Abstract
Elymus magellanicus (É.Desv.) Á.Löve is a foliage accent plant that found in South America. In this study, the mitochondrial genome of E. magellanicus was sequenced, assembled, and annotated. The complete circular mitogenome of E. magellanicus is 583,450 bp in length and the overall G + C content of mitogenome is 42.27%. It harbors 39 protein-coding genes, 64 transfer RNA genes, eight ribosomal RNA genes, and 20 simple sequence repeats. Phylogenetic analysis indicates a relatively close relationship of E. magellanicus to Hordeum vulgare subsp. Spontaneum, Aegilops speltoides, Triticum aestivum, and T. aestivum cv. Chinese Yumai.
Introduction
The genus Elymus L. (Poaceae, Triticeae) is mainly distributed in Eurasia and northern North America. The plastome of E. tangutorum (Jing et al. Citation2019), the chloroplast genome of E. kamoji and E. magellanicus (Liu et al. Citation2021; Wu et al. Citation2022) have been reported, which provide a basis for the judgment of the relationship of related species and genera, and provides more support for plant phylogeny research. However, there is little research on the mitochondrial genome of E. magellanicus. E. magellanicus mainly known as blue wheatgrass, is a perennial grass with distinctive metallic-blue leaves, which is native to the mountains of Chile and Argentina (). E. magellanicus is tetraploid with an SSHH genome type and has many excellent traits such as drought tolerance, salt–alkali tolerance, barren tolerance, and disease resistance (Jensen Citation1993; Wu et al. Citation2022). Breeding efforts are ongoing in China trying to use it for genetic improvement of wheat. This is the first study to report the complete mitochondrial genome sequence of E. magellanicus, which will provide genomic and genetic sources for further research.
Materials and methods
The specimens were collected from the Botanical Garden of Center of Wheat Research, Xinxiang, Henan, China (113°88′E 35°30′N) in June 2022, under the permission granted by Henan Institute of Science and Technology. A voucher specimen was deposited at the Herbarium of Henan Institute of Science and Technology, Xinxiang, China (Zhengang Ru; [email protected]) under the accession number XM-W046. Mitochondria were isolated from leaves by using density gradient centrifugation and digested with DNase I (Promega, Madison, USA) to eliminate genomic DNA contamination. To obtain a full-length mitogenome sequence, we used both shot-read (Illumina NovaSeq 6000 platform) and long-read sequencing (PacBio Sequel platform) technologies in this study. The mitogenomes of higher plants are markedly variable in both structure and sequence content. To improve assembly reliability, we used two strategies to assemble the E. magellanicus mitogenome. In the first strategy, the short clean reads were de novo assembled with GetOrganelle v1.7.1 (Jin et al. Citation2020), and potential mitochondrial contigs were extracted by aligning against the mitochondrial protein-coding genes (PCGs) from plant mitogenome database (ftp://ftp.ncbi.nlm.nih.gov/refseq/release/mitochondrion/) with BLAST v 2.8.1+. Then, the putative long mitochondrial reads were baited by mapping the Pacbio long reads to the potential mitochondrial contigs using BLASR v5.1 (Chaisson and Tesler Citation2012). Finally, the putative long mitochondrial reads were assembled by Canu v2.1.1 (Koren et al. Citation2017). In the second strategy, all Pacbio long reads were assembled de novo by using Canu directly. Finally, the corrected contigs obtained from the above two assembly strategies were aligned with each other using MUMmer 3.23 (Kurtz et al. Citation2004), and the result showed that these two contigs were identical. Based on the above assembly steps, we obtained a master circle of the E. magellanicus mitogenome.
The E. magellanicus mitogenome sequences were annotated using the online GeSeq tool (Tillich et al. Citation2017) with default parameters to predict PCGs, transfer RNA (tRNA) genes, and ribosomal RNA (rRNA) genes. In addition, the simple sequence repeats (SSRs) were detected in the mitogenome using MISA (MIcroSAtellite Identification Tool; Beier et al. Citation2017). The mitochondrial genome map of E. magellanicus was drawn using the OGView tool (http://www.1kmpg.cn/ogview; ). The phylogenetic tree was constructed based on functional protein-coding sequences (CDS), aligned with the online tool MUSCLE under default setting (Madeira et al. Citation2019), and then performed the maximum likelihood (ML) phylogenetic analysis using PhyML 3.0 (Guindon et al. Citation2010; ).
Figure 2. Mitochondrial genome map of Elymus magellanicus. The assembled mitochondrial genome (583,450 bp) of E. magellanicus (GenBank ID: OQ086977) with major features: there are 39 protein-coding genes, 64 tRNA genes, and eight rRNA genes. The colored parabola in the center circle represents the dispersed repeats. This map was drawn using the OGView tool.
