Abstract
Staurogyne concinnula (Hance) O. Kuntze (Acanthaceae) is an important ornamental herb mainly distributed in the southern region of China, including Fujian, Guangdong, Hainan, and Taiwan provinces. However, the complete chloroplast genome of S. concinnula, which could serve as a genetic resource for studies on its taxonomy and evolution, is poorly studied at present. In this study, we reported the complete chloroplast genome of S. concinnula that was assembled using high-throughput sequencing data. The chloroplast genome was 153,783 bp long, with a typical quadripartite structure containing a small single-copy region (SSC; 17,855 bp), a large single-copy region (LSC; 84,636 bp) and a pair of inverted repeats (IRs; each 25,646 bp). The overall GC content of the chloroplast genome was 38.04%. A total of 86 protein-coding genes (PCGs), 8 rRNA genes, and 37 tRNA genes were predicted. Phylogenetic analysis based on the combined sequences of 86 PCGs with the other 16 closely related species of Acanthaceae indicated that S. concinnula is closely related to Avicennia marina. The genomic data and finding from the phylogenetic studies of S. concinnula could provide useful information and give light to in-depth studies on the evolution pattern of the understudied species, as well as Staurogyne.
Acanthaceae is a large family with more than 250 genera and is consisted of over 4000 different species. Members of Acanthaceae are widely distributed in tropical and subtropical regions, of which most of them prefer the warm and humid climate. Staurogyne Wall is one of the largest genera in Acanthaceae, accounting for approximately 80 species and most of them are herbaceous plants; while 14 of them were recorded in China (Flora of China Citation2002a). Staurogyne concinnula (Hance) O. Kuntze, 1891 is an important ornamental herb that is widely distributed in Fujian, Guangdong, Hainan, and Taiwan provinces of China. It is usually planted as landscape plants and green belts for rivers owing to their beautiful red-petaled flowers, which usually bloom during spring (; Flora of China Citation2002b). Staurogyne concinnula has very short stems covered by long and soft hair; while the back of its leaf appears pale in color.
Figure 1. Staurogyne concinnula growing in its natural environment at Huaiji County, Zhaoqing City (23.91°N, 112.18°E). (a) growing environment; (b) morphological characteristics at flowering time. These two photos were taken and edited by DC at the sampling site.
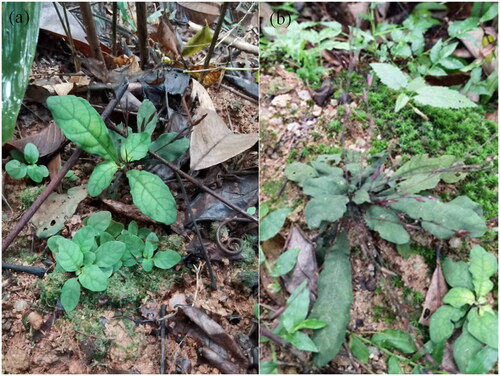
To date, studies on the phylogenetic relationship of S. concinnula are only limited to analysis based on morphology traits, mainly on the morphological characteristics of the pollen and leaf epidermal (Robert and Scotland Citation1992) as well as short gene sequences (McDade et al. Citation2012). However, the relationship within Staurogyne remains unresolved; the use of the combined chloroplast dataset trnS-trnG and ndhF-rpl32-trnL as well as the nuclear ribosomal internal transcribed spacer (ITS) region were not able to provide good resolution among the species examined (McDade et al. Citation2012). Genomic information on this species is generally lacking. Despite being an ornamental plant with great ecological value, the understudied species has been receiving less attention from researchers and ecologists. Therefore, to generate an amount of genetic data that could aid in the expansion of the genomic information as well as the genetic identity of this valuable herb species, in this study, we assembled and characterized the complete chloroplast genome of S. concinnula via next-generation sequencing technique. Phylogenetic inference was conducted to reveal the molecular placement of S. concinnula at the chloroplast genome level.
