Abstract
Petrocosmea qinlingensis is a protected wild plant endemic in China, inhabiting low-light limestone cliffs but the complete chloroplast genome has not been reported. In this study, we first sequenced and assembled the complete chloroplast genome of P. qinlingensis. The total size of this genome was 153,865 bp, including a large single-copy (LSC) region (84,737 bp), a small single-copy (SSC) region (18,244 bp), and two inverted repeats (IRs) regions (25,442 bp). This genome encoded 111 uniquegenes, consisted of 77 protein-coding genes, four ribosomal RNA genes, and 30 transfer RNA genes. Phylogenomic analysis based on the chloroplast protein-coding genes and showed that the genus Petrocosmea was the closest relative to Raphiocarpus. Our results will support further phylogeographic, population genetic studies of this species.
Introduction
Petrocosmea qinlingensis Wen-tsai Wang Citation1981 (Gesneriaceae), a rosette perennial herb with gorgeous light purple flowers, was first formally described by Wen-tsai Wang in 1981 (Wang Citation1981). It is classified under the Gesneriaceae, and it is also the northernmost species and the only species of Petrocosmea listed on the National Key Protected Wild Plants (category II, https://www.gov.cn/gongbao/content/2000/content_60072.htm) (Lu et al. Citation2021). Presently, its population is limited to a mere 1000 individuals, with an extremely narrow distribution in the valley around Chadian Town, Mian County, Shaanxi Province (Jiang et al. Citation2019). In contrast to other Petrocosmea species, P. qinlingensis is typically habitant in shaded environments beneath forest canopies on limestone hills and thrives on damp granite hills. This striking difference in habitat preference offers an invaluable opportunity for in-depth investigation, which carries profound implications for understanding the broader field of the evolutionary trajectory and migration route of Petrocosmea, as well as the composition, history, and dynamics of the Qinling Flora.
Chloroplasts are the primary site for photosynthesis in green plants, converting light energy into chemical energy (Sierra et al. Citation2023). They serve as the receivers of light and are one of the organelles that retain the genome within plant cells. The chloroplast genome of angiosperms is relatively conserved, typically ranging from 120 to 150 kb circular molecules in size (Tonti-Filippini et al. Citation2017). It consists of a larger single-copy region (LSC), a small single-copy region (SSC), and a pair of inverted repeats (IR), forming a quadripartite structure (Tonti-Filippini et al. Citation2017). In contrast to the nuclear genome, chloroplast genomes are generally inherited uniparentally (usually maternally), leading to infrequent homologous recombination (Mogensen Citation1996; Camus et al. Citation2022). Moreover, they exist in high-copy within the cell, making their assembly relatively straightforward (Freudenthal et al. Citation2020). Therefore, chloroplast genomes have been widely used to reconstruct the phylogenetic relationship. In addition, the chloroplast genome encodes a large number of protein complexes involved in photosynthesis (Forsythe et al. Citation2019), and is also an ideal object for exploring the adaptation to different light intensities.
Therefore, we assembled the chloroplast genome of P. qinlingensis, a species distributed in a shady environment, to investigate the phylogenetic position of this species and improve the protection of this endangered species.
Materials and methods
In this study, the leaves were collected from Chadian Town, Mian County, Shaanxi Province (36.6565° N, 117.1263° E) (), the total DNA was extracted by the modified CTAB method (Doyle and Doyle Citation1987), and the next-generation sequencing was performed on the Illumina HiSeq4000 platform (Novogene lnc.). The voucher specimen was deposited at Qilu Normal University (Chao-Qun Li, [email protected]) under the voucher number LCQ20210502011. A total of 31,810,878 raw reads (PE 150) were obtained, and there were still 31,481,168 high-quality reads after quality control (98.96% efficiency). We performed de novo assembly of high-quality reads using SPAdes v3.15.3, followed by manual adjustments using Bandage v0.8.1 (Bankevich et al. Citation2012; Wick et al. Citation2015). We used PGA to annotate the assembled chloroplast genome with Amborella trichopoda as a reference (GenBank: AJ506156) and checked it manually (Qu et al. Citation2019). The annotated complete chloroplast genome was finally obtained and submitted to NCBI (GenBank: NC_068657). The chloroplast genome map of P. qinlingensis was drawn by OGDRAW and CPGView (Greiner et al. Citation2019; Liu et al. Citation2023).
