Abstract
Pinus bhutanica is a critically endangered conifer and occurs only in central Bhutan, northwestern Yunnan and southeastern Xizang in China. In this study, the complete chloroplast genome of Pinus bhutanica was first assembled based on next-generation sequencing. The genome sequence was 116,919 bp in length with an overall GC content of 38.75%. A total of 106 functional genes were detected in the genome, including 72 protein-coding genes (PCGs), 30 transfer RNA (tRNA) genes, and four ribosomal RNA (rRNA) genes. The phylogenetic tree reconstructed by 12 chloroplast genomes revealed that P. bhutanica is most closely related to Pinus wangii in subsection Strobus of Pinus.
Introduction
Pinus bhutanica Grierson, D. G. Long & C. N. Page 1980 belongs to the subsection Strobus in Pinus (Gernandt et al. Citation2005). This species has an extremely narrow distribution and occurs only in central Bhutan, northwestern Yunnan, and southeastern Xizang in China (Farjon Citation1998). Although phylogenetic analyses have reconstructed a fully resolved phylogeny of the species in the subsection Strobus based on thousands of nuclear genes (Liu et al. Citation2022), the interspecific relationships of these species, and including P. bhutanica, are still unclear. The chloroplast genome is maternally inherited in coniferous trees (Mogensen Citation1996), making it an important resource for exploring the phylogenetic relationships of these species (Kress et al. Citation2005). However, a complete chloroplast genome of P. bhutanica is lacking. In this study, we sequenced and analyzed the complete chloroplast genome sequence of P. bhutanica, and inferred the phylogenetic relationships of this species in the subsection Strobus.
Materials and methods
Fresh leaves of P. bhutanica were collected from a single individual living in Linzhi, Xizang, China (geographic coordinates: 29°13′27″ N, 95°11′3″ E) (, Figure S1). Total genomic DNA was extracted from approximately 20 mg of dried leaves using a modified CTAB procedure (Doyle and Doyle Citation1987). A voucher specimen (2021-PB-1A) and an associated DNA sample were stored in the Herbarium of Jiangxi Agricultural University, Nanchang, China. Whole-genome sequencing was conducted on the DNBseq platform (BGI, Shenzhen, China) with paired-end reads of 150 bp. A total of 6.4 Gb clean data (∼20 million reads) with a Q20 of 97.87% were obtained and subsequently used for chloroplast genome assembly. The assembly was performed using NOVOPlasty 4.3.1 (Dierckxsens et al. Citation2016), and annotation of the assembled genome was performed by Plastid Genome Annotator (PGA) (Qu et al. Citation2019) with default parameters and the closely related species Pinus koraiensis Siebold et Zuccarini (NC_0046772.2) as the reference. The detailed chloroplast genome structure was visualized using CPGView (Liu et al. Citation2023). To verify the accuracy of the assembly, we mapped clean reads to the assembled chloroplast genome to assess the depth of coverage using a python script (Ni et al. Citation2023).
Figure 1. The reference image of Pinus bhutanica living in Linzhi, Xizang, China (geographic coordinates: 29°13′27″ N, 95°11′3″ E; photography by Yixuan Kou and Jing Wang).
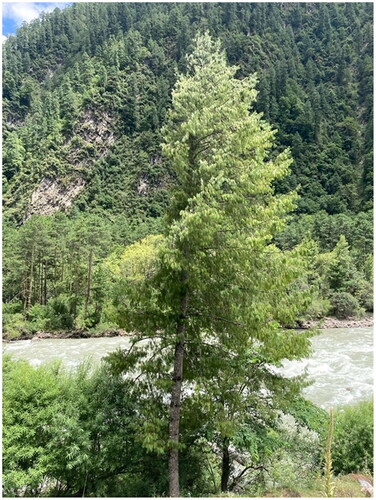
The phylogenetic relationships of P. bhutanica in subsection Strobus were explored based on chloroplast genome datasets. The chloroplast genome sequences of 10 species in subsection Strobus were downloaded and aligned with the genome of P. bhutanica using MAFFT v7.310 (Katoh and Standley Citation2013). Pinus krempfii Lecomte was used as the outgroup. A maximum-likelihood (ML) tree was constructed by FastTree 2.1.11 (Price et al. Citation2009) with the GTR substitution model.
