Abstract
Crataegus scabrifolia (Franch.) Rehd is a medicinal and edible plant in Southwest China. The chloroplast genome of C. scabrifolia was analyzed by high-throughput sequencing technology, and its genetic relationship to related species was discussed. The chloroplast genome is 159,637 bp long, with two inverted repeat (IR) regions (26,384 bp each) that separate a large single-copy (LSC) region (87,730 bp) and a small single-copy (SSC) region (19,139 bp). A total of 127 genes were annotated, including 83 protein-coding genes, 8 rRNA genes, and 36 tRNA genes. The phylogenetic tree shows that C. hupehensis is closely related to C. scabrifolia with strong bootstrap support.
Introduction
Crataegus scabrifolia (Franch.) Rehd (1890) is a deciduous plant belonging to the genus Crataegus of the Rosaceae family (). It is mainly distributed in the Yunnan Provinces, Guizhou Provinces, and Sichuan Provinces of China (Wu Citation1974). It grows in hillside mixed forests or secondary shrubs or forest margins at an altitude of 800–2400 m (Zhu et al. Citation2021). Crataegus plants contain various plant components, such as sugars and sugar alcohols, flavonoids, terpenoids, phenylpropanoids, steroids, monosteroids, sesquiterpenes, lignans, hydroxycinnamic acid, organic acids, and nitrogen compounds (Jurikova et al. Citation2012). It has various pharmacological uses, such as anti-hyperlipidemia, anti-hypertension, anti-oxidation, anti-inflammation, antibacterial, anti-cancer, anti-cardiac remodeling, anti-coagulation and anti-thrombus, anti-angiotensin converting enzyme, anti-arrhythmic, and anti-cataract (Martinelli et al. Citation2021). The medicinal parts of C. scabrifolia are mainly the fruit, but its pulp, leaves, and seeds can also be used as medicine (Wang et al. Citation2013; Kim et al. Citation2022;). As a medicinal and edible plant, there are few reports on the research of C. scabrifolia at present, which only reported its chemical constituents (Dahmer and Scott Citation2010). In this study, we characterized a complete chloroplast genome of C. scabrifolia and confirmed the phylogenetic relationship of the genus to provide an important basis for further study of the phylogenetic relationship and genetic diversity of Crataegus.
Figure 1. Plant morphological characteristics of Crataegus scabrifolia. (A) Leaves of this species ovate-lanceolate to ovate-elliptic. (B) The flowers of C. scabrifolia are corymbos or compound corymbos. The photos of C. scabrifolia were taken by the authors in Luoping Mountain, Eryuan County, Yunnan Province, China (coordinates: 99°52′19.15″E, 25°59′53.34″N).
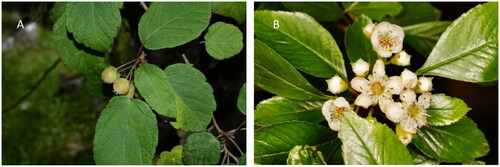
Materials and methods
DNA extraction and sequencing
Fresh leaves of C. scabrifolia were collected from Luoping Mountain, Eryuan County, Dali Bai Autonomous Prefecture, Yunnan Province, China (coordinates: 99°52′19.15″E, 25°59′53.34″N; altitude: 1900 m). The collection of specimens in this study did not require special permits. This research was conducted in accordance with relevant Chinese laws. A voucher specimen (SWFU20210783MFY) was deposited in the Herbarium of Southwest Forestry University, China (http://bbg.swfu.edu.cn/, Yu Xiao, email: [email protected]). Complete chloroplast DNA was extracted from dried leaf specimens of C. scabrifolia using the CTAB extraction method (Doyle and Doyle Citation1987). A total of 3 G of raw data from the Illumina Hiseq Platform (Illumina, San Diego, CA) were sequenced. Afterward, the raw data were used to assemble the complete chloroplast genome using GetOrganelle software (Jin et al. Citation2020) with C. pinnatifida (NC_057086.1) as the reference. The complete cp genome of C. scabrifolia was a typical quadripartite structure (Figure S1 and ). The annotated results were modified using Geneious Prime (Kearse et al. Citation2012). The complete chloroplast genome of C. scabrifolia has been submitted to GenBank with the accession number OP021659. The OGDRAW program (https://chlorobox.mpimp-golm.mpg.de/index.html) was used to draw a detailed physical map of the C. scabrifolia chloroplast genome.