Figure 1. Photographs of Petrocosmea qinlingensis Wen-tsai Wang (These photos were taken by Li Chaoqun in the glasshouse of Qilu Normal University, Jinan, Shandong, China.). It is a perennial herb with a green rosette and gorgeous light purple flowers. Its corolla is lavender, the inner surface of the upper lip is slightly densely covered with white pubescence, and the lower lip length is nearly equal to the upper lip. (A) top view of the vegetative organ, and (B) front view of the flower of P. qinlingensis.
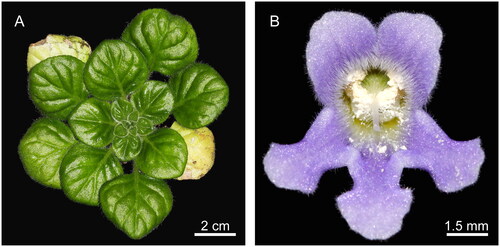
To further determine the phylogenetic position of the genus Petrocosmea, we downloaded the chloroplast genomes of representative species, covering all genera with published chloroplast genomes within Gesneriaceae. For genus with a greater number of species with published chloroplast genomes, we downloaded two representative species. Additionally, we download two species of Lamiaceae (Tectona grandis and Callicarpa americana) as outgroups. We extracted the coding regions of all protein-coding genes from P. qinlingensis and all downloaded chloroplast genomes by perl script. Then, we aligned all single gene matrix by MAFFT v7.490, filtered ambiguous blocks by Gblocks v0.91b, and concatenated by FASconCAT-G_v1.04.pl (Talavera and Castresana Citation2007; Patrick Kück and Longo Citation2014; Nakamura et al. Citation2018). Lastly, we used RAxML v8.2.12 and selected the GTRGAMMA model to reconstruct phylogenetic relationships (Stamatakis Citation2014).
Results
We obtained a total of 31,810,878 clean reads, of which 676,008 were putative chloroplast reads. The sequencing depth of the P. qinlingensis chloroplast genome was consistently uniform, with an average sequencing depth of 659.00 (SD = 65.8) (Figure S1). The chloroplast genome of P. qinlingensis was 153,865 bp in size, which is a typical quadripartite structure similar to other chloroplast genomes within the Gesneriaceae. It comprised of a large single-copy region of 84,737 bp, a small single-copy region of 18,244 bp, and a pair of inverted repeat regions of 25,442 bp (). The overall GC content of this chloroplast genome was 37.55%, and the GC content of the large single-copy region, the small single-copy region, and the inverted repeat region were 35.53%, 31.18%, and 43.21%, respectively. This genome contained 111 unique genes, including 77 protein-coding genes, four ribosomal RNA genes, and 30 transfer RNA genes. Among protein-coding genes, three genes (clpP, rps12, and ycf3) contained two introns, nine genes (atpF, ndhA, ndhB, petD, petB, rpl16, rpl2, rps16, and rpoC1) had one intron, and the other genes had no introns (Figure S2).
Figure 2. The map of the Petrocosmea qinlingensis complete chloroplast genome drawn by OGDRAW (Greiner et al. Citation2019). The outer circle is marked with annotation information. The gene name on the outside indicates clockwise transcription of the gene, while the inside indicates anticlockwise transcription. Different colors indicate different functional classifications of the gene. The inner circle represents the GC content of the chloroplast genome.
