Abstract
In this study, we sequenced and assembled the complete chloroplast genome of Chloranthus nervosus Collett ex Hemsl. 1890. The total length of the complete chloroplast sequence was found to be 158,002 bp. It consisted of a large single-copy (LSC) region of 87,127 bp, a small single-copy (SSC) region of 18,541 bp, and a pair of inverted repeat (IR) regions, each with a length of 26,167 bp. The overall GC content of the complete chloroplast genome was 38.9%, with the LSC region, SSC region, and IR regions exhibiting GC contents of 37.4%, 34.1%, and 43.1%, respectively. The annotation of the chloroplast genome revealed a total of 131 genes, comprising 86 protein-coding genes, 37 tRNA genes, and eight rRNA genes. Phylogenetic analysis revealed that the seven sampled species of Chloranthus were divided into two clades. Within the clade characterized by long filamentous anther connectives, C. nervosus showed the closest relation to C. japonicus. These findings validated the previous preliminary results on the phylogenetic relationships of the seven species of Chloranthus with strong support.
Introduction
Chloranthus Swartz (Chloranthaceae) plays a crucial role in studying the origin and early evolution of angiosperms (Friis et al. Citation1986). The genus Chloranthus contains two subgenera, i.e. subg. Tricercandra and subg. Chloranthus, and a total of 11 species (Kong Citation2000; Liu et al. Citation2019; He et al. Citation2022). The two subgenera are distinguished by specific features: subg. Tricercandra exhibits long filamentous anther connectives with thecae attached to the base, whereas subg. Chloranthus displays short, clavate, or helmet-like connectives with thecae attached to the median or apical parts (Kong Citation2000). Previous phylogenetic studies on Chloranthus, as well as Chloranthaceae, utilized sequences of a limited number of plastid sites and the nuclear ribosome ITS, which consistently supported the monophyly of Chloranthus and provided concordant and generally high resolution for intrageneric relationships, except within subg. Tricercandra where interspecific relationships had low to moderate support (Kong Citation2000; Kong and Chen Citation2000; Kong et al. Citation2002; Zhang et al. Citation2011, Citation2015). Subg. Tricercandra consists of four species: Chloranthus nervosus Collett ex Hemsl. 1890, C. japonicus, C. augustifolius, and C. fortunei. Among them, C. nervosus exhibits polymorphism in flower color, ranging from white or yellow to orange–red (Lu et al. Citation2020). Therefore, it is necessary to conduct further phylogenetic analysis to validate the relationships within subg. Tricercandra, with a particular focus on C. nervosus, using larger data that contains more informative sites, such as chloroplast genome data obtained through genome skimming sequencing.
The chloroplast genomes of six Chloranthus species have been published and are publicly available from NCBI. These genomes include all species of subg. Tricercandra except C. nervosus. In this study, we successfully assembled and annotated the complete chloroplast genome of C. nervosus () and conducted phylogenetic analysis of Chloranthus taxa, particularly in subg. Tricercandra. This study will enhance our understanding of the phylogeny of Chloranthus and provide preliminary data for future investigations on the taxonomy, phylogeography, and evolution of Chloranthus.
Materials and methods
A sample of C. nervosus was collected from Pohong Village (Nabo Town, Baise City, Guangxi, China 23°86′83″ N, 107°15′99″ E) and the voucher specimen (barcode IBK00441921) was deposited at the Herbarium of Guangxi Institute of Botany (http://www.gxib.cn/spIBK/, contact person: Chun-Rui Lin, email: [email protected]). The total DNA of C. nervosus was extracted by the CTAB method (Doyle and Doyle Citation1987). Genomic paired-end sequencing (PE150) was performed on an Illumina NovaSeq 6000 at Novogene (Tianjin, China) and approximately 3 G raw reads were generated. After quality filtering using fastp v0.20.1 (Chen et al. Citation2018), approximately 2.9 G of clean data was obtained. The complete chloroplast genome was de novo assembled using GetOrganelle v1.7.5 (Jin et al. Citation2020) with default parameters. The complete chloroplast genome was annotated using the online tool CPGAVAS2 (Shi et al. Citation2019) with C. japonious (NC_026565) as the reference. The annotation results were manually corrected using Geneious v9.0.2 (Kearse et al. Citation2012). The circular map of the chloroplast genome was generated using the CPGView program (Liu et al. Citation2023). The sequence had been submitted to GenBank under the accession number OR198055. To determine the coverage depth of each base, the clean reads were mapped to the assembled chloroplast genome using BWA-MEM v0.7.17 (Li Citation2013) and SAMtools v1.9 (Danecek et al. Citation2021). The resulting data were then used to generate a coverage depth map using OriginPro 2020 (OriginLab Corporation, Northampton, MA, USA).
