Abstract
We report the complete circular mitochondrial genome of the hydrothermal vent polychaete Paralvinella palmiformis (Annelida: Terebellida: Alvinellidae). The mitochondrial genome is 16386 bp in length with a GC content of 38.8%. It contains 36 genes, including 13 protein-coding sequences, 2 rRNA and 21 tRNA genes.
The family Alvinellidae (Annelida:Terebellida, Desbruyères and Laubier Citation1986) contains 11 species all endemic to deep-sea hydrothermal vent ecosystems. Nine species are found in eastern Pacific Ocean, two in the western Pacific, and one in the Indian ocean (Desbruyères and Laubier Citation1989, Citation1993; Han et al. Citation2021). Interestingly, the alvinellid family includes several hyper-thermophilic species which live just centimeters away from hydrothermal discharge reaching up to 300 °C. This unique adaptation appears to have evolved multiple times in the group (Fontanillas et al. Citation2017) and is speculated to reduce resource competition. Indeed, hyperthermophile species are found in sympatry with other less thermo-tolerant species (Desbruyères and Laubier Citation1982; McHugh Citation1989; Dilly et al. Citation2012). The wide biogeographic distribution of these worms, despite the sporadicity of their habitat and their convergent adaptation to extreme thermal and oxygen conditions, raises questions about their evolutive history. Owing to a lack of genetic data, the alvinellid phylogeny is yet to be fully resolved. Furthermore, there are important knowledge gaps about the physiology of the mesophile species.
Here, we report the complete mitochondrial genome of Paralvinella palmiformis (Desbruyères & Laubrier, 1986), which constitutes with the hyperthermophile Paralvinella sulfincola a well-studied model for thermal adaption. These two species inhabit hydrothermal vents of the northeastern Pacific ocean and have a predator/scavenger lifestyle (Tunnicliffe et al. Citation1993; Grelon et al. Citation2006; Lelièvre et al. Citation2017) but they preferentially occupy different positions on the sulfide chimneys (Sarrazin et al. Citation1999). Paralvinella sulfincola builds mucous houses high on the sulfide accretions whereas P. palmiformis typically lives further away from the hydrothermal output. Experimental evidence shows that P. palmiformis thermal preference is between 30 and 40 °C; about 10 °C lower than that of P. sulfincola. P. palmiformis also has a smaller thermal tolerance (0–45 °C vs. 2–63 °C for P. sulfincola (Dilly et al. Citation2012; Lee Citation2003; Rinke and Lee Citation2009)).
One P. palmiformis specimen was collected at Endeavor on the Juan de Fuca ridge (47.949993 N 129.096988 W; depth= −2197 m) with the ROV ROPOS during an expedition on board the GSS Tully in June 2016. DNA was extracted from the body wall tissues preserved in 95% ETOH with the phenol-chloroform method followed by ethanol precipitation (Sambrook et al. Citation1989) and purified with the Genomic DNA Clean & Concentrator-10 kit (ZYMO Research, CA, USA). DNA was then sent for sequencing on the Illumina NovaSeq and Oxford Nanopore platforms ultimately yielding 485973, and 5964 mitochondrial reads, respectively. The Illumina library was trimmed with trimmomatic v0.30 (Bolger et al. Citation2014) (parameters: leading = 10, trailing = 10, minlen = 50) and two rounds of binning with bbmap (Bushnell Citation2014) tool bbsplit.sh followed by assembly with SPAdes v3.11.1 (Bankevich et al. Citation2012) were used to recover a draft assembly of P. palmifomis mitogenome. The publicly available mitochondrial genomes of two closely-related species, P. sulfincola (FJ976042.1) and P. hessleri (MK192098.1), were used to recover mitochondrial reads in the first round.