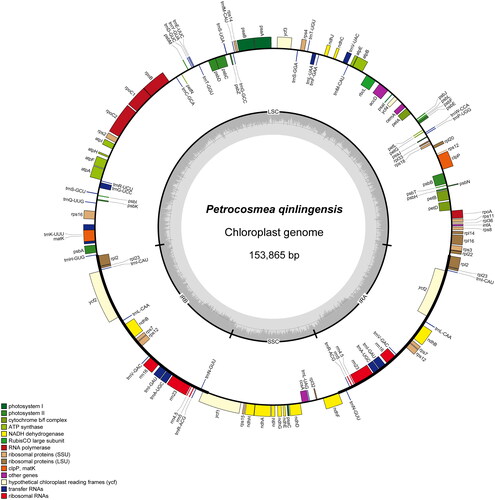
Gesneriaceae contains three subfamilies, namely Subfamily Gesnerioideae, Subfamily Sanangoideae, and Subfamily Didymocarpoideae (Weber et al. Citation2013; Ogutcen et al. Citation2021). Since the Subfam. Sanangoideae only contains one genus, Sanango, no chloroplast genome has been reported yet. The phylogenetic relationship we reconstructed based on the published chloroplast protein-coding genes of representative species divided into two clades (), which was consistent with previous results (Ogutcen et al. Citation2021). Those two clades were supported 100%, respectively. Our newly sequenced P. qinlingensis belongs to Subfam. Didymocarpoideae, and Raphiocarpus is its close sister branch, with a support value of 100%, consistent with previous research (Qiu et al. Citation2015; Ogutcen et al. Citation2021).
Figure 3. Phylogenetic tree based on the chloroplast protein-coding gene sequences of P. qinlingensis and its relatives. Bootstrap values were shown at nodes, asterisk indicates 100%. the following 39 chloroplast genomes were used: Achimenes cettoana NC_050917.1 (Li et al. Citation2021); Actinostephanus enpingensis NC_070427, Beccarinda cordifolia NC_070426.1, Boeica ferruginea NC_070431.1, Boeica multinervia NC_070432.1, Leptoboea multiflora NC_070430.1, Litostigma coriaceifolium NC_070433.1, Rhynchotechum nirijuliense NC_070428.1 and Rhynchotechum vestitum NC_070429.1 (Wen et al. Citation2022); Boea hygrometrica NC_016468.1 (Zhang et al. Citation2011); Briggsia chienii NC_063548.1 (Xu et al. Citation2022); Chirita eburnean NC_036100.1 (Hou et al. Citation2017); Corallodiscus flabellatus NC_050944.1 (Zhao et al. Citation2020); Hemiboea ovalifolia NC_054358.1 and Hemiboea malipoensis NC_080279.1 (Tian and Wariss Citation2021); Lysionotus pauciflorus NC_034660.1 (Ren et al. Citation2016); Oreocharis cotinifolia NC_053771.1 and Oreocharis mileensis MK342624.1 (Tang et al. Citation2021); Paraboea clavisepala NC_058814.1 and Paraboea rufescens NC_058817.1 (Wang et al. Citation2022); Petrocodon coriaceifolius NC_065790.1, Petrocodon multiflorus NC_065791.1, Primulina cordata NC_065792.1 and Primulina hezhouensis NC_065794.1 (Hsieh et al. Citation2022); Streptocarpus ionanthus subsp. velutinus MN935472.1 and Streptocarpus teitensis NC_037184.1 (Kyalo et al. Citation2020); Achimenes erecta NC_051524.1, Anna mollifolia NC_077643.1, Anna ophiorrhizoides NC_077644.1, Beccarinda tonkinensis NC_072338.1, Haberlea rhodopensis NC_031852.1, Henckelia pumila NC_065361.1, Loxostigma griffithii NC_082153.1, Pseudochirita guangxiensis NC_082154.1, Raphiocarpus begoniifolia NC_082152.1, Titanotrichum oldhamii NC_065773.1 and Whytockia hekouensis NC_082155.1 were direct submitted to NCBI; Tectona grandis NC_020098.1 (Maheswari et al. Citation2021) and Callicarpa americana NC_052696.1 (Hamilton et al. Citation2020).