To elucidate the intrageneric phylogenetic relationships, particularly the position of C. nervosus, the complete chloroplast genome sequences of 12 species were retrieved from NCBI, including six species in Chloranthus, as well as one species each from Sarcandra, Calycanthus, Drimys, Magnolia, Liriodendron, and Illicium. Illicium oligandrum was set as the outgroup according to the relationships of angiosperms (Moore et al. Citation2007). MAFFT v7.490 (Katoh and Standley Citation2013) was employed to align all sequences, and an in-house R package named alignmentFilter (freely available from: https://github.com/qiangzhang04/alignmentFilter) was used to mask ambiguously aligned segments (p < .05) and remove sites with more than 50% gaps. Subsequently, the phylogenetic tree was reconstructed using the maximum-likelihood (ML) method implemented in IQ-TREE v2.0 software (Minh et al. Citation2020). To determine the best-fit model, we employed the ModelFinder package within IQ-TREE, which identified GTR + F + R3 as the optimal model. We constructed the ML tree based on the GTR + F + R3 model, followed by 5000 ultrafast bootstraps to evaluate the branch support.
Results
The chloroplast genome of C. nervosus was composed of four regions, with a total length of 158,002 bp. The large single-copy (LSC) region spanned 87,127 bp, the small single-copy (SSC) region was 18,541 bp long, and two inverted repeat (IRa and IRb) regions, each with a length of 26,167 bp (). The overall GC content was 38.9%. Specifically, the LSC region had a GC content of 37.4%, the SSC region had a GC content of 34.1%, and the IR regions exhibited a higher GC content of 43.1%. The average coverage depth of C. nervosus was 961.5 (Figure S1). This genome contained a total of 131 genes, including 86 protein-coding genes, 37 tRNA genes, and eight rRNA genes. 11 genes contained one intron each, namely: ndhA, rpl16, petD, petB, rps16, rpoC1, atpF, rpl2, trnI-GAU, trnA-UGC, and ndhB. Only the ycf3 and clpP genes had two introns. Twenty genes were in the IR regions, including eight protein-coding genes (ycf1, rps12, rps7, ndhB, ycf2, rpl23, rpl2, and ndhF), four rRNA genes (rrn16, rrn23, rrn4.5, and rrn5) and eight tRNA genes (trnN-GUU, trnR-ACG, trnA-UGC, trnI-GAU, trnV-GAC, trnL-CAA, trnI-CAU, and trnH-GUG). There existed 11 cis-splicing genes including rps16, atpF, rpoC1, ycf3, clpP, petB, petD, rpl16, rpl2, ndhB, and ndhA (Figure S2A) and one trans-splicing gene, i.e. rps12 (Figure S2B).
Figure 2. Circular chloroplast genome map of C. nervosus. The map is made up of six circles. Starting from the center, the first circle represents the distribution of repeats. The second circle denotes the tandem repeats with short bars. Short bars in the third circle indicate microsatellite sequences. The positions of the LSC, SSC, IRa, and IRb regions are indicated on the fourth circle. The fifth circle shows the GC content. Genes with different functions are color-coded on the sixth circle, and the optional codon usage bias is shown in parentheses after the gene name.
