Abstract
This is the first report on the complete mitochondrial genome (mitogenome) of South African Thyrsites atun (Euphrasén, 1791) and its phylogenetic placement within the Gempylidae family. The complete mitogenome of snoek is 16,494 bp in length and comprises 2 rRNAs, 13 protein-coding genes, 22 tRNAs, and one control region. Gene order is similar to that found in gempylids and other marine fishes. Reconstruction of Gempylidae phylogeny implies that the mitogenomes of snoek, black snoek Thyrsitoides marleyi, and snake mackerel, Gempylus serpens are closely related in evolutionary terms.
1. Introduction
Fishes of the family Gempylidae are fast-swimming oceanic predators often found in the deep waters of tropical and subtropical seas (Matsubara and Iwai Citation1952; Nakamura Citation1993; Li et al. Citation2020; Hüne et al. Citation2021). The family consists of 24 species described in 16 genera (Nelson Citation2006; Li et al. Citation2020). A number of these species are economically important (Crawford and De Villiers Citation1985; Kailola et al. Citation1993; Griffiths Citation2002, Citation2003). Although the fishes of the Gempylidae have long been of interest to ichthyologists and considerable literature on this family has accumulated, the lack of consensus concerning phylogenetic relationships within this family has remained problematic (Matsubara and Iwai Citation1952; Johnson Citation1986; Carpenter et al. Citation1995; Li et al. Citation2020). In an effort to address this issue, we present here for the first time a full mitochondrial genome of the South African T. atun (GenBank Accession Number: OP133162). In a parallel effort, our research group assembled an additional four T. atun mitogenomes from the Amsterdam and Saint-Paul Islands, the Inaccessible Islands, Chile, and New Zealand, these are not described in the current paper. In teleosts, the use of mitogenomic data to resolve complex evolutionary relationships has been explicitly shown (Lavoué et al. Citation2005; Miya and Nishida Citation2015; Friedman et al. Citation2019). Thyrsites atun () is a medium-sized, pelagic predator distributed widely in the southern hemisphere where it supports both moderate and substantial fisheries (Griffiths Citation2003). In South Africa, T. atun (Cape snoek) is a commercially important species targeted by artisanal fisheries and commercial trawlers and constitutes a substantial portion of by-catch in teleost fisheries (Hutchings and Lamberth Citation2002).
Figure 1. Thyrsites atun species reference image. Snoek has an elongated and strongly compressed body, it is dark blue with a paler belly. The key distinguishing feature of snoek is the single lateral line running close to the upper contour of the body below most of the first dorsal-fin base then abruptly curving ventrally. Photo by Brian Gratwicke distributed under license Creative Commons Attribution-Share Alike v2.0 Generic.
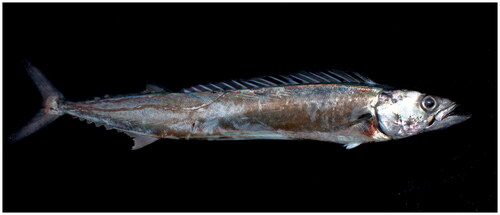
2. Materials
The sample whose mitogenome we present was provided by the Department of Forestry, Fisheries, and the Environment (DFFE) of South Africa, Cape Town. The fin clip was collected from a snoek individual caught in St Helena Bay, South Africa (32°44’29.5” S 18°01’12.2” E) in November 2010. The fin-clip sample (stored in 99% ethanol) and DNA are kept at Stellenbosch University’s Genetics Department in South Africa under sample ID: SASN032 (http://www.sun.ac.za/english/faculty/agri/genetics, Aletta Bester-van der Merwe, [email protected]).
3. Methods
Total genomic DNA was extracted from the tissue using the cetyltrimethylammonium bromide (CTAB) extraction protocol (Sambrook and Russell Citation2001). Whole-genome sequencing was performed on the Ion Torrent S5TM platform (ThermoFisher Scientific, Waltham, MA, USA) using 400 base-pair (bp) chemistry following the manufacturer’s protocol. An identical approach was used for all five samples. The New Zealand sample with 4,019,478 reads of 374 bp average read length post-quality filtering was the best candidate for a draft genome assembly and was thus assembled first and used as the reference genome for the subsequent samples. These reads were mapped to an incomplete mitogenome of G. serpens (AP012502) which is the closest relative of snoek according to a phylogeny published by Miya et al. (Citation2013), using Geneious Prime v2020.2.5 (Biomatters Ltd., Auckland, New Zealand). The result was an incomplete draft mitogenome missing the control region. Using the SPAdes assembler v3.15.5 (Prjibelski et al. Citation2020) we constructed the snoek mitogenome using the de novo technique. When the de novo technique failed to construct a complete mitogenome, we took the resultant contigs and mapped them against snoek’s partial mitogenome. The contigs extended to the presumed control region resulting in a full mitochondrial genome. The newly constructed T. atun mitogenome, OP598802, was then utilized as a reference sequence for the remaining T. atun genome assemblies including the assembly of South African snoek (OP133162). Read mapping and assembly were carried out in Geneious Prime v2020.2.5 software (https://www.geneious.com) utilizing the Geneious mapper with medium/low sensitivity and fine-tuning up to five iterations, followed by manual curation.
