Abstract
Tricyrtis xianjuensis Li, Chen & Ma 2014 is a rare and endangered species endemic to Zhejiang province, with fewer than 200 individuals in the wild. In our present study, the complete chloroplast genome of T. xianjuensis was assembled by using high-throughput sequencing data, and its genomic features were described and comparative genomic analyses within Liliaceae family were performed. The complete chloroplast genome of T. xianjuensis was 155,748 bp in length, exhibiting a GC content of 37.3%. This genome structure contained two inverted repeats (IRs), as well as a small single-copy (SSC) and a large single-copy (LSC) region. The IR region measured 26,371 bp, while the SSC and LSC regions were 17,729 bp and 85,277 bp in length, respectively. A total of 137 genes were identified, including 85 protein-coding genes, 38 tRNA genes, eight rRNA genes, and six pseudogenes. Phylogenic analysis revealed T. xianjuensis shared a clade with T. formosana Baker 1879 and T. macropoda Miq. 1867, with a support rate of 100%. The assembly and analysis of T. xianjuensis chloroplast genome provided an insight into further studies on the conservation genetics of this endangered species.
Introduction
Chloroplasts are organelles that perform diverse functions, including photosynthesis, as well as biosynthesis of phytohormones, starch, lipids, and vitamins (Chen et al. Citation2018). The chloroplast genome contains genes necessary for synthesis of proteins involved in photosynthesis and metabolic processes, and they are widely used in plant diversity, evolution, and species identification (Daniell et al. Citation2016). The genus Tricyrtis, classified within family Liliaceae, is a small-sized group comprising approximately 18 species, and they are predominantly distributed from Himalayas to East Asia (Wu et al. Citation2000; Sophia and Jury Citation2012). There are about 10 Tricyrtis species distributed in China, including T. macropoda Miq 1867, T. pilosa Wall. 1826, and T. xianjuensis Li, Chen & Ma 2014. T. macropoda and T. pilosa are widely distributed across many provinces in China (Wu et al. Citation2000), while T. xianjuensis has a limited distribution range and is only found in Shenxianju Mountain, Xianju County, Zhejiang Province (2014). By searching NCBI database, we found two species, T. macropoda and T. formosana Baker 1879, for which the chloroplast genomes have been assembled. Currently, there is only one published report available regarding the research on T. xianjuensis (Ma et al. Citation2014). To clarify the sequence characteristics, gene composition, and taxonomic relationship, the chloroplast genome of T. xianjuensis was assembled, and a phylogenetic tree was constructed, which could serve as a foundation for further investigation on conservation genetics.
Materials and methods
Plant sampling
Leaves were collected from Shenxianju Mountain (28°41′19″, 120°37′8″, 865 m), Xianju County, Zhejiang, China (). Leaves were kept in a foam box filled with ice packs and taken to our laboratory. A voucher specimen CHS20210038 was deposited in Molecular Biology Innovation Laboratory at Taizhou University (Ming Jiang, [email protected]).
Figure 1. Tricyrtis xianjuensis Li, Chen & Ma 2014. (A) Habitat; (B) seedling; (C) flower. All the photos were taken by Ming Jiang. Tricyrtis xianjuensis is a perennial herb up to 70 cm, with short rhizomes, ascending stems, alternate leaves, and yellow flowers. Flowering period of this species occurs between September and early October, while fruiting in October.