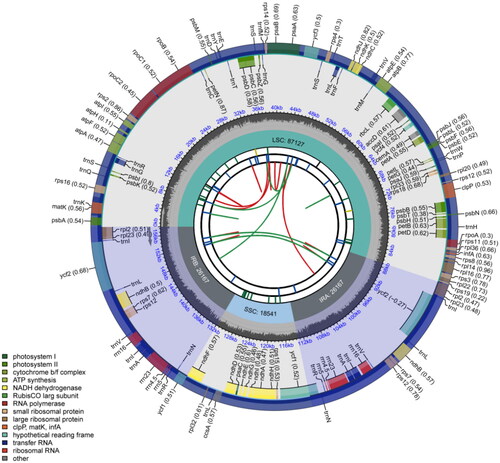
The phylogenetic analysis indicated that the seven sampled species of Chloranthus were segregated into two clades, corresponding well with the two defined subgenera (). Chloranthus nervosus was resolved as the sister to C. japonicus with maximum bootstrap value (BS = 100%). They in turn formed a strongly supported clade with a lineage consisting of C. angustifolius and C. fortunei (BS = 100%).
Figure 3. The maximum-likelihood (ML) tree was constructed based on the eight chloroplast genome sequences. The bootstrap support values are shown above branches. The following sequences were used: Chloranthus erectus NC_039627 (Zeng et al. Citation2018), C. spicatus NC_009598 (Hansen et al. Citation2007), C. henryi MK922064 (Liu et al. Citation2019), C. japonicus KP256024 (Sun et al. Citation2016), C. nervosus OR198055 (this study), C. fortunei NC_065306 (Kang et al. Citation2022), Sarcandra glabra MH939147 (Wang et al. Citation2020). Calycanthus floridus var. glaucus NC_004993 (Goremykin et al. Citation2003), Liriodendron tulipifera DQ899947, Magnolia tripetala MT682841, Drimys granadensis NC_008456 (Cai et al. Citation2006), and Illicium oligandrum NC_009600 (Hansen et al. Citation2007).
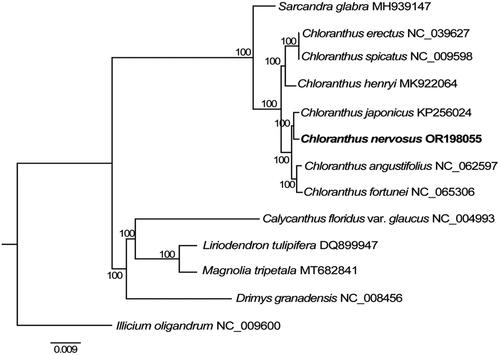
Discussion and conclusion
In this study, we successfully assembled and annotated the chloroplast genome sequence of C. nervosus for the first time. The findings from our phylogenetic analyses revealed a clear division of the seven sampled species of Chloranthus into two distinct clades that align with the two defined subgenera. Notably, C. nervosus was found to share the closest relationship with C. japonicus with strong support. The results obtained were consistent with those of previous studies. However, unlike the results of previous studies, the interspecific relationships in subg. Tricercandra in our results had a high resolution, which may be due to that the complete chloroplast genome sequences used in this study contain more informative sites, whereas only a few fragments used in the previous studies had much fewer informative sites (Kong Citation2000; Kong et al. Citation2002; Zhang et al. Citation2011, Citation2015). These results not only corroborated the preliminary relationships and further clarified unresolved interspecific relationships presented in earlier studies but also provided a robust foundation for future studies on the phylogeny and evolution of Chloranthus.
Ethical approval
The C. nervosus specimens used in this study are not endangered, protected, or privately owned. Therefore, no permission was necessary for collecting plant materials in this study. We confirm that the research adheres to ethical guidelines and local legislation.
Author contributions
Shu-Ting Yao: analyzed data and drafted the manuscript. Yong-Bin Lu: collected materials, conducted species identification, and facilitated the sample preparation for sequencing. Zhan-Jiang Zhang and Cui Li: provided financial program support and helped to revise the manuscript. Peng-Fei Wang and Xin-Mei Qin: designed the study, provided guidance for the analysis, and revised the manuscript. All authors have reviewed and approved the final manuscript for publication.
