Abstract
Castanopsis hystrix Hook. f. & Thomson ex A. DC. 1863 (Fagaceae) is an evergreen broad-leaved tree with high economic and ecological value. In this study, the complete chloroplast genome of C. hystrix was sequenced, assembled and annotated. The plastome (plastid genome) of C. hystrix was 160,624 bp in size, consisting of a pair of inverted repeats (IRs, 25,699 bp), a large-single-copy (LSC, 90,276 bp) region, and a small-single-copy (SSC, 18,950 bp). The overall GC content of C. hystrix was 36.8%. A total of 133 genes were annotated, including 88 protein-coding genes (PCG), 37 transfer RNA genes (tRNA), and eight ribosomal RNA genes (rRNA). A maximum likelihood analysis showed that the Castanopsis species form a monophyletic clade. C. hystrix is most closely related to C. tibetana with 100% bootstrap support value. The result enriches the genomic data for the genus Castanopsis, which will contribute to future studies in phylogenetics and evolution.
Introduction
Castanopsis hystrix Hook. f. & Thomson ex A. DC. 1863, an evergreen broad-leaved tree belonging to the family Fagaceae, is mainly distributed in low altitude areas of tropical and subtropical Asia (Huang et al. Citation1999). Its timber can be used in furniture production, the shipbuilding industry, and infrastructure construction. The seeds of this species are the preferred material for making wine and feed (Zhou Citation2007). The abundant litterfall of C. hystrix helps maintain the global carbon cycle in forest ecosystems (Liu et al. Citation2012). Genomic characterization is the most essential step in breeding programs. However, the genomic information of C. hystrix is poorly understood. In this study, the chloroplast genome of C. hystrix was sequenced, which can provide a reference for exploration, utilization and conservation and provide basic genetic resources for further genetic and phylogenetic studies.
Materials and methods
Fresh and mature leaves of Castanopsis hystrix () were obtained with permission from the Guangxi Forestry Research Institute (latitude 22.93 N, longitude 108.36E), and voucher specimen (collection number: 20210317001) was kept at the Guangxi Forestry Research Institute (http://www.gxlky.com.cn/index.php?siteid=6, Hui Zhu, [email protected]). Total genomic DNA was extracted using a DNeasy Plant Mini Kit (Qiagen, Germany). The library with insert sizes of 300–500 bp was constructed and sequenced on the Illumina HiSeq X Ten platform (San Diego, USA) at Novogene (Beijing, China). Approximately 2 Gb of clean data was obtained after filtering the adaptors and low-quality bases sequences by Trimmomatic (Bolger et al. Citation2014). NOVOPlasty (Dierckxsens et al. Citation2017) was employed to de novo assemble the paired-ends reads. The sequencing depth coverage was conducted by Geneious (Kearse et al. Citation2012). The chloroplast genome annotations of C. hystrix were generated by GeSeq (https://chlorobox.mpimp-golm.mpg.de/geseq.html, Tillich et al. Citation2017) and adjusted manually using Geneious. The map of chloroplast genome, cis-splicing genes and trans-splicing gene of C. hystrix was drawn by CPGview (http://www.1kmpg.cn/cpgview/, Liu et al. Citation2023). Full plastomes of 19 species were aligned by MAFFT (Katoh and Standley Citation2013), of which 18 were retrieved from the NCBI database. Two taxa, namely, Quercus aquifolioides (KX911971) and Q. tungmaiensis (MF593893), were chosen as outgroups according to a previous study (Ye et al. Citation2019), the maximum likelihood (ML) tree was constructed in RAxML (Stamatakis Citation2014) with 1000 bootstrap replicates and the best-fitting GTR + GAMMA model to investigate the phylogenetic relationships of C. hystrix within the genus Castanopsis.
Figure 1. The photograph of Castanopsis hystrix. This image was taken by Hui Zhu at Guangxi Forestry Research Institute, Nanning Guangxi, China. C. hystrix is an evergreen broad-leaved tree with leaf blade margin shallowly, serrate from middle to apex, secondary veins usually not reaching margin and petiole rarely longer than 1 cm.