Figure 2. Gene map of the Crataegus scabrifolia plastid genome. Genes drawn inside the circle are transcribed clockwise, and those outside are transcribed counterclockwise. Genes belonging to different functional groups are color coded. The darker gray in the inner circle corresponds to DNA G + C content, while the lighter gray corresponds to A + T content. LSC: large single-copy; SSC: small single-copy; IR: inverted repeat.
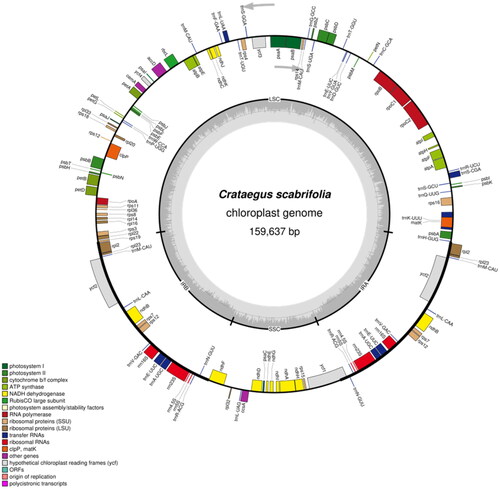
Simple sequence repeat analysis
Simple sequence repeats (SSRs) of C. scabrifolia were identified and localized using online MISA software (Beier et al. Citation2017). The repeat numbers of mononucleotide, dinucleotide, trinucleotide, tetranucleotide, pentanucleotide, and hexanucleotide were set to 10, 5, 4, 3, and 3, respectively. Identification of interspersed nuclear elements (INEs) included forward repeats, reverse repeats, palindromic repeats, and complementary repeats using the online Repter software (Kurtz et al. Citation2001). The maximum number of repeats was set to 50 and the minimum repeat size to 8 bp.
Phylogenetic analysis
A phylogenetic tree was reconstructed based on the chloroplast genome of C. scabrifolia and 21 species of Rosaceae, with Albizia julibrissin (NC_058305.1) and Cassia fistula (ON099431.1) as the outgroup. The MAFFT software was used for multiple alignments between the chloroplast genome of these 24 plants (scoring matrix = 200; PAM k = 2; gap open penalty = 1.53; offset value = 0.123) (Katoh and Standley Citation2013); the differences between the sequences were tested. Subsequently, the alignment results were checked using MEGA11 software, and the file was output in *.NET format. Next, an ML phylogeny tree was constructed using RAxML ver. 8.0.0. The parameters were set as bootstrap = 1000 and m = GTR + GAMMA (Stamatakis Citation2014). The maximum-likelihood phylogenetic tree was visualized using Fig Tree 1.4.3 software (http://tree.bio.ed.ac.uk/software/figtree/).
Results and discussion
Structural characteristics
The complete chloroplast genome of C. scabrifolia is 159,637 bp in length with a typical double-stranded circular tetrad structure, containing a small single-copy (SSC) region with a length of 19,139 bp, a large single-copy (LSC) region with a length of 87,730 bp, and a pair of inverted repeat (IR) regions with a length of 26,384 bp (). The contents of A, T, C, and G were 31.26%, 32.09%, 18.69%, and 17.96%, respectively. The overall GC content was 36.65% (LSC, 34.39%; SSC, 30.56%; IR, 42.64%). In total, 127 unique genes were annotated, including 83 protein-coding genes (PCGs), 8 ribosomal RNA genes (rRNAs), and 36 transfer RNA genes (tRNAs). Of the 127 genes encoded, there were 14 dual-copy genes, including 2 ribosome macro unit genes (rpl2, rpl23), 1 ribosome subunit gene (rps7), 1 NADH dehydrogenase gene (ndhB), 4 rRNA genes (rrn5, rrn4.5, rrn23, rrn16), 5 tRNA genes (trnV-GAC, trnR-ACG, trnN-GUU, trnL-CAA, trnA-UGC), and 1 unknown functional gene (ycf2). A total of 14 genes (atpF, ndhA, ndhB, petB, petD, rps16, rpl2, rpl22, rpoC1, trnA-UGC, trnE-UUC, trnK-UUU, trnL-UAA, trnS-CGA) contain one intron, and three genes (ycf3, rps12, clpP) contain two introns. The GC content in the IR region was much higher than in the LSC and SSC regions, and the different distribution of GC content was a typical feature of angiosperms (Kim and Lee Citation2004; Terakami et al. Citation2012). The main reason behind this phenomenon is that there are four GC-rich rRNA genes in the IR region (Qian et al. Citation2013).