Annotations were carried out using MitoAnnotator (Iwasaki et al. Citation2013) and confirmed with the MITOS Web server (Bernt et al. Citation2013). To investigate the placement of T. atun within the Gempylidae family, we performed Bayesian inference in MrBayes 3.2.7 (Ronquist et al. Citation2012). Thirteen protein-coding gene sequences excluding their stop codons were aligned independently and concatenated in the order in which they appear in the mitogenome producing an alignment of 11,400 bp. The nucleotide alignment was then gene-partitioned and used for tree inference. While it is common practice to select a substitution model for Bayesian phylogenetic inference using a priori model selection processes, we used the model averaging strategy instead. This strategy allows the user to choose from among all 203 alternative time-reversible rate matrices based on their posterior probability, avoiding uncertainty about the appropriate substitution model, which is an issue inherent in likelihood-based techniques (Ronquist et al. Citation2012; Bouckaert and Drummond Citation2017). The analysis was run for 10,000,000 generations with a sampling frequency of 2,000 and 25% of starting trees was discarded as burn-in. Species included in the analysis are reported in .
Table 1. List of mitochondrial sequences used in phylogenetic reconstruction of the family Gempylidae (Scombriformes) to investigate the placement of Thyrsites atun among 12 other species of gempylids. Trichiurus lepturus and Benthodesmus tenuis (Scombriformes: Trichiuridae) were used as outgroups (marked by an asterisk).
4. Results
The complete and circular mitogenome of South African T. atun is 16,494 bp in size and comprises 13 protein-coding regions, 22 tRNA genes, 12S, and 16S ribosomal RNAs, and a non-coding D-loop region. The gene arrangement and direction are typical of fishes. The ND6 subunit gene and eight tRNAs (trnQ(cca), trnA(gca), trnN(aac), trnC(tgc), trnY(tac), trnS(tca), trnE(gaa), and trnP(cca)) are encoded on the light-strand, while the other 28 genes are encoded on the heavy-strand (). The 13 protein-coding genes used the conventional ATN start codon except for COX1 and CYTB which used GTG. The ATP6, ATP8, COX1, ND1, ND4L, and ND5 genes used the TAA stop codon. The AGA stop codon was exclusive to ND4 and ATC to ND6, while an incomplete stop codon TA- was found for ND2 and COX3; and T– for COX2, ND3, and CYTB. The 13 protein-coding genes are 11,479 bp in total, and the 12S and 16S rRNAs are 959 and 1,701 bp, respectively. The 22 tRNA genes range from 65 bp (trnF(ttc)) to 75 bp (trnK(aaa)) in size. The mitogenome has an A + T (54.1%) bias, and nucleotide composition of A, 27.2%, T, 27%, G, 17.4%, and C, 28.5%.
Figure 2. The annotated mitochondrial genome of South African Thyrsites atun. The complete and circular mitochondrial genome is 16,494 base pairs long, it consists of 13 protein-coding genes, 22 transfer RNA genes, 2 ribosomal RNA genes, and a control region (D-loop). Arrows indicate transcription directions.
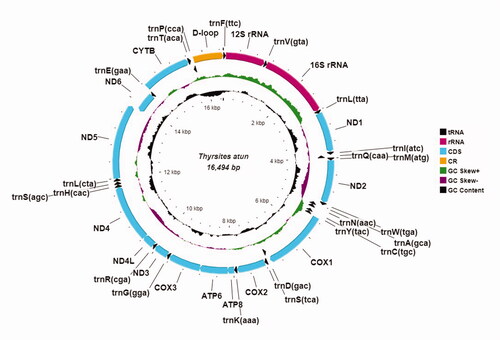
5. Discussion and conclusion
Although this is not well supported and could be the result of unrepresentative sampling, our result suggests the mitogenome of Thyrsites atun is closely related to that of T. marleyi and G. serpens (). This is in disagreement with previously published morphology-based phylogenies. Despite having a striking resemblance, Thyrsites atun, also known as snoek, and Thyrsitoides marleyi, commonly known as black snoek, are not closely related according to morphology-based phylogenies of Russo (Citation1983) and Beckett et al. (Citation2018). On the other hand, Gempylus and Thyrsites are the only Gempylidae species to share a carangiform swimming mode and a high number of dorsal and anal finlets, making them evolutionary close relatives (Monsch Citation2000).