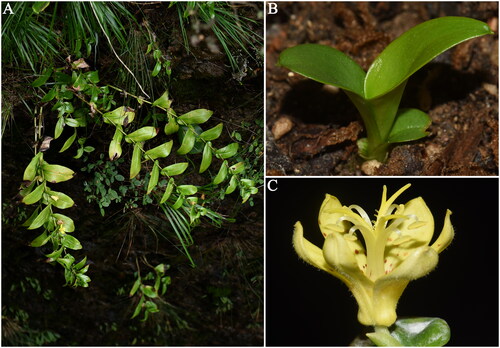
DNA isolation, sequencing, assembling, and annotation
To isolate genomic DNA, we utilized the CTAB method (Doyle and Doyle Citation1987). We constructed a genomic DNA library and performed sequencing by the Illumina Hiseq X Ten System. Low-quality sequences were filtered using NGSQCToolkit v2.3.3 (Patel and Jain Citation2012). The chloroplast genome was assembled by NOVOPlasty with default parameters (Dierckxsens et al. Citation2017), and matK of T. macropoda (KU303884) was utilized as a seed. A map of T. xianjuensis chloroplast genome was generated using CPGview (http://www.1kmpg.cn/cpgview/) (Liu et al. Citation2023). The simple sequence repeats (SSRs) were identified using MISA software (Allen et al. Citation2011).
Phylogenetic analysis
A total of 25 chloroplast genomes from species within Liliaceae family were retrieved from NCBI, and the sequences of Croomia japonica Miq. 1865 and C. pauciflora (Nutt.) Torr. 1840 were also downloaded as the outgroup. The genomes were aligned using MAFFT v7.450 (Katoh and Standley Citation2013), and a phylogenetic tree was constructed using the maximum-likelihood method by PhyML 3.1 (Guindon et al. Citation2010), with the best-fit substitution model of GTR + R.
Results
The high-throughput sequencing resulted in 3.79 G raw data, consisting of 12,618,427 reads, and the average sequencing depth was 853.83 (Figure S1). After removing low-quality reads, the clean data accounted for 3.75 G, comprising 12,484,278 high-quality reads. The complete chloroplast genome of T. xianjuensis was 155,748 bp in length, with a GC content of 37.3%. The genome structure included two inverted repeats (IRs), a small single-copy (SSC) region, and a large single-copy (LSC) region. The IR was 26,371 bp in length, while the SSC and LSC were 17,729 bp and 85,277 bp, respectively. The sequence of T. xianjuensis has been submitted to NCBI with the accession number of OR526424.
Gene annotation revealed a total of 137 genes on T. xianjuensis chloroplast genome, which consisted of 85 protein-coding genes, 38 tRNAs, eight rRNAs, and six pseudogenes (). A small proportion of genes exhibited two copies, with 11 protein-coding genes (ndhB, rpl2, rpl23, rpoC1, rps7, rps12, rps19, ycf1, ycf2, ycf15, and ycf68) and eight tRNAs (trnA-UGC, trnH-GUG, trnI-CAU, trnI-GAU, trnL-CAA, trnN-GUU, trnR-ACG, and trnV-GAC). Thirteen genes possessed one or two introns. Among them, rps16, atpF, rpoC1, petB, petD, rpl16, rpl2, ndhB (two copies), and rpl2 (two copies) contained one intron each, while ycf3 and clpP held two introns each (Figure S2). In addition to the cis-splicing genes mentioned above, there was also one trans-splicing gene, rps12, present in the genome (Figure S3). Moreover, a total of six pseudogenes were identified, including ψycf15 (two copies), ψycf68 (two copies), ψycf1, and ψrps19. A total of 33 SSRs were predicted, and all were found to be mononucleotides.
Figure 2. The chloroplast genome map of Tricyrtis xianjuensis. The map encompasses six tracks that depict various features of the genome. Starting from the center, the first track displays dispersed repeats, consisting of both direct and palindromic repeats, which are delineated by red and green arcs. The second track highlights long tandem repeats represented by short blue bars. In the third track, short tandem repeats or microsatellite sequences are illustrated as colored bars. Each color corresponds to a specific type of repeat, and accompanying descriptions provide valuable information about the characteristics of each repeat type. The colors and their respective repeat types are as follows: black: c (complex repeat); green: p1 (repeat unit size = 1); yellow: p2 (repeat unit size = 2); purple: p3 (repeat unit size = 3); blue: p4 (repeat unit size = 4); orange: p5 (repeat unit size = 5); red: p6 (repeat unit size = 6). The chloroplast genome contains an LSC region, an SSC region, and two IR regions, and they are shown on the fourth track. The GC content along the genome is shown on the fifth track. Genes within the genome visualization are meticulously color-coded based on their functional classification. The transcription directions of the inner genes are represented in a clockwise manner, while the outer genes are shown in an anticlockwise orientation. To assist with interpretation, the key for gene functional classification is provided in the bottom left corner of the visualization.