Results
The complete chloroplast genome of P. bhutanica was deposited in the GenBank database under accession number OP747469. It was circular and 116,919 bp in length with an overall GC content of 38.75% and an average coverage of 312.62× (, Figure S2). A total of 106 functional genes were annotated in the genome, including 72 protein-coding genes (PCGs), 30 transfer RNA (tRNA) genes, and four ribosomal RNA (rRNA) genes. Most of these genes occurred as a single copy and had no introns, except that psbA, trnR-ACG, trnS-GCU, and trnT-GGU had two copies, trnI-GAU had three copies, and 12 genes (atpF, petB, petD, rpl16, rpl2, rpoC1, trnA-UGC, trnG-UCC, trnI-GAU, trnK-UUU, trnL-UAA, and trnV-UAC) had one intron and two genes (rps12 and pafI) had two introns (). In addition, seven cis-splicing genes (atpF, rpoC1, petB, petD, rpl16, rpl2, and pafI) and one trans-splicing gene (rps12) were detected (Figure S3). The phylogenetic analyses showed that all species in subsection Strobus had high support, and P. bhutanica was most closely related to P. wangii Hu et Cheng ().
Figure 2. Genomic map of overall features of Pinus bhutanica chloroplast genome, generated by CPGView (Liu et al. Citation2023). The map contains six tracks by default. From the center outward, the first track shows the dispersed repeats. The dispersed repeats consist of direct and palindromic repeats, connected with red and green arcs. The second track shows the long tandem repeats as short blue bars. The third track shows the short tandem repeats or microsatellite sequences as short bars with different colors. The genome length and the GC content along the genome are shown on the fourth and fifth track, respectively. The genes are shown on the sixth track. Genes are color-coded by their functional classification.
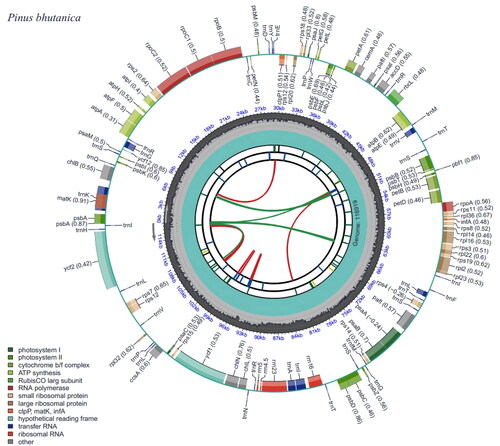
Figure 3. Maximum-likelihood (ML) tree inferred by FastTree with the GTR substitution model based on the complete chloroplast genome sequences of P. bhutanica and 10 other species in subsection Strobus. The numbers on the nodes represent the support values. The following sequences were used: Pinus wangii MH167924.1 (Yang et al. Citation2018), Pinus dabeshanensis KX255674.1 (Duan et al. Citation2016), Pinus armandii MT644189.1 (Jia et al. Citation2020), Pinus morrisonicola NC_039616.1 (Zeb et al. Citation2020), Pinus pumila NC_041108.1 (Zeb et al. Citation2019), Pinus cembra MN536531.1 (Schott et al. Citation2019), Pinus sibirica NC_028552.2 (Baturina et al. Citation2019), Pinus koraiensis NC_004677.2 (available in the GenBank of NCBI), Pinus lambertiana MH612865.1 (Gernandt et al. Citation2018), Pinus strobus NC_026302.1 (Zhu et al. Citation2016), and Pinus krempfii NC_011155.4 (Cronn et al. Citation2008).