Nanopore reads corrected with FMLRC v2 (Wang et al. Citation2018) were then mapped to the draft assembly with minimap2 v2.17 (Li Citation2018), extracted with bbmap’s filterbyname.sh tool, and assembled into a single circular chromosome (average coverage = 817) with Canu v2.1.1 (Koren et al. Citation2017). The final assembly was polished with one round of Pilon v1.23 (Walker et al. Citation2014). P. palmiformis mitochondrial genome is 16,386 bp in length with a GC content of 38.8%. Gene and tRNA predictions were performed in GeSeq using Arwen v1.2.3 (Laslett and Canbäck Citation2008) for tRNA prediction and Mitos2 webserver (Bernt et al. Citation2013). The resulting annotations were manually curated in Geneious (Kearse et al. Citation2012). This mitochondrial genome was deposited in GenBank under the following accessions (BioProject: PRJNA786438, BioSample: SAMN23672631, Accession: OL802212). The P. palmiformis specimen and its DNA were deposited in the archives of the Deep-Sea Omics lab, Hong Kong University of Science and Technology under the accession P08H-3 1. Additional mitochondrial gene sequences from another P. palmiformis individual and from the species A. caudata, A. pompejana, P. fijiensis, P. grasslei, and P. pandorae, were recovered from transcriptomic data (Fontanillas et al. Citation2017; Stiller et al. Citation2020) by assembling the mitochondrial bins of their respective transcriptomes with Trinity and SPAdes.
The mitogenome of P. palmiformis has the same gene order and composition as P. sulfincola and P. hessleri with the exception of one tRNA which was not annotated in P. hessleri. The alvinellid phylogeny based on the concatenated alignment of 12 protein-coding genes (8,643 complete nucleotides, ) is consistent with all previously reported phylogenies based on genetic, morphologic and transcriptomic data for this group (Zhong et al. Citation2011; Fontanillas et al. Citation2017; Stiller et al. Citation2020) and places P. palmiformis as a sister species to P. grasslei, which is an alvinellid from the East Pacific Rise occupying a similar ecological niche (). It is possible that the two species had speciated due to connectivity barriers created by the fragmentation of the Farallon plate spreading ridge about 30Mya (Tunnicliffe Citation1988). Concordance analyses with Bucky (Larget et al. Citation2010) revealed topological variation in two genomic regions, that is, from cox2 to cox3 and from nad5 to rrnS. The maximum likelihood topology in these regions places P. pandorae as a taxon basal to all alvinellids whereas P. pandorae is basal to the genus Alvinella according to the rest of the coding mitochondrial genome.
Figure 1. Maximum likelihood phylogeny (GTR nucleotide substitution model) using a 10,665 bp alignment of 12 mitochondrial protein coding genes. The outgroups are species of the polychaete families Terebellidae (P. cristata) and Amphraetidae (E. vanelli). The mitogenome sequenced in this study is indicated in bold. Numbers below nodes are the bootstrap values.
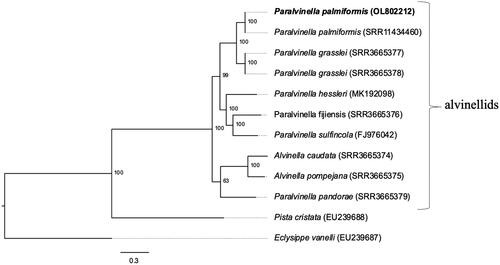
Ethical approval
No ethical approval was required because the study specimen does not belong to the list of regulated invertebrates.
Author contributions
MP designed the study, analyzed the data and drafted the manuscript, HW and BA critically revised the manuscript, P-YQ conceived the study and critically revised the manuscript. All authors have approved the final version of the paper and agreed to be accountable for all aspects of the work.
Acknowledgments
We would like to thank Verena Tunnicliffe, Rachel Boschen-Rose and the crews of the CCGS John P. Tully and ROV ROPOS for providing the P. palmiformis specimen.
Disclosure statement
No potential conflict of interest was reported by the author(s).
