Abstract
Aphaenogaster japonica (Forel, 1911) is an omnivorous ant that is widely distributed in eastern Asia. The mitochondrial genome of A. japonica reported here was 18,607 bp in length, consisting of 13 protein-coding genes, two ribosomal RNA genes, 22 transfer RNA genes, and a control region. The base composition was AT biased (the GC ratio is 18.9%). With A. japonica added, we obtain weak evidence that the sister group of the Stenammini group, including Aphaenogaster, is the Myrmicini group. Therefore, the Stenammini and Myrmicini groups may be not a robust monophyletic group, unlike the previous results based on the complete mitochondrial genome.
Aphaenogaster is a genus of elongated, slender ants containing 210 extant species distributed all over the world except in sub-Saharan Africa and South America (Kiran et al. Citation2013; Park et al. Citation2020). In North America (Giladi Citation2006; Ness et al. Citation2009) and East Asia (Higashi et al. Citation1989), Aphaenogaster species are often herb seed dispersers in temperate forests. Based on nuclear gene fragments, Aphaenogaster is within the Stenammini group with Stenamma, Messor, Goniomma and Oxyopomyrmex, while this group is sister to all myrmicines except the Myrmicini and Pogonomyrmecini groups (Ward et al. Citation2015). However, based on the complete mitochondrial genome, Stenammini and Myrmicini are grouped (Park et al. Citation2020; Sang et al. Citation2022; Yin et al. Citation2022). Aphaenogaster japonica is a common species found in eastern Asia and is currently more abundant in lowlands (Kwon et al. Citation2014) but is expected to increase in highlands in the future due to global warming (Kwon Citation2016). To better understand its phylogenetic relationships, we present the complete mitochondrial genome of A. japonica.
In this study, Aphaenogaster japonica workers were collected from Nanchong City (30°48′54.07″N, 106°4′11.08″E), Sichuan Province, China, in October 2020. All sample collection schemes in this study were approved by the Research Ethics Committee of China West Normal University (approval reference: CWNU2020D002). After morphological identification, we kept the voucher specimens (voucher number: NCAJ202010) in the Key Laboratory of Southwest China Wildlife Resources Conservation of China West Normal University (www.cwnu.edu.cn; Contact: Zhao-Min ZHOU, [email protected]). We extracted and sequenced the total genomic DNA of A. japonica with the Illumina Novaseq sequencing platform (Shanghai Personal Biotechnology Co. Ltd., Shanghai, China). The software A5-miseq v20150522 (Coil et al. Citation2015) and SPAdes V3.9.0 (Bankevich et al. Citation2012) were used to carry out the genome de novo assembly. We annotated the mitochondrial genome on the MITOS Web Server (Bernt et al. Citation2013). Finally, the sequence information of A. japonica was submitted to GenBank (accession number: MW915466).
The complete mitogenome of A. japonica was 18,607 bp (GC content is 18.9%) in size, consisting of 13 protein-coding genes (PCGs), two rRNA genes, 22 tRNA genes, and a control region. The base composition was 41.0% A, 13.4% C, 5.5% G, and 40.0% T. All PCGs started with ATN (five ATG, four ATT, and four ATA) and ended with TAA. The tRNA size ranged from 52 to 80 bp, which is similar to those of other ant species (54–90 bp) (Idogawa et al. Citation2021). Four PCGs (ND5, ND4, ND4L, and ND1) and 10 RNAS (tRNA-V, Q, C, Y, F, H, P, L1 and rRNA-L, S) were encoded by the majority strand (J-strand), whereas the others were located on the minority strand (N-strand). The lengths of 12S and 16S rRNA were 936 and 1589 bp, respectively. The gene order of A. japonica is consistent with the putative ancestral arrangement of Myrmicinae species (Babbucci et al. Citation2014; Vieira and Prosdocimi Citation2019).