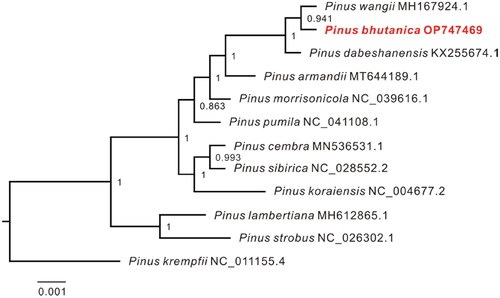
Discussion and conclusions
The chloroplast genome of Pinus bhutanica provides valuable information for understanding chloroplast genome evolution and phylogenetic inference in subsection Strobus in genus Pinus. In this study, the complete chloroplast genome of P. bhutanica was first reported and found to exhibit a total length of 116,919 bp. A total of 106 functional genes were annotated in the genome, including 72 PCGs, 30 tRNA genes, and four rRNA genes. The genome size and gene content of Pinus bhutanica is not significantly different from those of most chloroplast genomes in the subsection Strobus (Zeb et al. Citation2020), indicating that the genome evolution was relatively conservative in the subsection. The phylogenetic analysis revealed that P. bhutanica is most closely related to Pinus wangii in subsection Strobus. However, the discordance among the phylogenies from different publications was found for P. bhutanica and some other taxa in the subsection (Duan et al. Citation2016; Yang et al. Citation2018; Baturina et al. Citation2019; Schott et al. Citation2019; Zeb et al. Citation2019, Citation2020), suggesting that incomplete lineage sorting of chloroplast genome and/or introgressive hybridization among species might occur in the subsection. Therefore, more complete chloroplast genomes from subsection Strobus are necessary for further research on phylogenetic relationships in the subsection.
Author contributions
Shiqi Lv, Yixuan Kou, and Jing Wang conceived and designed the paper; Shiqi Lv, Jiao Chen, Bingbing Li, Taotao Fu, Mingliang Song, Pengtao Zhang, and Kang Liu performed the experiments and analyzed the data; Yixuan Kou and Jing Wang drafted the paper. All authors read and approved the final manuscript.
Ethics statement
The study was approved by the institutional review board of Jiangxi Agricultural University, Nanchang, China. The collection of plant materials was conducted in accordance with guidelines provided by Jiangxi Agricultural University and Xizang province regulations.
Supplemental Material
Download PDF (3.3 MB)Supplemental Material
Download PDF (33.1 KB)Disclosure statement
The authors have no conflicts of interest to declare.
Data availability statement
The genome sequence data that support the findings of this study are openly available in the GenBank of NCBI (https://www.ncbi.nlm.nih.gov/) under the accession number OP747469. The associated BioProject, SRA, and Bio-Sample numbers are PRJNA898140, SRR22188315, and SAMN31601598, respectively. A specimen of Pinus bhutanica was deposited at Jiangxi Agricultural University (https://www.jxau.edu.cn; contact person and email: Jing Wang, [email protected]) under the voucher number 2021-PB-1A.
Additional information
Funding
References
- Baturina OA, Tupikin AE, Goroshkevich SN, Petrova EA, Kabilov MR. 2019. The complete chloroplast genome sequences of Pinus sibirica Du Tour. Mitochondrial DNA B. 4(1):286–287. doi:10.1080/23802359.2018.1542983.
- Cronn R, Liston A, Parks M, Gernandt DS, Shen R, Mockler T. 2008. Multiplex sequencing of plant chloroplast genomes using Solexa sequencing-by-synthesis technology. Nucleic Acids Res. 36(19):e122. doi:10.1093/nar/gkn502.
- Dierckxsens N, Mardulyn P, Smits G. 2016. NOVOPlasty: de novo assembly of organelle genomes from whole genome data. Nucleic Acids Res. 45(4):e18. doi:10.1093/nar/gkw955.
- Doyle JJ, Doyle JL. 1987. A rapid DNA isolation procedure for small quantities of fresh leaf tissue. Phytochem Bull. 19(1):11–15.
- Duan RY, Yang LM, Lv T, Wu GL, Huang MY. 2016. The complete chloroplast genome sequence of Pinus dabeshanensis. Conserv Genet Resour. 8(4):395–397. doi:10.1007/s12686-016-0567-2.
- Farjon A. 1998. World checklist and bibliography of conifers. Kew, Richmond: Royal Botanical Gardens.