Data availability statement
This mitochondrial genome was deposited in GenBank of NCBI under the following accessions (BioProject: PRJNA786438, BioSample: SAMN23672631, Accession: OL802212). The P. palmiformis specimen and its DNA were deposited in the archives of the Deep-Sea Omics lab, Hong Kong University of Science and Technology under the accession P08H-3 (contact: Eric Lau, [email protected]). Additional data that support the findings of this study (e.g., gene sequence alignments) are openly available at https://github.com/maepz/Ppalmiformis_mitogenome.
Correction Statement
This article has been republished with minor changes. These changes do not impact the academic content of the article.
Additional information
Funding
Notes
1 Collection manager: Eric Lau, [email protected]
References
- Bankevich A, Nurk S, Antipov D, Gurevich AA, Dvorkin M, Kulikov AS, Lesin VM, Nikolenko SI, Pham S, Prjibelski AD, et al. 2012. SPAdes: a new genome assembly algorithm and its applications to single-cell sequencing. Journal of Computational Biology. 19(5):786–477.
- Bernt M, Donath A, Jühling F, Externbrink F, Florentz C, Fritzsch G, Pütz J, Middendorf M, Stadler PF. 2013. MITOS: Improved de novo metazoan mitochondrial genome annotation. Mol Phylogenet Evol. 69(2):313–319.
- Bolger AM, Lohse M, Usadel B. 2014. Trimmomatic: a flexible trimmer for Illumina sequence data. Bioinformatics. 30(15):2114–2120.
- Bushnell B. 2014. BBMap: a fast, accurate, splice-aware aligner. Berkeley, CA (United States): Lawrence Berkeley National Lab.(LBNL).
- Desbruyères D, Laubier L. 1986. Les Alvinellidae, une famille nouvelle d'annélides polychètes inféodées aux sources hydrothermales sous-marines: systématique, biologie et écologie. Can J Zool. 64(10):2227–2245. doi:https://doi.org/10.1139/z86-337.
- Desbruyères D, Laubier L. 1982. Paralvinella grasslei, new genus, new species of Alvinellinae (Polychaeta: Ampharetidae) from the Galapagos rift geothermal vents. Vol. 95. Proceedings of the Biological Society of Washington, Washington. p. 484–494.
- Desbruyères D, Laubier L. 1989. Paralvinella hessleri, new species of Alvinellidae (Polychaeta) from the Mariana back-arc basin hydrothermal vents. Vol. 102. Proceedings of the Biological Society of Washington, Washington. p. 761–767.
- Desbruyères D, Laubier L. 1993. New species of Alvinellidae (Polychaeta) from the North Fiji back-arc basin hydrothermal vents (southwestern Pacific). Vol. 106. Proceedings of the Biological Society of Washington, Washington. p. 225–236.
- Dilly GF, Young CR, Lane WS, Pangilinan J, Girguis PR. 2012. Exploring the limit of metazoan thermal tolerance via comparative proteomics: thermally induced changes in protein abundance by two hydrothermal vent polychaetes. Proceedings of the Royal Society of London B: Biological Sciences, London. rspb20120098.
- Fontanillas E, Galzitskaya OV, Lecompte O, Lobanov MY, Tanguy A, Mary J, Girguis PR, Hourdez S, Jollivet D. 2017. Proteome evolution of deep-Sea hydrothermal vent alvinellid polychaetes supports the ancestry of thermophily and subsequent adaptation to cold in some lineages. Genome Biol Evol. 9(2):279–296.
- Grelon D, Morineaux M, Desrosiers G, Juniper SK. 2006. Feeding and territorial behavior of Paralvinella sulfincola, a polychaete worm at deep-sea hydrothermal vents of the Northeast Pacific Ocean. J Exp Marine Biol Ecol. 329(2):174–186.
- Han Y, Zhang D, Wang C, Zhou Y. 2021. Out of the Pacific: a new alvinellid worm (Annelida: Terebellida) from the northern Indian Ocean hydrothermal vents. Front Mar Sci. 8:8.