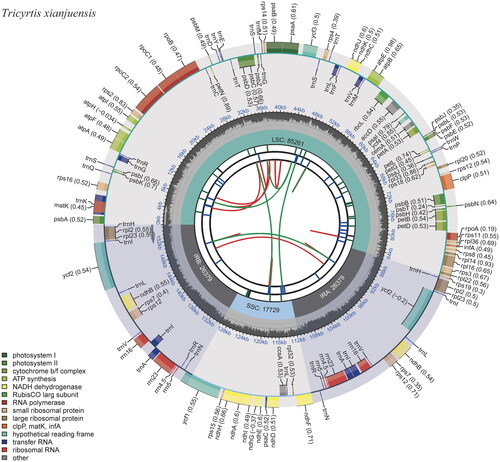
A phylogenetic tree was constructed using full length chloroplast genome sequences. The results showed that the 29 sequences could be divided into six major groups, namely I, II, III, IV, V, and VI. The phylogenetic analysis indicated that species from the same genus clustered together, such as Smilax (I), Ripogonum (II), Tricyrtis (III), and Lilium (V). However, there existed an exception where Prosartes lanuginosa (Michx.) D.Don 1839 formed a group (IV) with Streptopus ovalis (Ohwi) F.T.Wang & Y.C.Tang 1978 and S. obtusatus Fassett 1935 (). The results revealed a close relationship between the three Tricyrtis species; T. macropoda and T. formosana first clustered together, then formed a group with T. xianjuensis, supported by a 100% confidence rate.
Figure 3. The maximum-likelihood tree based on complete chloroplast genome sequences of Tricyrtis xianjuensis and 25 species of family Liliaceae, with Croomia japonica and C. pauciflora as the outgroup species. The numbers next to the nodes are bootstrap support values. NCBI accession numbers of each genome are shown in the figure. The following sequences were used: Croomia pauciflora MH191380 (Lu et al. Citation2018), C. japonica MH177871 (Lu et al. Citation2018), Smilax moranensis OL693684 (Ji et al. Citation2022), S. microphylla NC_056390 (Wu et al. Citation2021), S. weniae NC_067604 (Feng et al. Citation2022), S. nipponica NC_049024, S. riparia OL351263, S. glabra MZ442610, S. glycophylla NC_049023 (Do et al. Citation2020), Ripogonum scandens NC_049021 (Do et al. Citation2020), R. fawcettianum NC_081473, R. elseyanum NC_081472, R. discolor NC_081471, Tricyrtis formosana NC_058298, T. macropoda NC_040223 (Wang et al. Citation2018), Prosartes lanuginose NC_058296, Streptopus ovalis MT261171 (Do et al. Citation2020), S. obtusatus NC_058297, Lilium davidii NC_060550 (Li et al. Citation2021), L. leichtlinii var. maximowiczii MK753242, L. pensylvanicum NC_043876 (Ramekar et al. Citation2019), L. lancifolium MW465411, L. concolor NC_058994, L. callosum MT261160 (Do et al. Citation2020), L. distichum MK493296, L. hansonii MT261163 (Do et al. Citation2020), and L. tsingtauense KU230438 (Song et al. Citation2016).