Supplemental Material
Download MS Word (218.3 KB)Disclosure statement
No potential conflicts of interest are reported by the authors.
Data availability statement
The genome sequence data that support the findings of this study are openly available in GenBank of NCBI at (https://www.ncbi.nlm.nih.gov/) under accession no. OR198055. The associated BioProject, SRA, and Bio-Sample numbers are PRJNA990663, SRR25131669, and SAMN36271954, respectively.
Additional information
Funding
References
- Cai ZQ, Penaflor C, Kuehl JV, Leebens-Mack J, Carlson JE, dePamphilis CW, Boore JL, Jansen RK. 2006. Complete plastid genome sequences of Drimys, Liriodendron, and Piper: implications for the phylogenetic relationships of magnoliids. BMC Evol Biol. 6(1):77. doi:10.1186/1471-2148-6-77.
- Chen SF, Zhou YQ, Chen YR, Gu J. 2018. fastp: an ultra-fast all-in-one FASTQ preprocessor. Bioinformatics. 34(17):i884–i890. doi:10.1093/bioinformatics/bty560.
- Danecek P, Bonfield JK, Liddle J, Marshall J, Ohan V, Pollard MO, Whitwham A, Keane T, McCarthy SA, Davies RM, et al. 2021. Twelve years of SAMtools and BCFtools. Gigascience. 10(2):giab008. doi:10.1093/gigascience/giab008.
- Doyle JJ, Doyle JL. 1987. A rapid DNA isolation procedure for small quantities of fresh leaf tissue. Phytochem Bull. 19:11–15.
- Friis EM, Crane PR, Pedersen KR. 1986. Floral evidence for Cretaceous chloranthoid angiosperms. Nature. 320(6058):163–164. doi:10.1038/320163a0.
- Goremykin V, Hirsch-Ernst KI, Wölfl S, Hellwig FH. 2003. The chloroplast genome of the “basal” angiosperm Calycanthus fertilis – structural and phylogenetic analyses. Plant Syst Evol. 242(1-4):119–135. doi:10.1007/s00606-003-0056-4.
- Hansen DR, Dastidar SG, Cai ZQ, Penaflor C, Kuehl JV, Boore JL, Jansen RK. 2007. Phylogenetic and evolutionary implications of complete chloroplast genome sequences of four early-diverging angiosperms: Buxus (Buxaceae), Chloranthus (Chloranthaceae), Dioscorea (Dioscoreaceae), and Illicium (Schisandraceae). Mol Phylogenet Evol. 45(2):547–563. doi:10.1016/j.ympev.2007.06.004.
- He LH, Zhao XL, Yu J, Zhang Z. 2022. Chloranthus coccineus (Chloranthaceae), a new species from Hubei, China. Phytotaxa. 566(3):279–289. doi:10.11646/phytotaxa.566.3.3.
- Jin JJ, Yu WB, Yang JB, Song Y, dePamphilis CW, Yi TS, Li DZ. 2020. GetOrganelle: a fast and versatile toolkit for accurate de novo assembly of organelle genomes. Genome Biol. 21(1):241. doi:10.1186/s13059-020-02154-5.
- Kang JS, Kim BY, Yoo KO. 2022. The complete plastid genome sequence of Chloranthus fortunei (A. Gray) Solms-Laub. in Chloranthaceae. Mitochondrial DNA B Resour. 7(10):1829–1833. doi:10.1080/23802359.2022.2132840.
- Katoh K, Standley DM. 2013. MAFFT multiple sequence alignment software version 7: improvements in performance and usability. Mol Biol Evol. 30(4):772–780. doi:10.1093/molbev/mst010.
- Kearse M, Moir R, Wilson A, Stones-Havas S, Cheung M, Sturrock S, Buxton S, Cooper A, Markowitz S, Duran C, et al. 2012. Geneious Basic: an integrated and extendable desktop software platform for the organization and analysis of sequence data. Bioinformatics. 28(12):1647–1649. doi:10.1093/bioinformatics/bts199.