Figure 3. Phylogenetic tree depicting placement of Thyrsites atun [OP133162] within the Gempylidae family derived from a Bayesian analysis of 13 protein-coding genes. Members of Trichiuridae; Trichiurus lepturus [MK333401] and Benthodesmus tenuis [AP012522] are included as outgroups. Nodal support is indicated by Bayesian Posterior Probabilities (in red are BPP <0.95, and in black are BPP≥ 0.95).
![Figure 3. Phylogenetic tree depicting placement of Thyrsites atun [OP133162] within the Gempylidae family derived from a Bayesian analysis of 13 protein-coding genes. Members of Trichiuridae; Trichiurus lepturus [MK333401] and Benthodesmus tenuis [AP012522] are included as outgroups. Nodal support is indicated by Bayesian Posterior Probabilities (in red are BPP <0.95, and in black are BPP≥ 0.95).](/cms/asset/dd0f2fdd-74e2-4ecb-bb35-606cdf95e6b0/tmdn_a_2179353_f0003_c.jpg)
Ethical approval
The study was exempted from ethical approval or permissions. The sample used in the current study was donated by the Department of Forestry, Fisheries and the Environment of Cape Town, South Africa.
Authors’ contributions
The authors confirm their contribution to the paper as follows; study conception and design: R.R.W., A.B.M.; analysis and interpretation of results: S.M.; draft manuscript preparation: S.M. All authors reviewed the results and approved the final version of the manuscript.
Acknowledgments
The authors thank the Department of Forestry, Fisheries, and the Environment for providing the tissue sample. The authors thank all staff at the Central Analytical Facility, Stellenbosch University who assisted in the sequencing of this sample.
Disclosure statement
The authors report no conflict of interest.
Data availability statement
The genome sequence data that support the findings of this study are openly available in GenBank of NCBI at https://www.ncbi.nlm.nih.gov/ under accession no. OP133162. The associated BioProject, SRA, and Bio-Sample numbers are PRJNA878677, SRP396379, and SAMN30747289, respectively.
Additional information
Funding
References
- Beckett HT, Giles S, Johanson Z, Friedman M. 2018. Morphology and phylogenetic relationships of fossil snake mackerels and cutlassfishes (Trichiuroidea) from the Eocene (Ypresian) London clay formation. Pap Palaeontol. 4(4):577–603.
- Bernt M, Donath A, Jühling F, Externbrink F, Florentz C, Fritzsch G, Pütz J, Middendorf M, Stadler PF. 2013. MITOS: improved de novo metazoan mitochondrial genome annotation. Mol Phylogenet Evol. 69(2):313–319.
- Bouckaert RR, Drummond AJ. 2017. bModelTest: Bayesian phylogenetic site model averaging and model comparison. BMC Evol Biol. 17(1):1–11.
- Bustamante C, Ovenden JR. 2016. The complete validated mitochondrial genome of the silver gemfish Rexea solandri (Cuvier, 1832) (Perciformes: Gempylidae). Mitochondrial DNA Part A DNA Mapp Seq Anal. 27(1):405–406.
- Carpenter K, Collette B, Russo J. 1995. Unstable and stable classifications of Scombroid fishes. Bull Mar Sci. 56:379–405.
- Crawford RJM, De Villiers G. 1985. Snoek and their prey – interrelationships in the Benguela upwelling system. S Afr J Sci. 81:91–97.
- Friedman M, Feilich KL, Beckett HT, Alfaro ME, Faircloth BC, Černý D, Miya M, Near TJ, Harrington RC. 2019. A phylogenomic framework for pelagiarian fishes (Acanthomorpha: Percomorpha) highlights mosaic radiation in the open ocean. Proceedings of the Royal Society B: Biological Sciences 286: p. 1–59.
- Griffiths MH. 2002. Life history of South African snoek, Thyrsites atun (Pisces: Gempylidae): a pelagic predator of the Benguela ecosystem. Fish Bull. 100:690–710.