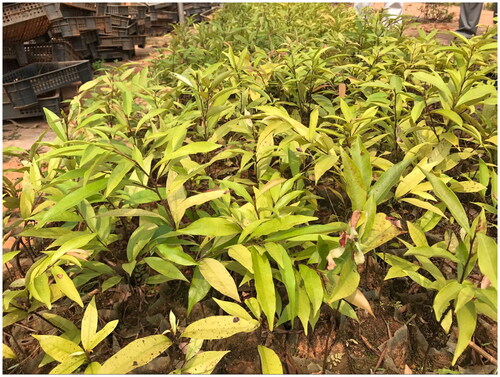
Results
A total of 740,890 reads were assembled to the complete plastid genome of Castanopsis hystrix with an average coverage of 692 (Figure S1). The assembled plastid genome was finally deposit to the NCBI (National Center for Biotechnology Information, https://www.ncbi.nlm.nih.gov/) with accession number ON184044. The plastome possesses a quadripartite structure with 160,624 bp in length, consisting of a pair of inverted repeats (IRs, 25,699 bp), a large-single-copy (LSC, 90,276 bp) region, and a small-single-copy (SSC, 18,950 bp) (). The plastome of C. hystrix was AT-rich, and the overall GC content was 36.8%, with that of IRs, SSC and LSC at 42.8%, 34.6%, and 30.9%, respectively. A total of 133 genes were annotated, including 88 protein-coding genes (PCG), 37 transfer RNA genes (tRNA), and eight ribosomal RNA genes (rRNA). Of these, 18 genes were duplicated in IR regions, including 7 PCGs (rpl2, rpl23, ycf2, ndhB, rps7, rps12 and ycf15), 7 tRNA genes (trnI-CAT, trnL-CAA, trnV-GAC, trnI-GAU, trnA-UGC, trnR-ACG and trnN-GTT), and 4 rRNA genes (rrn16, rrn23, rrn4.5 and rrn5). In addition, we detected 11 cis-splicing (intron removal) genes, namely rps16, atpF, rpoC1, ycf3, clpP, petB, petD, rpl16, rpl2(*2), ndhB(*2), and ndhA (Figure S2) and one trans-splicing (spliced leader addition) genes rps12 (Figure S3).
Figure 2. The complete chloroplast genome map of Castanopsis hystrix, which was generated by CPGview. LSC, SSC, and IR (IRa and IRb) with their length are represented on the first circle. The second circle shows the GC ratio in dark gray. The third circle displays the genes with the colors based on their functional classification presented at the left of the circular map. Genes located on the inner are transcribed in a clockwise, and those outer of circle are transcribed in an anticlockwise.
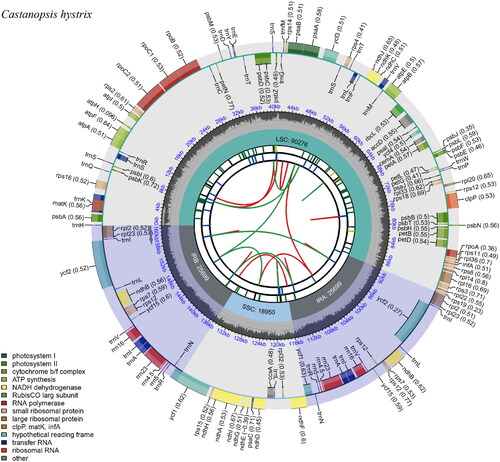
A maximum likelihood phylogenetic analysis shows the genus Castanopsis forms a monophyletic clade (). Three different accessions of C. hystrix (ON184044, MZ433364, OM321038) are clustered together as a sister group to C. tibetana (ON710842), with a statistical value of 100%. However, one C. hystrix (OQ024217) sample is grouped together with C. echinocarpa (KJ001129). In addition, the sister species pairs, C. hainanensis (MG383644), C. lamontii (OQ024218) and C. sclerophylla (MK387847), C. concinna (KT793041) and C. fordii (ON710841) had 98% and 95% support, respectively.
Figure 3. A maximum-likelihood (ML) based phylogenetic tree of Castanopsis hystrix using Quercus aquifolioides and Q. tungmaiensis as outgroups. The scale bar represents the number of nucleotide substitutions per site. The numbers on each node indicated the ML bootstrap support with 1000 replicates. The following sequences were used: Castanea seguinii MF996572, Castanea henryi MH998384 (Gao et al. Citation2019), Castanopsis carlesii MK745999 (Sun et al. Citation2019), Castanopsis concinna KT793041, Castanopsis echinocarpa KJ001129, Castanopsis fargesii MK571045 (Ye et al. Citation2019), Castanopsis fordii ON710841 (Wang et al. Citation2023), Castanopsis hystrix OQ024217, Castanopsis hystrix ON184044 (this study), Castanopsis hystrix OM321038, Castanopsis hystrix MZ433364, Castanopsis sieboldii MZ028444 (Park et al. Citation2021), Castanopsis hainanensis MG383644 (Chen et al. Citation2018), Castanopsis mekongensis MW043480 (Peng et al. Citation2021), Castanopsis sclerophylla MK387847 (Ye et al. Citation2019), Castanopsis tibetana ON710842, Quercus aquifolioides KX911971 (Yang et al. Citation2018), Quercus tungmaiensis MF593893 (Yang et al. Citation2018).