Fresh leaves were collected from one individual of S. concinnula at Huaiji County, Zhaoqing City in the middle of Guangdong Province (23.91°N, 112.18°E). The voucher specimen was deposited in the Herbarium of Guangdong Academy of Agricultural Sciences under the accession number HGAAS-1254 (Contact person: Shike Cai; e-mail: [email protected]). Total genomic DNA was extracted using a modified CTAB method (Doyle and Doyle Citation1987) and the concentration of the DNA extract was quantified using Qubit 3.0 Fluorometer (Fisher Scientific, USA). A 350-bp insert-size library was constructed using VAHTS Universal DNA Library Prep Kit for Illumina V3 (Vazyme, Nanjing) using 1.0 μg total DNA and then was sequenced on an Illumina NovaSeq 6000 platform (Illumina, USA) following the manufacturer’s protocol via PE 150 mode. Approximately 5.0 GB of raw data was obtained, which was used as input data in the GetOrganelle v1.6.2e pipeline (Jin et al. Citation2020) for chloroplast genome assembly. The assembly was completed by using 10,100,000 effective reads with an average base coverage of 429×. The assembled chloroplast genome sequence was annotated using both CpGAVAS2 (Shi et al. Citation2019) and GeSeq v2.03 (Tillich et al. Citation2017), with the chloroplast genome of Avicennia marina (GenBank accession number: MT012822; Ruang-Areerate et al. Citation2022) as the reference. The annotations were manually checked for errors.
Its minimum depth of the assembled chloroplast genome was 91× while the average was 1607× (Supplementary Figure 1). The complete chloroplast genome of S. concinnula was 153,783 bp in length, with a typical quadripartite structure that had similar length and structure when compared to other closely related species of Acanthaceae (Huang et al. Citation2020; Kaewdaungdee et al. Citation2022). The inverted repeat (IR) regions were 25,646 bp long for each and were separated by the 84,636-bp large single-copy (LSC) as well as the 17,855-bp small single-copy (SSC) regions. The overall GC content of the chloroplast genome was 38.04% with 30.61% A, 18.70% G, 31.35% C and 19.34% G, respectively. Finally, a total of 131 genes were predicted, including 86 protein-coding genes (PCGs), 8 rRNA genes, and 37 tRNA genes (). The map of the complete chloroplast genome was drawn using the CPGView program (http://www.1kmpg.cn/cpgview, Liu et al. Citation2023). Furthermore, there are 11 genes that contain two exons and 3 genes contain three exons (Supplementary Figure 2), while rps12 gene was identified as the trans-splicing gene (Supplementary Figure 3).
Figure 2. Chloroplast genome map of Staurogyne concinnula. From the center outward, the first track shows the dispersed repeats. The dispersed repeats consist of direct (D) and Palindromic (P) repeats, connected with red and green arcs. The following circular shows the long tandem repeats by short blue bars. The third track shows microsatellite sequences as short bars with different colors. The small single-copy (SSC), inverted repeat (IRa and IRb), and large single-copy (LSC) regions are shown on the fourth circular. The GC content along the genome is plotted on the fifth circular. The base frequency at each site along the genome will be shown between the fourth and fifth tracks. The genes are shown on the sixth track. The outer and inner colored boxes present transcribed clockwise and counterclockwise genes, respectively.