Phylogenetic relationships were inferred from 30 ants, including A. japonica, and an outgroup species, Apis mellifera ligustica. We aligned 13 PCGs and two rRNA genes by MAFFT 7.450 (Katoh and Standley Citation2013) and concatenated them in PhyloSuite v1.2.2 (Zhang et al. Citation2020). The best-fit model determined by ModelFinder was GTR + F+I + G4 (Kalyaanamoorthy et al. Citation2017). The maximum-likelihood and neighbor-joining trees were made by MEGA X (Kumar et al. Citation2018), and a Bayesian inference tree was constructed using MrBayes 3.2.6 (Ronquist et al. Citation2012). The phylogenetic trees showed that A. japonica and A. famelica are grouped with high support values, which may have been caused by high similarity between two mitogenomes in the same genus (). Stenammini and Myrmicini were grouped in two of the three trees (), which is congruent with the results based on the complete mitochondrial genome (Park et al. Citation2020; Sang et al. Citation2022; Yin et al. Citation2022), but the support values of the monophyletic relationship were much lower.
Figure 1. Maximum-likelihood, neighbor-joining, and Bayesian’s inference phylogenetic trees showing phylogenetic relationships among 30 ant species. Apis mellifera ligustica was used as an outgroup. A phylogenetic tree was constructed based on the maximum-likelihood phylogenetic tree. The numbers at nodes indicate bootstrap support values for maximum-likelihood and neighbor-joining trees and posterior probability for the Bayesian inference tree, in order.
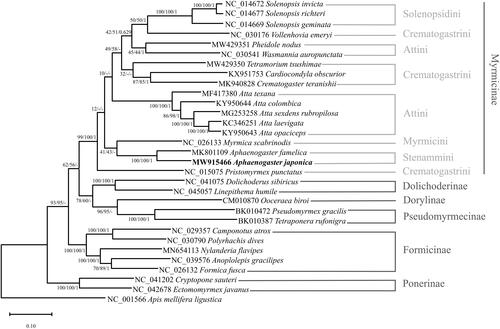
Author contributions
All authors contributed to the study conception and design. Analysis and interpretation of the data were performed by Xin-Yu Luo, Ru-Yi Yin, and Xiang-Qin Huang. The first draft of the manuscript was written by Xin-Yu Luo and Ru-Yi Yin. Yi Luo and Zhao-Min Zhou revised it critically for intellectual content, and all authors commented on previous versions of the manuscript. All authors agree to be accountable for all aspects of the work.
Disclosure statement
No potential conflict of interest was reported by the author(s).
Data availability statement
The data that support the findings of this study are openly available in GenBank of NCBI at https://www.ncbi.nlm.nih.gov, reference number MW915466. The associated BioProject, SRA, and Bio-Sample numbers are PRJNA814821, SRX14435162, and SAMN26567376, respectively.
Additional information
Funding
References
- Babbucci M, Basso A, Scupola A, Patarnello T, Negrisolo E. 2014. Is it an ant or a butterfly? Convergent evolution in the mitochondrial gene order of Hymenoptera and Lepidoptera. Genome Biol Evol. 6(12):3326–3343.
- Bankevich A, Nurk S, Antipov D, Gurevich AA, Dvorkin M, Kulikov AS, Lesin VM, Nikolenko SI, Pham S, Prjibelski AD, et al. 2012. SPAdes: a new genome assembly algorithm and its applications to single-cell sequencing. J Comput Biol. 19(5):455–477.
- Bernt M, Donath A, Jühling F, Externbrink F, Florentz C, Fritzsch G, Pütz J, Middendorf M, Stadler PF. 2013. MITOS: improved de novo metazoan mitochondrial genome annotation. Mol Phylogenet Evol. 69(2):313–319.
- Coil D, Jospin G, Darling AE. 2015. A5-miseq: an updated pipeline to assemble microbial genomes from Illumina MiSeq data. Bioinformatics. 31(4):587–589.