- Gernandt DS, López GG, García SO, Liston A. 2005. Phylogeny and classification of Pinus. Taxon. 54(1):29–42. doi:10.2307/25065300.
- Gernandt DS, Reséndiz Arias C, Terrazas T, Aguirre Dugua X, Willyard A. 2018. Incorporating fossils into the Pinaceae tree of life. Am J Bot. 105(8):1329–1344. doi:10.1002/ajb2.1139.
- Jia Y, Milne RI, Zhu J, Gao LM, Zhu GF, Zhao GF, Liu J, Li ZH. 2020. Evolutionary legacy of a forest plantation tree species (Pinus armandii): implications for widespread afforestation. Evol Appl. 13(10):2646–2662. doi:10.1111/eva.13064.
- Katoh K, Standley DM. 2013. MAFFT multiple sequence alignment software version 7: improvements in performance and usability. Mol Biol Evol. 30(4):772–780. doi:10.1093/molbev/mst010.
- Kress WJ, Wurdack KJ, Zimmer EA, Weigt LA, Janzen DH. 2005. Use of DNA barcodes to identify flowering plants. Proc Natl Acad Sci U S A. 102(23):8369–8374. doi:10.1073/pnas.0503123102.
- Liu S, Ni Y, Li J, Zhang X, Yang H, Chen H, Liu C. 2023. CPGView: a package for visualizing detailed chloroplast genome structures. Mol Ecol Resour. 23(3):694–704. doi:10.1111/1755-0998.13729.
- Liu YY, Jin WT, Wei XX, Wang XQ. 2022. Phylotranscriptomics reveals the evolutionary history of subtropical East Asian white pines: further insights into gymnosperm diversification. Mol Phylogenet Evol. 168:107403. doi:10.1016/j.ympev.2022.107403.
- Mogensen HL. 1996. The hows and whys of cytoplasmic inheritance in seed plants. Am J Bot. 83(3):383–404. doi:10.2307/2446172.
- Ni Y, Li J, Zhang C, Liu C. 2023. Generating sequencing depth and coverage map for organelle genomes. doi:10.17504/protocols.io.4r3l27jkxg1y/v1.
- Price MN, Dehal PS, Arkin AP. 2009. FastTree: computing large minimum-evolution trees with profiles instead of a distance matrix. Mol Biol Evol. 26(7):1641–1650. doi:10.1093/molbev/msp077.
- Qu XJ, Moore MJ, Li DZ, Yi TS. 2019. PGA: a software package for rapid, accurate, and flexible batch annotation of plastomes. Plant Methods. 15(1):50. doi:10.1186/s13007-019-0435-7.
- Schott T, Schroeder H, Schöning-Stierand K, Kersten B. 2019. The complete chloroplast genome sequence of Pinus cembra L. (Pinaceae). Mitochondrial DNA B Resour. 4(2):4202–4203. doi:10.1080/23802359.2019.1693297.
- Yang MQ, Du Y, Ling LZ. 2018. Characterization of the complete chloroplast genome of Pinus wangii (Pinaceae), an endangered and endemic species in China. Mitochondrial DNA B Resour. 3(2):1195–1197. doi:10.1080/23802359.2018.1519381.
- Zeb U, Dong WL, Zhang TT, Wang RN, Shahzad K, Ma XF, Li ZH. 2020. Comparative plastid genomics of Pinus species: insights into sequence variations and phylogenetic relationships. J Syst Evol. 58(2):118–132. doi:10.1111/jse.12492.
- Zeb U, Wang R, Dong P, Wang N, Zhang T, Lin C, Wang X. 2019. Characterization of the complete chloroplast genome sequence of Pinus pumila (Pinaceae). Mitochondrial DNA B. 4(1):290–291. doi:10.1080/23802359.2018.1542985.
- Zhu A, Guo W, Gupta S, Fan W, Mower JP. 2016. Evolutionary dynamics of the plastid inverted repeat: the effects of expansion, contraction, and loss on substitution rates. New Phytol. 209(4):1747–1756. doi:10.1111/nph.13743.