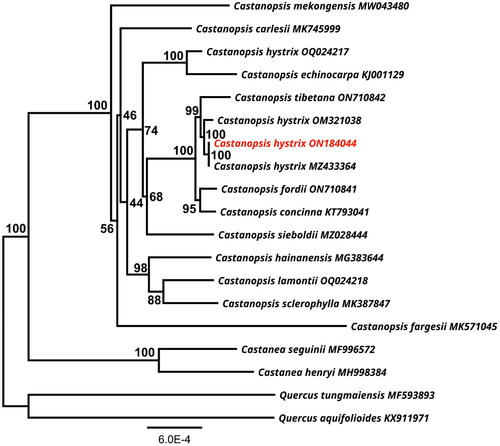
Discussion and conclusion
Castanopsis is one of the three largest genera in the Fagaceae family (Huang et al. Citation2023). However, the phylogenetic relationship and species identification remain poorly resolved due to phenotypic diversity, coupled with hybridization issue. Plastome data could be an ideal tool in resolving the phylogenetic relationships and species identification. Castanopsis hystrix is of great economic and ecological value. In this study, the chloroplast genome of C. hystrix has been sequenced, assembled and annotated. The structural characteristics of C. hystrix is similar to most angiosperm, which shows the typical four parts, consisting of a pair of inverted repeats, a large-single-copy region, and a small-single-copy (Palmer Citation1985). By comparing with previously known genomes of C. hystrix, the high-level conserved chloroplast genome was confirmed which could be demonstrated as a super-DNA barcode to distinguish C. hystrix from the related Castanopsis species. Additionally, the presumably misidentification of C. hystrix (OQ024217) was also recognized in the present study. Moreover, the phylogenetic position of C. hystrix in the genus Castanopsis has been clearly resolved. The result enriches the genomic data for the genus Castanopsis, which will contribute to phylogenetic and evolutionary studies in future.
Ethical approval
The sample of Castanopsis hystrix was collected with the permission from the Guangxi Forestry Research Institute and strictly complied with local and Chinese regulations. No ethical approval is required in this study.
Author contributions
Zifan Gui and Hui Zhu were involved in the conception and design. Yueqi Sun, Dong Lin, Lianxiang Zhong Qiulan Wei and Mimi Li were involved in analysis the data. Zifan Gui and Yueqi Sun were involved in interpretation of the drafting of the paper. Zifan Gui, Hui Zhu and Mimi Li revised it critically for intellectual content and the final approval of the version to be published. All authors agree to be accountable for all aspects of the work.
Supplemental Material
Download MS Word (503 KB)Disclosure statement
No potential conflict of interest was reported by the author(s).
Data availability statement
The genome sequence data that support the findings of this study are openly available in GenBank of NCBI at (https://www.ncbi.nlm.nih.gov/) under the accession number ON184044. The associated BioProject, SRA, and Bio-Sample numbers are PRJNA828973, SRR18843872 and SAMN27681347, respectively.
Additional information
Funding
References
- Bolger AM, Lohse M, Usadel B. 2014. Trimmomatic: a flexible trimmer for Illumina sequence data. Bioinformatics. 30(15):2114–2120. doi:10.1093/bioinformatics/btu170.
- Chen X, Yang J, Yang Y, Zhang X, Du X, Zhao G. 2018. Characterization of the complete plastid genome of Castanopsis hainanensis Merrill. Conservation Genet Resour. 10(4):825–828. doi:10.1007/s12686-017-0940-9.
- Dierckxsens N, Mardulyn P, Smits G. 2017. NOVOPlasty: de novo assembly of organelle genomes from whole genome data. Nucleic Acids Res. 45(4):e18. doi:10.1093/nar/gkw955.
- Gao XX, Yan F, Liu M, Zulfiqar S, Zhao P. 2019. The complete chloroplast genome sequence of an endemic species Pearl chestnut (Castanea henryi). Mitochondrial DNA B Resour. 4(1):551–552. doi:10.1080/23802359.2018.1553522.
- Huang CJ, Zhang YT, Bartholo B. 1999. Fagaceae. In: Wu ZY, Raven PH, Hong DY, editors. Flora of China Volume 4 (Fagaceae). Beijing & St. Louis: Science Press & Missouri Botanical Garden Press.