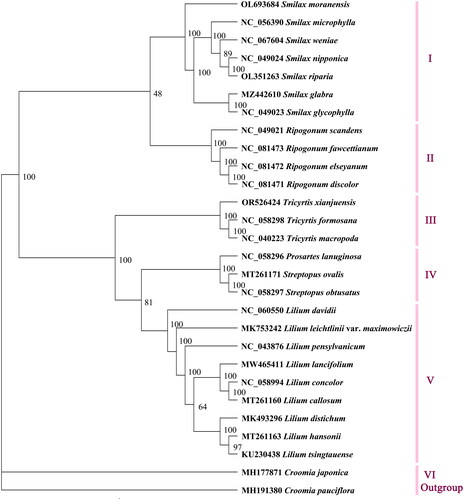
Discussion and conclusions
Chloroplast genomes are highly conserved in both structure and gene content, and they are regarded as useful molecular tools for genetic diversity and evolution studies (Perumal et al. Citation2021). The chloroplast genome of T. xianjuensis was not assembled and analyzed, which hinders further investigation into its conservation genetics. It was observed that the length of the chloroplast genomes in Tricyrtis is highly conserved. The full length of T. formosana (NC_058298.1) is 156,018 bp, only 270 bp longer than that of T. xianjuensis. Similarly, the complete length of T. macropoda (NC_040223.1) (Wang et al. Citation2018) is 155,778 bp, merely 22 bp longer than that of T. xianjuensis. However, compared to matK gene and rps16 intron, which were previously used for phylogenetic and evolutionary analyses of Tricyrtis species, the chloroplast genome offers a greater number of variable sites (Sophia and Jury Citation2012).
T. xianjuensis demonstrated a duplication event involving the rps19 gene, with one copy being a pseudogene. The pseudogene was characterized by a 3′ end deletion, triggered by the IRb/LSC boundary located within this copy. Pseudogenization of rps19 caused by the IRb/LSC boundary has been documented in multiple plant species, including Dianthus superbus var. longicalyncinus (Maxim.) Will. 1899, Decaisnea insignis (Griff.) Hook. fil. & Thomson 1854, and Cerasus humilis (Bunge) S. Ya. Sokolov 1954 (Raman and Park Citation2015; Li et al. Citation2017; Mu et al. Citation2018). The ycf68 gene was present in two copies in T. xianjuensis. However, both copies were pseudogenes with several internal stop codons within their sequences. Similar occurrences have been observed in other plants such as Angiopteris evecta (G.Forst.) Hoffm. 1793, several Mammillaria species, and Zephyranthes phycelloides (Herb.) Nic. García 2019 (Roper et al. Citation2007; Solórzano et al. Citation2019; Contreras-Díaz et al. Citation2022).
In conclusion, the complete chloroplast genome of T. xianjuensis was 155,748 bp with a total of 137 genes, and phylogenetic analysis revealed T. xianjuensis shared a clade with T. formosana and T. macropoda. Assembly and sequence analysis of the complete chloroplast genome sequence of T. xianjuensis provided insights into population genetics and biodiversity studies for this rare species in the future.
Author contributions
Ming Jiang and Junfeng Wang were responsible for the conception and design of the study. Huijuan Zhang and Ming Jiang wrote the paper. Leqin Huang, Zhenyu Lu, Junfeng Wang, and Honghua Bao isolated DNA and performed sequence analysis. Ming Jiang and Huijuan Zhang assembled and annotated the chloroplast genome. All the authors were involved in the analysis and interpretation of the data, revising the manuscript critically for intellectual content, and gave the final approval of the version to be published. All authors have read and approved the final manuscript.
Ethics statement
Our manuscript does not contain any studies with human participants or animals performed by any of the authors. The study has been carried out in accordance with guidelines provided by our institutions and national regulations. We strictly comply with the regulations of the People’s Republic of China on the Protection of Wild Plants, the International Union for Conservation of Nature, the Convention on Biological Diversity and the Convention on International Trade in Endangered Species of Wild Fauna and Flora. The leaves were collected in a location not designated as a protected area in China.
Supplemental Material
Download MS Word (351.8 KB)Disclosure statement
No potential conflict of interest was reported by the author(s).