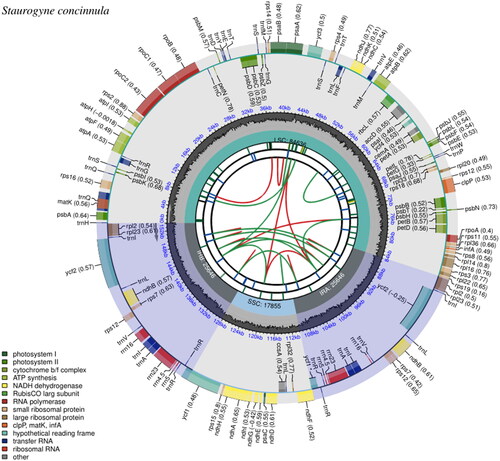
To infer the molecular phylogenetic position of S. concinnula in Acanthaceae, the chloroplast genome sequences of additional 16 species of Acanthaceae were included in the phylogenetic analysis. Four closely related species, including Scoparia dulcis (GenBank accession number: MZ958832; unpublished), Plantago atrata (GenBank accession number: MW877580; Mower et al. Citation2021), Neopicrorhiza scrophulariiflora (GenBank accession number: MK986819; Zhang et al. Citation2019), and Callitriche palustris (GenBank accession number: MW774642; unpublished) were included as outgroups. The combined 86 coding protein genes of chloroplast genome sequences were aligned using MAFFT v7.3 (Katoh and Standley Citation2013) and phylogenetic tree reconstruction was conducted based on two different methods – maximum likelihood (ML) and Bayesian inference (BI). For ML, the nucleotide substitution model GTRGAMMA was applied in RAxML v8.2.12 (Stamatakis Citation2006), coupled with 1,000 bootstrap replicates. For BI, the most optimum nucleotide substitution model determined using ModelTest-NG (Darriba et al. Citation2020) was GTR + I+G4. The parameters used in the MrBayes v3.2.7 (Ronquist et al. Citation2012) pipeline were set at 1000,000 generations, in which sampling was conducted every 100 cycles. As both trees showed similar topology, only the ML tree was displayed (). Overall, the phylogenetic relationship among the members of Acanthaceae was resolved; all the branch nodes were strongly supported (ML bootstrap value ≥75%, BI posterior probability ≥0.90). Based on the current circumscription, S. concinnula was closely related to Avicennia marina. This finding is congruent with the phylogenetic study reported by McDade et al. (Citation2012); as most of the chloroplast genome records from members of Nelsonioideae are not yet available publicly, Avicennia and Mendoncia would be the most immediate genera after Staurogynei. As the chloroplast genome sequence of S. concinnula reported in this study is the first among the species of the same genus, the finding of this study has provided insight into the phylogenetic placement of Staurogynei at the chloroplast genome level. As the chloroplast genome sequence is proposed to be useful in resolving the relationship among species in complicated genera (Li et al. Citation2018; Han et al. Citation2022), it may provide a greater resolution toward phylogenetic analysis of Staurogynei; thus, provides aid to the understanding of the evolution pattern and phylogenomics of Acanthaceae.
Figure 3. Phylogenetic tree combined with Maximum-likelihood and Bayes Inference trees based on 86 combined PCGs’s sequences. The number on each node indicates the ML bootstrap value (left) and BI posterior probability (right). The top scale bar represents the number of substitutions per site. The following 16 sequences in Acanthaceae has participated in phylogenetic analyses: Thunbergia erecta (MZ555773, Tong et al. Citation2022); Avicennia marina (MT012822, Li et al. Citation2020); four Dicliptera species (MK833947, MK830556, MK833946, MK848596, Huang et al. Citation2020); Peristrophe japonica (NC_056198, MW411448, Chen et al. Citation2021); Clinacanthus nutans (NC_042162, Li et al. Citation2019); Pseuderanthemum haikangense (NC_057986, Gao et al. Citation2020); two Echinacanthus species (MH045155, MH045157, unpublished); Barleria prionitis (MK548575, Alzahrani et al. Citation2020); Aphelandra knappiae (NC_041424, Huang et al. Citation2019); two Acanthus species (MW752129, Xu et al. Citation2021; MT240944, unpublished).
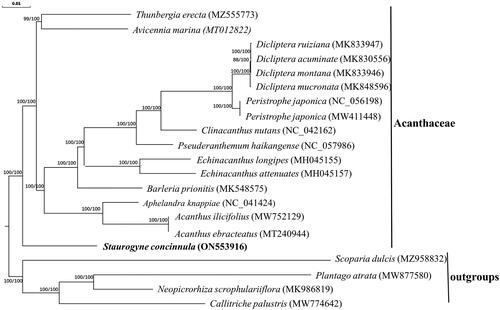
Authors contributions
Samples were collected by YM, DC & SC. YM & DC analyzed the sequenced data and drafted this paper. SC designed this work and accomplished the revision of this paper.