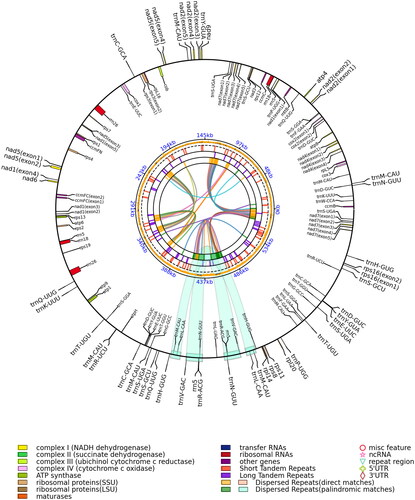
Figure 3. The maximum likelihood (ML) phylogenetic tree was constructed based on mitochondrial protein-coding genes of 10 species. The following sequences were used: Elymus magellanicus OQ086977, Aegilops speltoides AP013107.1, Hordeum vulgare subsp. Spontaneum MN127974.1, Tripsacum dactyloides cultivar Pete DQ984517.1, Tripsacum dactyloides NC_008362.1, Zea mays subsp. Parviglumis DQ645539.1, Triticum aestivum cultivar Chinese Yumai NC_036024.1 (Cui et al. Citation2009), Triticum aestivum AP008982.1 (Ogihara et al. Citation2005), Oryza coarctata MG429050.1 (Mondal et al. Citation2017), Oryza rufipogon AP011076.1 (Fujii et al. Citation2010). Numbers next to each node indicate bootstrap support values.
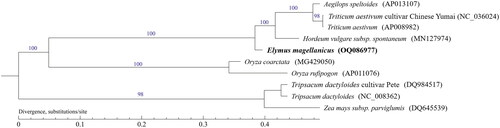
Results
The entire mitogenome of E. magellanicus (GenBank accession number: OQ086977) was circular in shape with 583,450 bp in length. The overall base composition of mitogenome was 28.73% A, 21.06% G, 21.20% C, and 29.00% T, with a GC content of 42.27%. The mitogenome encoded 111 genes, including 39 PCGs, 64 tRNA genes, and eight rRNA genes. The cumulative length of the 39 PCGs is 32,028 bp, and the PCGs were between 207 bp and 2013 bp in length., which accounts for 5.49% of the complete genome. The cumulative length of the tRNA genes is 4833 bp, with an average gene length of 75 bp, accounting for 0.83% of the complete genome. The lengths of rRNA genes are 1975 bp (rrn18), 3466 bp (rrn26), and 99 bp (rrn5), which together account for 1.93% of the complete genome. The cumulative length of 20 SSR loci identified is 234 bp which comprises approximately 0.04% of the genome length. Thirty-seven of the 39 PCGS started with ATG, and the remaining one started with ACG (nad1) and the other with AGA (rps19). Ten of 39 PCGs had stop codons TGA (ccmB, cox3, nad4, rps12, rps1, ccmFC, rps13, atp6, atp9, atp1), nine had TAG (atp4, mttB, ccmC, nad7, cob, rps3, ccmFN, rps2, nad7), and the remaining 20 ended with TAA (rpL5, atp8, cox2, nad2, nad1, nad3, nad9, nad5, rpL16, cox1, rps7, rps4, nad6, rps19, atpH, rpl14, rps8, rps11, rpl20, rps16). To investigate the evolutionary relationship of E. magellanicus, we performed a phylogenetic analysis based on nucleotide sequences of 10 complete mitochondrial genomes. The results of phylogenetic analysis supported a relatively close relationship of E. magellanicus to Hordeum vulgare subsp. Spontaneum, Aegilops speltoides, Triticum aestivum, and T. aestivum cv. Chinese Yumai ().
Discussion and conclusion
In this study, the mitochondrial genome of E. magellanicus was assembled and analyzed. In addition, the complete mitochondrial genome sequences of other Elymus species have not been seen yet. The results of the constructed phylogenetic tree showed with strong support that a relatively close relationship of E. magellanicus to Triticeae species compared with Oryzeae and tribe Maydeae species. Our research institute has made good progress in the research of common wheat and E. magellanicus hybridization, and it is also very supportive of this clustering result. The complete mitogenome of E. magellanicus reported in this study will provide important information for improving the taxonomic system and phylogenetics of Elymus.
Author contributions
Conception and design: Xiangdong Chen, Xiaojun Wu, and Zhengang Ru; analysis and interpretation of the data: Jinlong Zhang, Man Zhang, and Junchao You; drafting of the paper, revising it critically for intellectual content: Xiangdong Chen and Xiaojun Wu; all authors have approved the final version of the manuscript and all authors agree to be accountable for all aspects of the work.
Ethical statement
Specimen collection conforms to the requirements of international ethics. No endangered or protected species were involved in the study. The experimental procedures and objectives of this study were in accordance with the rules and regulations of our institution. There are no ethical issues in this study.
Disclosure statement
No potential conflict of interest was reported by the authors.
Data availability statement
The genome sequence data that support the findings of this study are openly available in GenBank of NCBI at (https://www.ncbi.nlm.nih.gov/), reference number OQ086977. The associated BioProject, Bio-Sample, and SRA numbers are PRJNA912383, SAMN32240077, and SRP413342, respectively.
