Abstract
The genus Echinochloa (Poaceae) includes orphan crops and important agricultural weeds. Here, we assembled the complete chloroplast genome of a diploid Echinochloa species (E. haploclada). The chloroplast genome is 139,844 bp in length, which includes a large single copy region (81,893 bp), a small single copy region (12,533 bp) and two separated inverted repeat regions (45,418 bp). A total of 119 unique genes were annotated, consisting of 83 protein-coding genes, 32 tRNA genes and 4 rRNA genes. Hexaploid E. crus-galli, one of the most serious weeds worldwide, was derived from a hybrid between tetraploid E. oryzicola and an unknown diploid species. Based on chloroplast genomes of eight Echinochloa species (varieties), the phylogenetic analysis showed that E. crus-galli clustered firstly with diploid E. haploclada rather than tetraploid E. oryzicola, supporting previous assumption that E. oryzicola is the paternal donor of E. crus-galli.
The genus Echinochloa (Poaceae) includes orphan crops (barnyard millet) and many problematic weeds in agricultural fields, e.g. hexaploid E. crus-galli (the dominant Echinochloa species) is one of the most detrimental weeds in rice paddies (Ye and Fan Citation2021). Chloroplast genome sequences are useful for understanding plant origin and evolution. So far, there are at least eight Echinochloa species (varieties) with released chloroplast genomes (NCBI Organelle Genome Resources), e.g. hexaploid E. crus-galli, tetraploid E. oryzicola and hexaploid E. colona (Ye et al. Citation2014; Nah et al. Citation2016; Perumal et al. Citation2016; Lee et al. Citation2017; Piot et al. Citation2018). Among them, however, the diploid species are still lacking, which hinders our understanding of the evolution of Echinochloa species.
In this study, we assembled the complete chloroplast genome of a diploid Echinochloa species, E. haploclada, which was collected in Kenya, near Muhaka (04°20.201 S, 39°28.137 E) and deposited in the Herbarium of Zhejiang University (accession number HZU60206921), based on whole-genome high-throughput sequencing data generated by us previously (Ye et al. Citation2020). After quality control with NGSQCToolkit v2.3 (Patel and Jain Citation2012), the clean data was applied in de novo assembly by NOVOPlasty v3.6 (Dierckxsens et al. Citation2017) using the Panicum virgatum (neighboring genus of Echinochloa) complete chloroplast genome (GenBank accession number NC_015990) as a reference. Genome annotation was performed by the GeSeq online (Tillich et al. Citation2017). The assembled genome sequences and annotation information have been submitted in National Genomics Data Center (NGDC, China) under accession number GWHBAUW01000000 and Genbank under accession number MW672445.1.
The total length of E. haploclada chloroplast genome is 139,844 bp. Similar to most angiosperm chloroplast genomes, this genome exhibited a distinct quadripartite structure, including a pair of inverted repeats (IRa and IRb, 22,709 bp each), the large single-copy region (LSC, 81,893 bp) and the small single-copy region (SSC, 12,533 bp). The GC contents of the IR, LSC and SSC regions are 36.4%, 33.1%, and 44.0%, respectively. A total of 119 unique genes were annotated and 24 genes, including 10 protein-coding genes, 8 tRNA genes and 4 rRNA genes, were duplicated in the IR regions. Among these 119 genes, there are 83 protein-coding genes, 32 tRNA genes and 4 rRNA genes, and 7 genes contained introns (6 and 1 genes contained 1 and 2 introns, respectively).
To investigate the evolutionary position of E. haploclada among Echinochloa species, we built a phylogenetic tree of eight Echinochloa species (varieties) and four other sister groups (Alloteropsis, Panicum, Setaria and Digitaria) based on complete chloroplast genome sequences using Oryza sativa as an outgroup. We first performed alignment by MAFFT v7.310 (Katoh et al. Citation2002) with the parameter ‘auto’ (‘FFT-NS-2’ was finally assigned by MAFFT). Then, IQ-tree v1.6.12, an effective algorithm for estimating maximum-likelihood phylogenies, was used to construct a phylogenetic tree with recommended setting ‘-m MFP -bb 1000 -bnni’ (GTR + F+R4 model was finally selected) (Nguyen et al. Citation2015). Finally, the tree was illustrated and modified using iTOL (Letunic and Bork Citation2019).
The phylogeny showed that E. haploclada first clustered with E. crus-galli forming a monoclade (). Compared to tetraploid E. oryzicola, diploid E. haploclada showed closer relationship to hexaploid E. crus-galli. The dominant hexaploid species E. crus-galli was arisen from the hybridization between tetraploid E. oryzicola and an unknown diploid species. It has been assumed that E. oryzicola is the paternal donor based on nuclear DNA internal transcribed spacer and chloroplast DNA segments (Aoki and Yamaguchi Citation2008). Additionally, our previous study revealed that E. haploclada is not but is very close to the direct ancestor of E. crus-galli based on nuclear genome sequences (Ye et al. Citation2020). Therefore, considering the maternal inheritance of chloroplast genome, the phylogenetic analysis in this study supported that tetraploid E. oryzicola is the paternal donor while an unknown diploid species (close to E. haploclada) is the maternal donor of hexaploid E. crus-galli.