Data availability statement
The data that support the findings of this study are openly available in GenBank of NCBI at https://www.ncbi.nlm.nih.gov/nuccore/ OR526424. The associated BioProject, SRA, and Bio-Sample numbers are PRJNA1025334, SRR26334644, and SAMN37721183, respectively.
Additional information
Funding
References
- Allen JF, de Paula WB, Puthiyaveetil S, Nield J. 2011. A structural phylogenetic map for chloroplast photosynthesis. Trends Plant Sci. 16(12):645–655. doi:10.1016/j.tplants.2011.10.004.
- Chen Y, Zhou B, Li J, Tang H, Tang J, Yang Z. 2018. Formation and change of chloroplast-located plant metabolites in response to light conditions. Int J Mol Sci. 19(3):654. doi:10.3390/ijms19030654.
- Contreras-Díaz R, Arias-Aburto M, van den Brink L. 2022. Characterization of the complete chloroplast genome of Zephyranthes phycelloides (Amaryllidaceae, Tribe Hippeastreae) from Atacama region of Chile. Saudi J Biol Sci. 29(1):650–659. doi:10.1016/j.sjbs.2021.10.035.
- Daniell H, Lin CS, Yu M, Chang WJ. 2016. Chloroplast genomes: diversity, evolution, and applications in genetic engineering. Genome Biol. 17(1):134. doi:10.1186/s13059-016-1004-2.
- Dierckxsens N, Mardulyn P, Smits G. 2017. NOVOPlasty: de novo assembly of organelle genomes from whole genome data. Nucleic Acids Res. 45(4):e18. doi:10.1093/nar/gkw955.
- Do HDK, Kim C, Chase MW, Kim JH. 2020. Implications of plastome evolution in the true lilies (monocot order Liliales). Mol Phylogenet Evol. 148:106818. doi:10.1016/j.ympev.2020.106818.
- Doyle JJ, Doyle JL. 1987. A rapid DNA isolation procedure for small quantities of fresh leaf tissue. Phytochem Bull. 19:11–15.
- Feng JY, Jin XJ, Zhang SL, Yang JW, Fei SP, Huang YS, Liu Y, Qi ZC, Li P. 2022. Smilax weniae, a new species of Smilacaceae from limestone areas bordering Guizhou and Guangxi, China. Plants. 11(8):1032. doi:10.3390/plants11081032.
- Guindon S, Dufayard JF, Lefort V, Anisimova M, Hordijk W, Gascuel O. 2010. New algorithms and methods to estimate maximum-likelihood phylogenies: assessing the performance of PhyML 3.0. Syst Biol. 59(3):307–321. doi:10.1093/sysbio/syq010.
- Ji B, Pei L, Cui N. 2022. The complete chloroplast genome and phylogenetic analysis of Smilax moranensis (Liliales: Smilacaceae). Mitochondrial DNA B Resour. 7(7):1206–1207. doi:10.1080/23802359.2022.2091960.
- Katoh K, Standley DM. 2013. MAFFT multiple sequence alignment software version 7: improvements in performance and usability. Mol Biol Evol. 30(4):772–780. doi:10.1093/molbev/mst010.
- Li B, Lin F, Huang P, Guo W, Zheng Y. 2017. Complete chloroplast genome sequence of Decaisnea insignis: genome organization, genomic resources and comparative analysis. Sci Rep. 7(1):10073. doi:10.1038/s41598-017-10409-8.
- Li J, Cai J, Qin HH, Price M, Zhang Z, Yu Y, Xie DF, He XJ, Zhou SD, Gao XF. 2021. Phylogeny, age, and evolution of tribe Lilieae (Liliaceae) based on whole plastid genomes. Front Plant Sci. 12:699226. doi:10.3389/fpls.2021.699226.
- Liu SY, Ni Y, Li JL, Zhang XY, Yang HY, Chen HM, Liu C. 2023. CPGview: a package for visualizing detailed chloroplast genome structures. Mol Ecol Resour. 23(3):694–704. doi:10.1111/1755-0998.13729.
