, Pentony MM, Flowers JM, Pelser P, Barcelona J, Inovejas SA, et al. 2014. Possible loss of the chloroplast genome in the parasitic flowering plant Rafflesia lagascae (Rafflesiaceae). Mol Biol Evol. 31(4):793–803.
- Nie L, Cui Y, Wu L, Zhou J, Xu Z, Li Y, Li X, Wang Y, Yao H. 2019. Gene losses and variations in chloroplast genome of parasitic plant Macrosolen and phylogenetic relationships within Santalales. Int J Mol Sci. 20(22):5812.
- Park J, Suh Y, Kim S. 2020. A complete chloroplast genome sequence of Gastrodia elata (Orchidaceae) represents high sequence variation in the species. Mitochondrial DNA Part B. 5(1):517–519.
- Patel RK, Jain M. 2012. NGS QC Toolkit: a toolkit for quality control of next generation sequencing data. PLOS One. 7(2):e30619.
- Schuiteman A. 2012. Nothodoritis zhejiangensis transferred to Phalaenopsis. Renziana. 2:48–50.
- Serna-Sánchez MA, Pérez-Escobar OA, Bogarín D, Torres-Jimenez MF, Alvarez-Yela AC, Arcila-Galvis JE, Hall CF, de Barros F, Pinheiro F, Dodsworth S, et al. 2021. Plastid phylogenomics resolves ambiguous relationships within the orchid family and provides a solid timeframe for biogeography and macroevolution. Sci Rep. 11(1):6858.
- Thiel T, Michalek W, Varshney RK, Graner A. 2003. Exploiting EST databases for the development and characterization of gene-derived SSR-markers in barley (Hordeum vulgare L.). Theor Appl Genet. 106(3):411–422.
- Tsai WC, Fu CH, Hsiao YY, Huang YM, Chen LJ, Wang M, Liu ZJ, Chen HH. 2013. OrchidBase 2.0: comprehensive collection of Orchidaceae floral transcriptomes. Plant Cell Physiol. 54(2):e7.
- Tsi ZH. 1989. New taxa of Orchidaceae from China. Bull Bot Res. 9(2):21–32.
- Tsi ZH, Chen SC, Luo YB, Zhu GH. 1999. Flora Reipublicae Popularis Sinicae. Vol. 19. Beijing: Science Press.
- Wang JY, Liu ZJ, Zhang GQ, Peng CC. 2019. The complete chloroplast genome sequence of Phalaenopsis lowii (Orchidaceae). Mitochondrial DNA Part B. 4(2):3569–3570.
- Wicke S, Schneeweiss GM, dePamphilis CW, Müller KF, Quandt D. 2011. The evolution of the plastid chromosome in land plants: gene content, gene order, gene function. Plant Mol Biol. 76(3–5):273–297.
- Wu ZY, Raven PH, Hong DY. 2009. Flora of China. Vol. 25. Beijing and St. Louis: Science Press and Missouri Botanical Garden Press.
- Yao J, Zhao F, Xu Y, Zhao K, Quan H, Su Y, Hao P, Liu J, Yu B, Yao M, et al. 2020. Complete chloroplast genome sequencing and phylogenetic analysis of two Dracocephalum plants. Biomed Res Int. 2020:4374801.
- Yukawa T, Kita K. 2005. Doritis zhejiangensis (Z.H.Tsi). Acta Phytotax Geobot. 56(2):157.
- Zeng S, Chen Z, Wu K, Zhang J, Bai C, Teixeira da Silva JA, Duan J. 2011. Asymbiotic seed germination, induction of calli and protocorm-like bodies, and in vitro seedling development of the rare and endangered Nothodoritis zhejiangensis Chinese orchid. HortScience. 46(3):460–465.
- Zhang Y, Chen B, Zheng Y, Cao Y, Wei Z, Zhao K, Zhou Y. 2020. Characterization of the complete chloroplast genome of Phalaenopsis lobbii (Orchidaceae), an important horticultural plant in China. Mitochondrial DNA Part B. 5:3450–3451.