- Kong HZ, Chen ZD. 2000. Phylogeny in Chloranthus Swartz (Chloranthaceae) inferred from sequence analysis of nrDNA ITS region. Acta Bot Sin. 42(7):762–764.
- Kong HZ, Chen ZD, Lu AM. 2002. Phylogeny of Chloranthus (Chloranthaceae) based on nuclear ribosomal ITS and plastid trnL-F sequence data. Am J Bot. 89(6):940–946. doi:10.3732/ajb.89.6.940.
- Kong HZ. 2000. Systematics of the genus Chloranthus Swartz (Chloranthaceae) [PhD dissertation]. Beijing: Institute of Botany, Chinese Academy of Sciences.
- Li H. 2013. Aligning sequence reads, clone sequences and assembly contigs with BWA-MEM. arXiv: 1303.3997v1302 [q-bio.GN].
- Liu DT, Cai L, Chen G. 2019. Chloranthus flavus (Chloranthaceae), a new species from Guangxi, China. Phytotaxa. 391(4):273–276. doi:10.11646/phytotaxa.391.4.6.
- Liu XD, Liao XY, Liu ZJ, Lan SR. 2019. Complete chloroplast genome of Chloranthus henryi (Chloranthaceae). Mitochondrial DNA B Resour. 4(2):2964–2965. doi:10.1080/23802359.2019.1637793.
- Liu SY, Ni Y, Li JJ, Zhang XY, Yang HY, Chen HM, Liu C. 2023. CPGView: a package for visualizing detailed chloroplast genome structures. Mol Ecol Resour. 23(3):694–704. doi:10.1111/1755-0998.13729.
- Lu YB, Jiang YL, Qin XM, Zhang Q. 2020. Morphological diversity and geographical distribution of Chloranthus in China. Guihaia. 40(1):31–43.
- Minh BQ, Schmidt HA, Chernomor O, Schrempf D, Woodhams MD, von Haeseler A, Lanfear R. 2020. IQ-TREE 2: new models and efficient methods for phylogenetic inference in the genomic era. Mol Biol Evol. 37(5):1530–1534. doi:10.1093/molbev/msaa015.
- Moore MJ, Bell CD, Soltis PS, Soltis DE. 2007. Using plastid genome-scale data to resolve enigmatic relationships among basal angiosperms. Proc Natl Acad Sci USA. 104(49):19363–19368. doi:10.1073/pnas.0708072104.
- Shi LC, Chen HM, Jiang M, Wang LQ, Wu X, Huang LF, Liu C. 2019. CPGAVAS2, an integrated plastome sequence annotator and analyzer. Nucleic Acids Res. 47(W1):W65–W73. doi:10.1093/nar/gkz345.
- Sun J, Zhang G, Li YM, Chen Y, Zhang XF, Tang ZS, Wu HF. 2016. The complete chloroplast genome sequence of Chloranthus japonicus. Mitochondrial DNA A DNA Mapp Seq Anal. 27(5):3202–3204. doi:10.3109/19401736.2015.1007347.
- Wang W, Zou PS, Liu GF, Dai SP. 2020. Characterization of the complete chloroplast genome sequence of Sarcandra glabra (Chloranthales). Mitochondrial DNA B Resour. 5(1):864–865. doi:10.1080/23802359.2020.1715858.
- Zeng CX, Hollingsworth PM, Yang J, He ZS, Zhang ZR, Li DZ, Yang JB. 2018. Genome skimming herbarium specimens for DNA barcoding and phylogenomics. Plant Methods. 14(1):43. doi:10.1186/s13007-018-0300-0.
- Zhang Q, Antonelli A, Feild TS, Kong HZ. 2011. Revisiting taxonomy, morphological evolution, and fossil calibration strategies in Chloranthaceae. J Syst Evol. 49(4):315–329. doi:10.1111/j.1759-6831.2011.00129.x.
- Zhang Q, Feild TS, Antonelli A. 2015. Assessing the impact of phylogenetic incongruence on taxonomy, floral evolution, biogeographical history, and phylogenetic diversity. Am J Bot. 102(4):566–580. doi:10.3732/ajb.1400527.