Ethics statement
This study was ethically approved and received permission for collecting the sample from Guangdong Academy of Agricultural Sciences.
Supplemental Material
Download JPEG Image (338.6 KB)Supplemental Material
Download JPEG Image (132.8 KB)Supplemental Material
Download TIFF Image (1.4 MB)Disclosure statement
No potential conflict of interest was reported by the author(s).
Data availability statement
The chloroplast genome sequence data in this study are openly available in GenBank of NCBI at https://www.ncbi.nlm.nih.gov/ with the accession number is ON553916. Raw sequencing data was deposited in the SRA database of NCBI under accession number SRR19345956. The associated “BioProject”, and “Bio-Sample” numbers are PRJNA841032, and SAMN28573330, respectively.
Additional information
Funding
References
- Alzahrani DA, Yaradua SS, Albokhari EJ, Abba A. 2020. Complete chloroplast genome sequence of Barleria prionitis, comparative chloroplast genomics and phylogenetic relationships among Acanthoideae. BMC Genomics. 21(1):393.
- Chen JX, Wang L, Zhao YC, Qin MJ. 2021. The complete chloroplast genome of a Chinese medicinal plant, Peristrophe japonica (Thunb.) Bremek. (Lamiales: canthaceae) from Nanjing, China. Mitochondrial DNA B Resour. 6(7):1888–1889.
- Darriba D, Posada D, Kozlov AM, Stamatakis A, Morel B, Flouri T. 2020. ModelTest-NG: a new and scalable tool for the selection of DNA and protein evolutionary models. Mol Biol Evol. 37(1):291–294.
- Doyle JJ, Doyle JL. 1987. A rapid DNA isolation procedure for small quantities of fresh leaf tissue. Phytochem Bull. 19:11–15.
- Flora of China. 2002a. 70:31. [1]
- Flora of China. 2002b. 70:38. [2]
- Gao X, Wang HX, Zhu ZX, Wang HF. 2020. Complete plastome sequence of Pseuderanthemum haikangense C.Y. Wu & H.S. Lo (Acanthaceae): a medicinal plant in South China. Mitochondrial DNA B Resour. 5(3):3197–3198.
- Han S, Wang R, Hong X, Wu C, Zhang S, Kan X. 2022. Plastomes of Bletilla (Orchidaceae) and Phylogenetic Implications. IJMS. 5; 23(17):10151.
- Huang SN, Deng YF, Ge XJ. 2019. The complete chloroplast genome of Aphelandra knappiae (Acanthaceae). Mitochondrial DNA Part B. 4(1):273–274.
- Huang SN, Ge XJ, Cano A, Salazar BGM, Deng YF. 2020. Comparative analysis of chloroplast genomes for five Dicliptera species (Acanthaceae): molecular structure, phylogenetic relationship, and adaptive evolution. Peer J. 8:e8450.
- Jin JJ, Yu WB, Yang JB, Song Y, Yi TS, Depamphilis CW, Li DZ. 2020. GetOrganelle: a fast and versatile toolkit for accurate de novo assembly of organelle genomes. Genome Biol: 21(1):241.
- Kaewdaungdee S, Sudmoon R, Tanee T, Lee SY, Chaveerach A. 2022. Chloroplast Genome Analysis for Genetic Information and Authentication in Five Barleria Species. Genes. 13(10):1705.
- Katoh K, Standley DM. 2013. MAFFT multiple sequence alignment software version 7: improvements in performance and usability. Mol Biol Evol. 30(4):772–780.
- Li H, Ma DN, Li J, Wei MY, Zheng HL, Zhu XY. 2020. Illumina sequencing of complete chloroplast genome of Avicennia marina, a pioneer mangrove species. Mitochondrial DNA B Resour. 5(3):2131–2132.