- Griffiths MH. 2003. Stock structure of snoek Thyrsites atun in the Benguela: a new hypothesis. Afr J Mar Sci. 25(1):383–386.
- Hüne M, Oyarzún PA, Reyes P, Rivera G, Montecinos M. 2021. Phylogeographic analysis of Thyrsites atun (Perciformes: Gempylidae) reveals connectivity between fish from South Africa and Chile. Mar Biol Res. 17(4):401–413.
- Hutchings K, Lamberth SJ. 2002. Bycatch in the gillnet and beach-seine fisheries in the Western Cape, South Africa, with implications for management. S Afr J Mar Sci. 7615:227–241.
- Iwasaki W, Fukunaga T, Isagozawa R, Yamada K, Maeda Y, Satoh TP, Sado T, Mabuchi K, Takeshima H, Miya M, et al. 2013. Mitofish and mitoannotator: a mitochondrial genome database of fish with an accurate and automatic annotation pipeline. Mol Biol Evol. 30(11):2531–2540.
- Johnson GD. 1986. Scombroid phylogeny: an alternative hypothesis. Bull Mar Sci. 39:1–41.
- Kailola PJ, Williams MJ, Stewart PC, Reichelt RE, McNee A, Grieve C. 1993. Australian fisheries resources. Bureau of Resources Sciences, Department of Primary Industries and Energy and the Fisheries Research and Development Corporation, Canberra, Australia. p. 341–343.
- Lavoué S, Miya M, Inoue JG, Saitoh K, Ishiguro NB, Nishida M. 2005. Molecular systematics of the gonorynchiform fishes (Teleostei) based on whole mitogenome sequences: implications for higher-level relationships within the Otocephala. Mol Phylogenet Evol. 37(1):165–177.
- Li Z, Qi J, Ran Q, Xu Y, Yu H, Xu P, Zhang J. 2020. The complete mitochondrial genome sequence of snake mackerels Paradiplospinus antarcticus (Scombroidei: Gempylidae). Mitochondrial DNA Part B: Resour. 5(3):2377–2378.
- Matsubara K, Iwai T. 1952. Studies on some Japanese fishes of the family Gempylidae. Pac Sci. 6:193–212.
- Miya M, Nishida M. 2015. The mitogenomic contributions to molecular phylogenetics and evolution of fishes: a 15-year retrospect. Ichthyol Res. 62(1):29–71.
- Miya M, Friedman M, Satoh TP, Takeshima H, Sado T, Iwasaki W, Yamanoue Y, Nakatani M, Mabuchi K, Inoue JG, et al. 2013. Evolutionary origin of the Scombridae (Tunas and Mackerels): Members of a Paleogene adaptive radiation with 14 other pelagic fish families. PLOS One. 8(9):e73535.
- Monsch KA. 2000. The phylogeny of the Scombroid fishes. Unpublished Ph.D. dissertation, University of Bristol, United Kingdom.
- Mukundan LP, Sukumaran S, Sebastian W, Gopalakrishnan A. 2020. Characterization of the whole mitogenome of Largehead hairtail Trichiurus lepturus (Trichiuridae): insights into special characteristics. Biochem Genet. 58(3):430–451.
- Nakamura I. 1993. FAO species catalog vol. 15. Snake mackerels and cutlassfishes of the world (families Gempylidae and Trichiuridae). FAO Fish Synop. 125:30–32.
- Nelson JS. 2006. Fishes of the world.Hoboken, New Jersey: John Wiley & Sons, Inc.; 431.
- Prjibelski A, Antipov D, Meleshko D, Lapidus A, Korobeynikov A. 2020. Using SPAdes de novo assembler. Curr Protocn Bioinformatics. 70(1):1–29.
- Ronquist F, Teslenko M, Van Der Mark P, Ayres DL, Darling A, Höhna S, Larget B, Liu L, Suchard MA, Huelsenbeck JP. 2012. Mrbayes 3.2: efficient bayesian phylogenetic inference and model choice across a large model space. Syst Biol. 61(3):539–542.
- Russo JL. 1983. [Interrelationships of the gempylid fishes (Teleostei, Scombroidei)]. [Unpubl Ph.D. Dissertation]. Washington, D.C: George Washington Univ.
- Sambrook J, Russell D. 2001. Molecular cloning: a laboratory manual 3rd edition. Cold Spring Harbor Laboratory Press, Cold Spring Harbor, New York.