Sequence repeats analysis
A total of 98 SSRs were discovered by the online software MISA-web (Beier et al. Citation2017), with the numbers of mono-, di-, tri-, tetra-, pentanucleotides, and hexanucleotide SSRs being 72, 20, 1, 4, 1, and 0, respectively. According to the frequency of classified repeat types (considering sequence complementarity), mononucleotide repeats have A/T and C/G, 67 and 5, respectively; dinucleotide repeats have AG/CT and AT/AT, 1 and 19, respectively; trinucleotide repeats have only one AAT/ATT; tetranucleotide repeats have four AAAT/ATTT; and pentanucleotide repeats have only one AATCC/ATTGG. The single nucleotides in the C. scabrifolia chloroplast genome are biased toward A/T repeats. They have the largest number, which is in line with the results of the largest number of single nucleotides A and T in previous studies (Kuang et al. Citation2011; Yang et al. Citation2021;). A total of 50 repeats were identified in the chloroplast genome of C. scabrifolia, including 26 forward repeats, 7 reverse repeats, 14 palindromic repeats, and 3 complementary repeats. There are 36 repeats with a length of 20–30 bp, accounting for the majority (72%).
Phylogenetic analyses
Based on the phylogenetic analysis, all species of Crataegus have formed a monophyletic clade. In addition, the analysis results showed that all Crataegus plants are divided into two clades, among which Crataegus mollis formed a single clade, and the other Crataegus plants formed a compound clade. C. hupehensis is closely related to C. scabrifolia, with a bootstrapped support rate of 86% (). The present sample strongly supported the taxonomic result. The fruits of the two species are red and the inflorescences are glabrous. The phylogenetic analysis results are also consistent with the classification of Flora Reipublicae Popularis Sinicae (Wu Citation1974). The complete chloroplast genome of C. scabrifolia can provide reference value and a theoretical basis for further taxonomic research, genetic engineering, and comparative genomics.
Figure 3. The best maximum-likelihood (ML) phylogenetic tree reconstructed by RAxML ver. 8.0.0 based on complete chloroplast genome sequences from 22 species of Rosaceae, including 9 Crataegus species, Albizia julibrissin, and Cassia fistula as outgroup. The numbers on branches are bootstrap support values from 1,000 replicates. The following sequences were used: Pyrus turcomanica NC 061559.1 (Yang et al. Citation2020); Pyrus georgica NC_061558.1 (Wang et al. Citation2016); Pyrus demetrii NC 061933.1 (Hong et al. Citation2021); Pyrus salicifolia NC 061935.1 (Katayama and Uematsu Citation2005); Pyrus hopeiensis MF521826.1 (Li et al. Citation2021); Pyrus ussuriensis NC 041461.1 (Boby et al. Citation2021); Sorbus insignis NC 051947.1 (Tan et al. Citation2020); Sorbus koehneana NC 063569.1(Li et al. Citation2021); Sorbus rehderiana OK012001.1(Ma et al. Citation2007); Malus ombrophila MW115598.1 (Lu et al. Citation2022); Malus transitoria MK098838.1 (Lu et al. Citation2022); Aronia arbutifolia NC 045391.1 (Taheri et al. Citation2013); Aronia melanocarpa MT527725.1 (Jurikova et al. Citation2017); Crataegus mollis NC 062346.1(Cha et al. Citation2018); Crataegus laevigata NC 062347.1 (Nguyen et al. Citation2021); Crataegus rhipidophylla NC 062345.1 (Żurek et al. Citation2021); Crataegus cuneata NC 058896.1 (Cui et al. Citation2022); Crataegus pinnatifida var. major MW653326.1 (Pang et al. Citation2021); Crataegus pinnatifida MW653325.1 (Guo et al. Citation2021); Crataegus bretschneideri MW963339.1(Zheng et al. Citation2021); Crataegus hupehensis NC 054155.1 (Hu et al. Citation2021); Albizia julibrissin NC 058305.1 (Zhang et al. Citation2021); Cassia fistula ON099431.1 (Sharma et al. Citation2021).