- Lu Q, Ye W, Lu R, Xu W, Qiu Y. 2018. Phylogenomic and comparative analyses of complete plastomes of Croomia and Stemona (Stemonaceae). Int J Mol Sci. 19(8):2383. doi:10.3390/ijms19082383.
- Ma DD, Chen ZH, Li GY, Zhu ZM, Zhang RZ, Peng JL. 2014. Tricyrtis xianjuensis (Liliaceae), a new species from eastern China. Ann Bot Fennici. 51:217–221.
- Mu X, Wang P, Du J, Gao YG, Zhang J. 2018. The chloroplast genome of Cerasus humilis: genomic characterization and phylogenetic analysis. PLOS One. 13(4):e0196473. doi:10.1371/journal.pone.0196473.
- Patel RK, Jain M. 2012. NGS QC Toolkit: a toolkit for quality control of next generation sequencing data. PLOS One. 7(2):e30619. doi:10.1371/journal.pone.0030619.
- Perumal S, Waminal NE, Lee J, Koo HJ, Choi B, Park JY, Ahn K, Yang TJ. 2021. Nuclear and chloroplast genome diversity revealed by low-coverage whole-genome shotgun sequence in 44 Brassica oleracea breeding lines. Hortic Plant J. 7(6):539–551. doi:10.1016/j.hpj.2021.02.004.
- Raman G, Park S. 2015. Analysis of the complete chloroplast genome of a medicinal plant, Dianthus superbus var. longicalyncinus, from a comparative genomics perspective. PLOS One. 10(10):e0141329. doi:10.1371/journal.pone.0141329.
- Ramekar RV, Park KC, Kwak M, Choi IY. 2019. The complete chloroplast genome of a rare species in Korea, Lilium dauricum Ker Gawl. Mitochondrial DNA B Resour. 4(2):3591–3592. doi:10.1080/23802359.2019.1676174.
- Roper JM, Hansen SK, Wolf PG, Karol KG, Mandoli DF, Everett KDE, Kuehl J, Boore JL. 2007. The complete plastid genome sequence of Angiopteris evecta (G. Forst.) Hoffin. (Marattiaceae). Am Fern J. 97(2):95–106. doi:10.1640/0002-8444(2007)97[95:TCPGSO]2.0.CO;2.
- Solórzano S, Chincoya DA, Sanchez-Flores A, Estrada K, Díaz-Velásquez CE, González-Rodríguez A, Vaca-Paniagua F, Dávila P, Arias S. 2019. De novo assembly discovered novel structures in genome of plastids and revealed divergent inverted repeats in Mammillaria (Cactaceae, Caryophyllales). Plants. 8(10):392. doi:10.3390/plants8100392.
- Song JH, Yoon CY, Do HDK, Lee WB, Kim JH. 2016. The complete chloroplast genome sequence of Lilium tsingtauense Gilg (sect. Martagon, Liliaceae). Mitochondrial DNA B Resour. 1(1):318–320. doi:10.1080/23802359.2016.1172043.
- Sophia WPH, Jury SL. 2012. Phylogeny and molecular evolution of Tricyrtis (Liliaceae s.l.) inferred from plastid DNA matK spacer nucleotide sequences. J Plant Stud. 1(2):1–10.
- Wang RH, Gao J, Wu X, Li MD, Shen C, Wu JJ, Yu WD, Liu JL, Qi ZC, Liang ZS. 2018. Characterization of the complete chloroplast genome of toad lily Tricyrtis macropoda (Liliaceae). Mitochondrial DNA B Resour. 3(1):145–146. doi:10.1080/23802359.2018.1431069.
- Wu F, Liang D, Yang J. 2021. The complete chloroplast genome sequence of Smilax microphylla CH Wright. Mitochondrial DNA B Resour. 6(8):2235–2236. doi:10.1080/23802359.2021.1947914.
- Wu ZY, Raven PH, Hong DY. 2000. Flora of China. Vol. 24. Beijing/St. Louis: Science Press/Missouri Botanical Garden Press.