- Li MN, Pei HQ, Wang JH, Zhao KK, Zhu ZH, Wang HF. 2019. Complete plastome sequence of Clinacanthus nutans (Acanthaceae): a medicinal species in Southern China. Mitochondrial DNA Part B. 4(1):118–119.
- Li Y, Yang Y, Yu L, Du X, Ren G. 2018. Plastomes of nine hornbeams and phylogenetic implications. Ecol Evol. 8(17):8770–8778.
- Liu SY, Ni Y, Li JL, Zhang XY, Yang HY, Chen HM, Liu C. 2023. CPGView: a package for visualizing detailed chloroplast genome structures. Mol Ecol Resour. 23(3):694–704.
- McDade LA, Daniel TF, Kiel CA, Borg AJ. 2012. Phylogenetic placement, delimitation, and relationships among genera of the enigmatic Nelsonioideae (Lamiales: Acanthaceae). Taxon. 61(3):637–651.
- Mower JP, Guo W, Partha R, Fan W, Levsen N, Wolff K, Nugent JM, Pabon-Mora N, Gonzalez F. 2021. Plastomes from tribe Plantagineae (Plantaginaceae) reveal infrageneric structural synapormorphies and localized hypermutation for Plantago and functional loss of ndh genes from Littorella. Molecular Phylogenet Evol. 162:107217.
- Robert W, Scotland. 1992. Systematics, similarity and Acanthaceae pollen morphology[J]. Botanical Journal of the Linnean Society.109(4):529–541.
- Ronquist F, Teslenko M, Van Der Mark P, Ayres DL, Darling A, Höhna S, Larget B, Liu L, Suchard MA, Huelsenbeck JP. 2012. MrBayes 3.2: efficient Bayesian phylogenetic inference and model choice across a large model space. Syst Biol. 61(3):539–542.
- Ruang-Areerate P, Yoocha T, Kongkachana W, Phetchawang P, Maknual C, Meepol W, Jiumjamrassil D, Pootakham W, Tangphatsornruang S. 2022. Comparative analysis and phylogenetic relationships of Ceriops Species (Rhizophoraceae) and Avicennia lanata (Acanthaceae): Insight into the chloroplast genome evolution between middle and seaward zones of mangrove forests. Biology. 11(3):383.
- Shi LC, Chen HM, Jiang M, Wang LQ, Wu X, Huang LF, Liu C. 2019. CPGAVAS2, an integrated plastome sequence annotator and analyzer. Nucleic Acids Research. 47(W1):W65–W73.
- Stamatakis A. 2006. RAxML-VI-HPC: maximum likelihood-based phylogenetic analyses with thousands of taxa and mixed models. Bioinformatics. 22(21):2688–2690.
- Tillich M, Lehwark P, Pellizzer T, Ulbricht-Jones ES, Fischer A, Bock R, Greiner S. 2017. GeSeq – versatile and accurate annotation of organelle genomes. Nucleic Acids Research. 45(W1):W6–W11.
- Tong LL, Tian L, Xu XG, Cheng Y. 2022. The complete chloroplast genome sequence of Thunbergia erecta (Benth.) T. Anders. (Acanthaceae). Mitochondrial DNA B Resour. 7(11):1952–1954.
- Xu L, Wang XR, Sun K, Yu T, Xu JH, Ding PX, Tang LM, Zhang DX, Guan WB. 2021. The complete chloroplast genome of Acanthus ilicifolius, an excellent mangrove plant. Mitochondrial DNA B Resour. 6(4):1283–1284.
- Zhang YM, Qian ZG, Zhang AL, Yang CW, Li GD, Liu XL. 2019. The complete plastid genome sequence of Neopicrorhiza scrophulariiflora (Plantaginaceae): an endangered species endemic to The Himalayas regions. Mitochondrial DNA B Resour. 4(2):2504–2505.