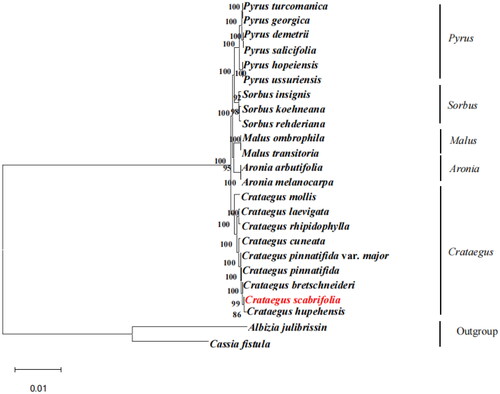
Conclusions
The C. scabrifolia chloroplast genome was obtained using the Illumina HiSeq sequencing platform. The repeated sequences in this experiment may provide more specific and effective molecular markers for the classification, phylogenetic evolution, development, and gene map construction of Crataegus resources.
Authors contributions
X. Y. conceived the study and collected the molecular materials; T. P. drafted the manuscript and analyzed the experimental data; Z. N. Z. analyzed the experimental data. All authors provided comments and final approval.
Supplemental Material
Download MS Word (249.7 KB)Disclosure statement
No potential conflict of interest was reported by the authors.
Data availability statement
The data that newly obtained at this study are available in the NCBI under accession number of OP021659 (https://www.ncbi.nlm.nih.gov/nuccore/OP021659). The associated BioProject, SRA, and Bio-Sample numbers are PRJNA860797, SRR20339770, and SAMN29862852, respectively.
Additional information
Funding
References
- Beier S, Thiel T, Münch T, Scholz U, Mascher M. 2017. MISA-web: a web server for microsatellite prediction. Bioinformatics. 33(16):2583–2585.
- Boby N, Abbas MA, Lee EB, Im ZE, Hsu WH, Park SC. 2021. Protective effect of Pyrus ussuriensis Maxim. extract against ethanol-induced gastritis in rats. Antioxidants. 10(3):439.
- Cha DH, Powell THQ, Feder JL, Linn CE.. 2018. Identification of a new blend of host fruit volatiles from Red Downy hawthorn, Crataegus mollis, Attractive to Rhagoletis pomonella Flies from the Northeastern United States. J Chem Ecol. 44(7-8):671–680.
- Cui Y, Du K, Hou S, Yang R, Qi L, Li J, Chang Y. 2022. A comprehensive strategy integrating metabolomics with multiple chemometric for discovery of function related active markers for assessment of foodstuffs: a case of hawthorn (Crataegus cuneata) fruits. Food Chem. 383:132464.
- Dahmer S, Scott E. 2010. Health effects of hawthorn (complementary and alternative medicine). Am Fam Physician. 81(4):465–468.
- Doyle JJ, Doyle JL. 1987. A rapid DNA isolation procedure for small amounts of fresh leaf material. Photochem. Bull. 19:11–15.
- Guo C, Wang Y, Zhang S, Zhang X, Du Z, Li M, Ding K. 2021. Crataegus pinnatifida polysaccharide alleviates colitis via modulation of gut microbiota and SCFAs metabolism. Int J Biol Macromol. 181:357–368.
- Hong SY, Lansky E, Kang SS, Yang M. 2021. A review of pears (Pyrus spp.), ancient functional food for modern times. BMC Complement Med Ther. 21(1):219.
- Hu G, Zheng S, Pan Q, Dong N. 2021. The complete chloroplast genome of Crataegus hupehensis Sarg. (Rosaceae), a medicinal and edible plant in China. Mitochondrial DNA Part B 6(2):315–317.