- Giladi I. 2006. Choosing benefits or partners: a review of the evidence for the evolution of myrmecochory. Oikos. 112(3):481–492.
- Higashi S, Tsuyuzaki S, Ohara M, Ito F. 1989. Adaptive advantages of ant-dispersed seeds in the myrmecochorous plant Trillium tschonoskii (Liliaceae). Oikos. 54(3):389–394.
- Idogawa N, Lee CC, Yang CCS, Dobata S. 2021. The complete mitochondrial genome of a parthenogenetic ant, Monomorium triviale (Hymenoptera: Formicidae). Mitochondrial DNA B Resour. 6(10):2793–2795.
- Kalyaanamoorthy S, Minh BQ, Wong TKF, Von Haesele A, Jermiin LS. 2017. ModelFinder: fast model selection for accurate phylogenetic estimates. Nat Methods. 14(6):587–589.
- Katoh K, Standley DM. 2013. MAFFT multiple sequence alignment software version 7: improvements in performance and usability. Mol Biol Evol. 30(4):772–780.
- Kiran K, Alipanah H, Paknia O. 2013. A new species of the ant genus Aphaenogaster Mayr (Hymenoptera: Formicidae) from Iran. Asian Myrmecol. 5:45–51.
- Kumar S, Stecher G, Li M, Knyaz C, Tamura K. 2018. MEGA X: molecular evolutionary genetics analysis across computing platforms. Mol Biol Evol. 35(6):1547–1549.
- Kwon TS, Lee CM, Park J, Kim SS, Chun JH, Sung JH. 2014. Prediction of abundance of ants due to climate warming in South Korea. J Asia Pac Biodivers. 7(2):e179–e196.
- Kwon TS. 2016. Foraging activity of competing ants along altitudinal gradient on a high mountain, South Korea. J Asia Pac Entomol. 19(1):95–101.
- Ness JH, Morin DF, Giladi I. 2009. Uncommon specialization in a mutualism between a temperate herbaceous plant guild and an ant: are Aphaenogaster ants keystone mutualists? Oikos. 118(12):1793–1804.
- Park J, Xi H, Park J. 2020. The complete mitochondrial genome of Aphaenogaster famelica (Smith, 1874) (Hymenoptera: Formicidae). Mitochondrial DNA B Resour. 5(1):492–494.
- Ronquist F, Teslenko M, van der Mark P, Ayres DL, Darling A, Hohna S, Larget B, Liu L, Suchard MA, Huelsenbeck JP. 2012. MrBayes 3.2: efficient Bayesian phylogenetic inference and model choice across a large model space. Syst Biol. 61(3):539–542.
- Sang Y, Yin RY, Luo Y, Zhou ZM. 2022. The complete mitochondrial genome of Pheidole nodus (Smith, 1874) (Hymenoptera: Formicidae). Mitochondrial DNA B Resour. 7(3):451–453.
- Vieira GA, Prosdocimi F. 2019. Accessible molecular phylogenomics at no cost: obtaining 14 new mitogenomes for the ant subfamily Pseudomyrmecinae from public data. PeerJ. 7:e6271.
- Ward PS, Brady SG, Fisher BL, Schultz TR. 2015. The evolution of myrmicine ants: phylogeny and biogeography of a hyperdiverse ant clade (Hymenoptera: Formicidae). Syst Entomol. 40(1):61–81.
- Yin RY, Luo Y, Zhou ZM. 2022. The complete mitochondrial genome of Tetramorium tsushimae (Emery, 1925) (Hymenoptera: Formicidae). Mitochondrial DNA B Resour. 7(1):40–42.
- Zhang D, Gao FL, Jakovlić I, Zou H, Zhang J, Li WX, Wang GT. 2020. PhyloSuite: an integrated and scalable desktop platform for streamlined molecular sequence data management and evolutionary phylogenetics studies. Mol Ecol Resour. 20(1):348–355.