Additional information
Funding
References
- Beier S, Thiel T, Münch T, Scholz U, Mascher M. 2017. MISA-web: a web server for microsatellite prediction. Bioinformatics. 33(16):2583–2585. doi: 10.1093/bioinformatics/btx198.
- Chaisson MJ, Tesler G. 2012. Mapping single molecule sequencing reads using basic local alignment with successive refinement (BLASR): application and theory. BMC Bioinf. 13:238. doi: 10.1186/1471-2105-13-238.
- Cui P, Liu H, Lin Q, Ding F, Zhuo GY, Hu SN, Liu DC, Yang WL, Zhan KH, Zhang AM, et al. 2009. A complete mitochondrial genome of wheat (Triticum aestivum cv. Chinese Yumai), and fast evolving mitochondrial genes in higher plants. J Genet. 88(3):299–307. doi: 10.1007/s12041-009-0043-9.
- Fujii S, Kazama T, Yamada M, Toriyama K. 2010. Discovery of global genomic re-organization based on comparison of two newly sequenced rice mitochondrial genomes with cytoplasmic male sterility-related genes. BMC Genomics. 11:209. doi: 10.1186/1471-2164-11-209.
- Guindon S, Dufayard JF, Lefort V, Anisimova M, Hordijk W, Gascuel O. 2010. New algorithms and methods to estimate maximum-likelihood phylogenies: assessing the performance of PhyML 3.0. Syst Biol. 59(3):307–321. doi: 10.1093/sysbio/syq010.
- Jensen K. 1993. Cytogenetics of Elymus magellanicus and its intra- and inter-generic hybrids with Pseudoroegneria spicata, Hordeum violaceum, E. trachycaulus, E. lanceolatus, and E. glaucus (Poaceae: Triticeae). Genome. 36(1):72–76. doi: 10.1139/g93-010.
- Jin JJ, Yu WB, Yang JB, Song Y, dePamphilis CW, Yi TS, Li DZ. 2020. GetOrganelle: a fast and versatile toolkit for accurate de novo assembly of organelle genomes. Genome Biol. 21(1):241. doi: 10.1186/s13059-020-02154-5.
- Jing M, Ma Y, Li H, Wang J. 2019. Characterization of the complete plastome of Elymus tangutorum (Poaceae: Triticeae). Mitochondrial DNA B Resour. 4(2):3356–3357. doi: 10.1080/23802359.2019.1674217.
- Koren S, Walenz BP, Berlin K, Miller JR, Bergman NH, Phillippy AM. 2017. Canu: scalable and accurate long-read assembly via adaptive k-mer weighting and repeat separation. Genome Res. 27(5):722–736. doi: 10.1101/gr.215087.116.
- Kurtz S, Phillippy A, Delcher AL, Smoot M, Shumway M, Antonescu C, Salzberg SL. 2004. Versatile and open software for comparing large genomes. Genome Biol. 5(2):R12. doi: 10.1186/gb-2004-5-2-r12.
- Liu M, Zhang Y, Zhang Y, Cui G, Zhou Q, Wei X. 2021. Characterization of the complete chloroplast genome of Elymus kamoji (Ohwi) S. L. Chen. Mitochondrial DNA B Resour. 6(11):3177–3178. doi: 10.1080/23802359.2021.1882898.
- Madeira F, Park YM, Lee J, Buso N, Gur T, Madhusoodanan N, Basutkar P, Tivey ARN, Potter SC, Finn RD, et al. 2019. The EMBL-EBI search and sequence analysis tools APIs in 2019. Nucleic Acids Res. 47(W1):W636–W641. doi: 10.1093/nar/gkz268.
- Mondal TK, Rawal HC, Gaikwad K, Sharma TR, Singh NK. 2017. First de novo draft genome sequence of Oryza coarctata, the only halophytic species in the genus Oryza. F1000Res. 6:1750. doi: 10.12688/f1000research.12414.2.
- Ogihara Y, Yamazaki Y, Murai K, Kanno A, Terachi T, Shiina T, Miyashita N, Nasuda S, Nakamura C, Mori N, et al. 2005. Structural dynamics of cereal mitochondrial genomes as revealed by complete nucleotide sequencing of the wheat mitochondrial genome. Nucleic Acids Res. 33(19):6235–6250. doi: 10.1093/nar/gki925.
- Tillich M, Lehwark P, Pellizzer T, Ulbricht-Jones E, Fischer A, Bock R, Greiner S. 2017. GeSeq-versatile and accurate annotation of organelle genomes. Nucleic Acids Res. 45(W1):W6–W11. doi: 10.1093/nar/gkx391.
- Wu X, Chen X, Huang Z, Ren C, Hu T, Ru Z. 2022. De novo assembly and characterization of the complete chloroplast genome of Elymus magellanicus (É.Desv.) Á.Löve, 1984 (Poaceae, Pooideae). Mitochondrial DNA B Resour. 7(10):1873–1875. doi: 10.1080/23802359.2022.2135400.