- Jin JJ, Yu WB, Yang JB, Song Y, DePamphilis CW, Yi TS, Li DZ. 2020. GetOrganelle: a fast and versatile toolkit for accurate de novo assembly of organelle genomes. Genome Biol. 21(1):1–31.
- Jurikova T, Mlcek J, Skrovankova S, Sumczynski D, Sochor J, Hlavacova I, Snopek L, Orsavova J. 2017. Fruits of Black Chokeberry Aronia melanocarpa in the prevention of chronic diseases. Molecules. 22(6):944.
- Jurikova T, Sochor J, Rop O, Mlcek J, Balla S, Szekeres L, Adam V, Kizek R. 2012. Polyphenolic profile and biological activity of Chinese hawthorn (Crataegus pinnatifida BUNGE) fruits. Molecules. 17(12):14490–14509.
- Katayama H, Uematsu C. 2005. Structural analysis of chloroplast DNA in Prunus (Rosaceae): evolution, genetic diversity and unequal mutations. Theor Appl Genet. 111(7):1430–1439.
- Katoh K, Standley DM. 2013. MAFFT multiple sequence alignment software version 7: improvements in performance and usability. Mol Biol Evol. 30(4):772–780.
- Kearse M, Moir R, Wilson A, Stones-Havas S, Cheung M, Sturrock S, Buxton S, Cooper A, Markowitz S, Duran C, et al. 2012. Geneious Basic: an integrated and extendable desktop software platform for the organization and analysis of sequence data. Bioinformatics. 28(12):1647–1649.
- Kim E, Jang E, Lee JH. 2022. Potential roles and key mechanisms of hawthorn extract against various liver diseases. Nutrients. 14(4):867.
- Kim KJ, Lee HL. 2004. Complete chloroplast genome sequences from Korean ginseng (Panax schinseng Nees) and comparative analysis of sequence evolution among 17 vascular plants. DNA Res. 11(4):247–261.
- Kuang D-Y, Wu H, Wang Y-L, Gao L-M, Zhang S-Z, Lu L. 2011. Complete chloroplast genome sequence of Magnolia kwangsiensis (Magnoliaceae): implication for DNA barcoding and population genetics. Genome. 54(8):663–673.
- Kurtz S, Choudhuri JV, Ohlebusch E, Schleiermacher C, Stoye J, Giegerich R. 2001. REPuter: the manifold applications of repeat analysis on a genomic scale. Nucleic Acids Res. 29(22):4633–4642.
- Li Y, Zhang J, Wang S, Zhang Y, Yang M. 2021. The distribution and origins of Pyrus hopeiensis – "Wild plant with tiny population”. Front Plant Sci. 12:668796.
- Li J, Zhu K, Wang Q, Chen X. 2021. Genome size variation and karyotype diversity in eight taxa of Sorbus sensustricto (Rosaceae) from China. Comp Cytogenet. 15(2):137–148.
- Lu Y, Wang X, Wu Y, Wang Z, Zhou N, Li J, Shang X, Lin P. 2022. Chemical characterization of the antioxidant and α-glucosidase inhibitory active fraction of Malus transitoria leaves. Food Chem. 386:132863.
- Ma JM, Liu SR, Shi ZM, Zhang YD, Chen BY. 2007. Quantitative analysis of different restoration stages during natural succession processes of subalpine dark brown coniferous forests in western Sichuan, China. Ying Yong Sheng Tai Xue Bao. 18(8):1695–1701.
- Martinelli F, Perrone A, Yousefi S, Papini A, Castiglione S, Guarino F, Cicatelli A, Aelaei M, Arad N, Gholami M, et al. 2021. Botanical, phytochemical, anti-microbial and pharmaceutical characteristics of hawthorn (Crataegus monogyna Jacq.), Rosaceae. Molecules. 26(23):7266.
- Nguyen QTN, Fang M, Zhang M, Do NQ, Kim M, Zheng SD, Hwang E, Yi TH. 2021. Crataegus laevigata suppresses LPS-induced oxidative stress during inflammatory response in human keratinocytes by regulating the MAPKs/AP-1, NFκB, and NFAT signaling pathways. Molecules. 26(4):869.