- Huang W, Liao B, Liu H, Liang Y, Chen X, Wang B, Xia H. 2023. A chromosome-scale genome assembly of Castanopsis hystrix provides new insights into the evolution and adaptation of Fagaceae species. Front Plant Sci. 14:1174972. doi:10.3389/fpls.2023.1174972.
- Kearse M, Moir R, Wilson A, Stones-Havas S, Cheung M, Sturrock S, Buxton S, Cooper A, Markowitz S, Duran C, et al. 2012. Geneious Basic: an integrated and extendable desktop software platform for the organization and analysis of sequence data. Bioinformatics. 28(12):1647–1649. doi:10.1093/bioinformatics/bts199.
- Liu S, Ni Y, Li J, Zhang X, Yang H, Chen H, Liu C. 2023. CPGView: A package for visualizing detailed chloroplast genome structures. Mol Ecol Resour. 23(3):694–704. doi:10.1111/1755-0998.13729.
- Katoh K, Standley DM. 2013. MAFFT multiple sequence alignment software version 7: improvements in performance and usability. Mol Biol Evol. 30(4):772–780. doi:10.1093/molbev/mst010.
- Liu E, Wang H, Liu SR. 2012. Characteristics of carbon storage and sequestration in different age beech (Castanopsis hystrix) plantations in south subtropical area of China. Ying Yong Sheng Tai Xue Bao. 23:335–340.
- Palmer JD. 1985. Comparative Organization of Chloroplast Genomes. Annu Rev Genet. 19(1):325–354. doi:10.1146/annurev.ge.19.120185.001545.
- Park J, Xi H, Son J, Shin HT, Kang H, Park S. 2021. The complete chloroplast genome of Castanopsis sieboldii (Makino) Hatus (Fagaceae). Mitochondrial DNA B Resour. 6(9):2743–2745. doi:10.1080/23802359.2021.1966339.
- Peng J, Yang L, Duan A, Wang D, Li S. 2021. The complete chloroplast genome of Castanopsis mekongensis A. Camus (Fagaceae). Mitochondrial DNA B Resour. 6(1):168–170. doi:10.1080/23802359.2020.1860707.
- Stamatakis A. 2014. RAxML version 8: a tool for phylogenetic analysis and post-analysis of large phylogenies. Bioinformatics. 30(9):1312–1313. doi:10.1093/bioinformatics/btu033.
- Sun RX, Ye XM, Wang ZL, Lin XF. 2019. The complete chloroplast genome of Castanopsis carlesii (Hemsl.) Hay. Mitochondrial DNA B Resour. 4(2):2591–2592. doi:10.1080/23802359.2019.1641437.
- Tillich M, Lehwark P, Pellizzer T, Ulbricht-Jones ES, Fischer A, Bock R, Greiner S. 2017. GeSeq – versatile and accurate annotation of organelle genomes. Nucleic Acids Res. 45(W1):W6–W11. doi:10.1093/nar/gkx391.
- Wang YF, Huang YL, He RJ, Yang BY, Liu ZB. 2023. The complete chloroplast genome sequence of Castanopsis fordii Hance (Fagaceae). Mitochondrial DNA B Resour. 8(2):236–239. doi:10.1080/23802359.2023.2167477.
- Yang Y, Zhang H, Ren T, Zhao G. 2018. Characterization of the complete plastid genome of Quercus tungmaiensis. Conservation Genet Resour. 10(3):457–460. doi:10.1007/s12686-017-0848-4.
- Yang Y, Zhu J, Feng L, Zhou T, Bai G, Yang J, Zhao G. 2018. Plastid genome comparative and phylogenetic analyses of the key genera in Fagaceae: highlighting the effect of codon composition bias in phylogenetic inference. Front Plant Sci. 9:82. doi:10.3389/fpls.2018.00082.
- Ye X, Guo Y, Lei X, Sun R. 2019. The complete chloroplast genome of Castanopsis fargesii Franch. (Fagaceae), Mitochondrial DNA B Resour. 4(1):1656–1657. doi:10.1080/23802359.2019.1605850.
- Ye X, Hu D, Guo Y, Sun R. 2019. Complete chloroplast genome of Castanopsis sclerophylla (Lindl.) Schott: genome structure and comparative and phylogenetic analysis. PLoS One. 14(7):e0212325. doi:10.1371/journal.pone.0212325.
- Zhou C. 2007. Research Overview and Biological Characteristics of Precious Timber Tree Species-Castanopsis hystrix. Jiangxi Forestry Sci Technol. 5:29–31.