- Kearse M, Moir R, Wilson A, Stones-Havas S, Cheung M, Sturrock S, Buxton S, Cooper A, Markowitz S, Duran C, et al. 2012. Geneious Basic: an integrated and extendable desktop software platform for the organization and analysis of sequence data. Bioinformatics. 28(12):1647–1649.
- Koren S, Walenz BP, Berlin K, Miller JR, Bergman NH, Phillippy AM. 2017. Canu: scalable and accurate long-read assembly via adaptive k-mer weighting and repeat separation. Genome Res. 27(5):722–736.
- Larget BR, Kotha SK, Dewey CN, Ané C. 2010. BUCKy: Gene tree/species tree reconciliation with Bayesian concordance analysis. Bioinformatics. 26(22):2910–2911.
- Laslett D, Canbäck B. 2008. ARWEN: A program to detect tRNA genes in metazoan mitochondrial nucleotide sequences. Bioinformatics. 24(2):172–175.
- Lee RW. 2003. Thermal tolerances of deep-sea hydrothermal vent animals from the northeast Pacific. Biol Bull. 205(2):98–101.
- Lelièvre Y, Legendre P, Matabos M, Mihály S, Lee RW, Sarradin P-M, Arango CP, Sarrazin J. 2017. Astronomical and atmospheric impacts on deep-sea hydrothermal vent invertebrates. Proc Biol Sci. 284(1852):20162123.
- Li H. 2018. Minimap2: pairwise alignment for nucleotide sequences. Bioinformatics. 34(18):3094–3100.
- McHugh D. 1989. Population structure and reproductive biology of two sympatric hydrothermal vent polychaetes, Paralvinella pandorae and P. palmiformis. Marine Biology. 103(1):95–106.
- Rinke C, Lee RW. 2009. Pathways, activities and thermal stability of anaerobic and aerobic enzymes in thermophilic vent paralvinellid worms. Mar Ecol Prog Ser. 382:99–112.
- Sambrook J, Fritsch EF, Maniatis T. 1989. Molecular cloning: a laboratory manual. New York, NY: Cold Spring Harbor Laboratory Press..
- Sarrazin J, Juniper SK, Massoth G, Legendre P. 1999. Physical and chemical factors influencing species distributions on hydrothermal sulfide edifices of the Juan de Fuca Ridge, northeast Pacific. Mar Ecol Prog Ser. 190:89–112.
- Stiller J, Tilic E, Rousset V, Pleijel F, Rouse GW. 2020. Spaghetti to a Tree: A robust phylogeny for Terebelliformia (Annelida) based on transcriptomes, molecular and morphological data. Biology. 9(4):73.
- Tunnicliffe V, Desbruyères D, Jollivet D, Laubier L. 1993. Systematic and ecological characteristics of Paralvinella sulfincola Desbruyères and Laubier, a new polychaete (family Alvinellidae) from northeast Pacific hydrothermal vents. Can J Zool. 71(2):286–297.
- Tunnicliffe V. 1988. Biogeography and evolution of hydrothermal-vent fauna in the eastern Pacific Ocean. Vol. 233. Proceedings of the Royal Society of London B: Biological Sciences, London, p. 347–366.
- Walker BJ, Abeel T, Shea T, Priest M, Abouelliel A, Sakthikumar S, Cuomo CA, Zeng Q, Wortman J, Young SK, et al. 2014. Pilon: an integrated tool for comprehensive microbial variant detection and genome assembly improvement. PLOS One. 9(11):e112963.
- Wang JR, Holt J, McMillan L, Jones CD. 2018. FMLRC: Hybrid long read error correction using an FM-index. BMC Bioinformatics. 19(1):50.
- Zhong M, Hansen B, Nesnidal M, Golombek A, Halanych KM, Struck TH. 2011. Detecting the symplesiomorphy trap: a multigene phylogenetic analysis of terebelliform annelids. BMC Evol Biol. 11:369.