- Pang X, Wang M, Wang SY, Zhang J, Du YP, Zhao Y, Zheng XH, Ma BP. 2021. Phenolic compounds from the leaves of Crataegus pinnatifida Bge. var. major N.E.Br. And their lipid-lowering effects. Bioorg Med Chem Lett. 47:128211.
- Qian J, Song J, Gao H, Zhu Y, Xu J, Pang X, Yao H, Sun C, Li X, Li C, et al. 2013. The complete chloroplast genome sequence of the medicinal plant Salvia miltiorrhiza. PLOS One. 8(2):e57607.
- Sharma R, Jindal R, Faggio C. 2021. Cassia fistula ameliorates chronic toxicity of cypermethrin in Catla catla. Comp Biochem Physiol C Toxicol Pharmacol. 248:109113.
- Stamatakis A. 2014. RAxML version 8: a tool for phylogenetic analysis and post-analysis of large phylogenies. Bioinformatics. 30(9):1312–1313.
- Taheri R, Connolly BA, Brand MH, Bolling BW. 2013. Underutilized chokeberry (Aronia melanocarpa, Aronia arbutifolia, Aronia prunifolia) accessions are rich sources of anthocyanins, flavonoids, hydroxycinnamic acids, and proanthocyanidins. J Agric Food Chem. 61(36):8581–8588.
- Tan J, Wang Y, Wu W, Li Y, Zhao W, Liao W, Fan Q, Liao B. 2020. The complete genome sequence of Sorbus insignis (Rosaceae: Amygdaloideae), an epiphytic shrub in this genus. Mitochondrial DNA Part B 5(3):3239–3240.
- Terakami S, Matsumura Y, Kurita K, Kanamori H, Katayose Y, Yamamoto T, Katayama H.,. 2012. Complete sequence of the chloroplast genome from pear (Pyrus pyrifolia): genome structure and comparative analysis. Tree Genet Genomes. 8(4):841–854.
- Wang J, Xiong X, Feng B. 2013. Effect of crataegus usage in cardiovascular disease prevention: an evidence-based approach. Evid Based Complement Alternat Med. 2013:149363.
- Wang GM, Yin H, Qiao X, Tan X, Gu C, Wang BH, Cheng R, Wang YZ, Zhang SL. 2016. F-box genes: genome-wide expansion, evolution and their contribution to pollen growth in pear (Pyrus bretschneideri). Plant Sci. 253:164–175.
- Wu ZY. 1974. Crataegus scabrifolia. Flora Reipublicae Popularis Sinicae. Beijing : science Press. 36(1):p. 197.
- Yang H, Li Y, Jin Y, Kan L, Shen C, Malladi A, Nambeesan S, Xu Y, Dong C. 2020. Transcriptome analysis of Pyrus betulaefolia seedling root responses to short-term potassium deficiency. IJMS. 21(22):8857.
- Yang H, Wang L, Chen H, Jiang M, Wu W, Liu S, Wang J, Liu C. 2021. Phylogenetic analysis and development of molecular markers for five medicinal Alpinia species based on complete plastome sequences. BMC Plant Biol. 21(1):1–16.
- Zhang J, Huang H, Qu C, Meng X, Meng F, Yao X, Wu J, Guo X, Han B, Xing S. 2021. Comprehensive analysis of chloroplast genome of Albizia julibrissin Durazz. (Leguminosae sp.). Planta. 255(1):26.
- Zheng S, Song H, Dong N. 2021. The complete chloroplast genome of Crataegus bretschneideri Schneid. (Rosaceae). Mitochondrial DNA Part B. 6(12):3322–3324.
- Zhu QJ, Lang LJ, Jiang B, Shen Y, Wang Y, Xiao CJ. 2021. Chemical constituents of the fruits of wild Crataegus scabrifolia. J Dali Univ. 6(04):7–9.
- Żurek N, Karatsai O, Rędowicz MJ, Kapusta IT. 2021. Polyphenolic compounds of Crataegus berry, leaf, and flower extracts affect viability and invasive potential of human glioblastoma cells. Molecules. 26(9):2656.