Figure 1. Maximum-likelihood phylogenetic tree of eight Echinochloa species (varieties) based on complete chloroplast genomes (E. ugandensis, NC_036127; E. colona, KT983255; E. frumentacea, KU242342; E. oryzicola, KJ000048; E. stagnina, MF563381; E. crus-galli var. grus-galli, KJ000047; E.crus-galli var. praticola, KR822686; A. angusta, KX752090; A. cimicina, MT950760; A. paniculata, NC_032078; A. semialata, MT950759.1; D. exilis, NC_024176; D. glauca, MK593550; S. italica, KJ001642; S. viridis, NC_028075; P. miliaceum, NC_029732; P. sumatrense, NC_032378; P.virgatum, NC_015990) with O. sativa as an outgroup (NC_031333). Bootstrap support value from 1000 replicates is shown on each node.
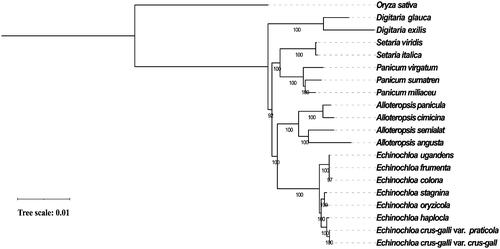
Disclosure statement
No potential conflict of interest was reported by the author(s).
Data availability statement
The genome sequence data that support the findings of this study are openly available in GenBank of NCBI at [https://www.ncbi.nlm.nih.gov] (https://www.ncbi.nlm.nih.gov/) under the accession no. MW672445.1. The associated BioProject, SRA, and Bio-Sample numbers are PRJNA752977, SAMN20668670, and SRS9707358 respectively.
Additional information
Funding
References
- Aoki D, Yamaguchi H. 2008. Genetic relationship between Echinochloa crus-galli and Echinochloa oryzicola accessions inferred from internal transcribed spacer and chloroplast DNA sequences. Weed Biol Manag. 8(4):233–242.
- Dierckxsens N, Mardulyn P, Smits G. 2017. NOVOPlasty: de novo assembly of organelle genomes from whole genome data. Nucleic Acids Res. 45(4):e18.
- Katoh K, Misawa K, Kuma KI, Miyata T. 2002. MAFFT: a novel method for rapid multiple sequence alignment based on fast Fourier transform. Nucleic Acids Res. 30(14):3059–3066.
- Lee J, Kim JW, Lee IY, Ahn JH. 2017. Comparison of the complete genomes of two Echinochloa species: barnyard grass and jungle rice. Mitochondrial DNA Part B. 2(2):512–513.
- Letunic I, Bork P. 2019. Interactive Tree Of Life (iTOL) v4: recent updates and new developments. Nucleic Acids Res. 47(W1):W256–W259. doi:https://doi.org/10.1093/nar/gkz239.
- Nah G, Im JH, Kim JW, Kim K, Lim JS, Choi AY, Cho IY, Yang TJ, Park TS, Lee DK, et al. 2016. The complete chloroplast genomes of three Korean Echinochloa crus-galli accessions. Mitochondrial DNA Part A. 27(6):4357–4358.
- Nguyen LT, Schmidt HA, Haeseler A, Minh BQ. 2015. IQ-TREE: A fast and effective stochastic algorithm for estimating maximum-likelihood phylogenies. Mol Biol Evol. 32(1):268–274.
- Patel RK, Jain M. 2012. NGS QC Toolkit: a toolkit for quality control of next generation sequencing data. PLoS One. 7(2):e30619.
- Perumal S, Jayakodi M, Kim DS, Yang T, Natesan S. 2016. The complete chloroplast genome sequence of Indian barnyard millet, Echinochloa frumentacea (Poaceae). Mitochondrial DNA Part B. 1(1):79–80.
- Piot A, Hackel J, Christin PA, Guillaume B. 2018. One-third of the plastid genes evolved under positive selection in PACMAD grasses. Planta. 247(1):255–266.
- Tillich M, Lehwark P, Pellizzer T, Ulbricht ES, Fischer A, Bock R, Greiner S. 2017. GeSeq – versatile and accurate annotation of organelle genomes. Nucleic Acids Res. 45(W1):W6–W11.
- Ye CY, Fan LJ. 2021. Orphan crops and their wild relatives in the genomic era. Mol Plant. 14(1):27–39.
- Ye CY, Lin ZX, Li GM, Wang YY, Qiu J, Fu F, Zhang HQ, Chen L, Ye SS, Song WJ, Jin GL, Zhu JW, et al. 2014. Echinochloa chloroplast genomes: insights into the evolution and taxonomic identification of two weedy species. PLoS One. 9(11):e113657.
- Ye CY, Wu DY, Mao LF, Jia L, Qiu J, Lao ST, Chen MH, Jiang BW, Tang W, Peng Q, et al. 2020. The genomes of the allohexaploid Echinochloa crus-galli and its progenitors provide insights into polyploidization-driven adaptation. Mol Plant. 13(9):1298–1310.