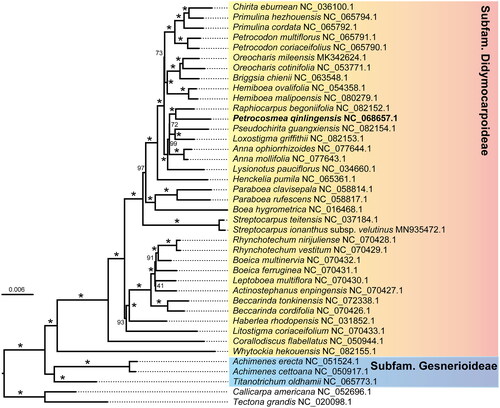
Discussion and conclusion
Petrocosmea consists of about 70 species, with elegant flowers and rosette leaves, is considered a potential flower with great development value (Qiu et al. Citation2015; Cai et al. Citation2022). Genomic data is considered an important source for modern plant diversity and conservation. However, despite the potential held by this genus, its genome, encompassing the chloroplast, mitochondrial, and nuclear, remains unexplored so far. Hence, we reported the first chloroplast genome of Petrocosmea in this study. Moreover, the chloroplast-based phylogenetic analyses support Raphiocarpus as its close sister branch, with a support value of 100%, consistent with previous research (Wang et al. Citation2010; Qiu et al. Citation2015). Chloroplasts are the site of photosynthesis and contain genes related to photosynthesis, which can be inherited independently. These genes will leave fingerprints in chloroplasts under different light environment (Zhang et al. Citation2016). Despite the Petrocosmea species are mainly distributed from a very dark (few species, e.g. P. serica on the cliff cracks with weak scattered light), to short-term direct light (e.g. P. bicolor on the shady limestone cliff with no shrubs covered). But, the adaptative mechanisms of this genus to low-light habitats still unrevealed. Our results would provide some clues. In conclusion, we reported the first chloroplast genome of Petrocosmea, providing an invaluable genetic resource for species conservation, trait improvement, and future applications of this genus.
Ethical approval Statement
This research was carried out in accordance with guidelines provided by the Institute of Botany of the Chinese Academy of Sciences in Beijing. Field works have complied with local legislation, and appropriate permissions/license were granted. The plant samples were collected in accordance with the regulations of the International Union for Conservation of Nature (IUCN), which did not cause any destruction to this endangered species.
Author contributions
SK, GL, and CL were involved in the conception and design, drafting, of the paper critically for intellectual content; CL, YL, and QM were involved in the analysis and interpretation of the data; and all authors agree to be accountable for all aspects of the work.
Supplemental Material
Download TIFF Image (2 MB)Supplemental Material
Download TIFF Image (1.6 MB)Disclosure statement
No potential conflict of interest was reported by the author(s).
Data availability statement
The genome sequence data that support the findings of this study are openly available in the GenBank of NCBI at [https://www.ncbi.nlm.nih.gov] (https://www.ncbi.nlm.nih.gov/) under the accession OM677633/NC_068657. The associated BioProject, SRA, and Bio-Sample numbers are PRJNA804599, SRR17952273, and SAMN25761307, respectively.
Additional information
Funding
References
- Bankevich A, Nurk S, Antipov D, Gurevich AA, Dvorkin M, Kulikov AS, Lesin VM, Nikolenko SI, Pham S, Prjibelski AD, et al. 2012. SPAdes: a new genome assembly algorithm and its applications to single-cell sequencing. J Comput Biol. 19(5):455–477. doi:10.1089/cmb.2012.0021.
- Cai L, Ya J, Yu Z, Liang Z, Wen F, Cai J. 2022. Petrocosmea hsiwenii (Gesneriaceae), a new species from Yunnan, China. Taiwania. 67:591–594.
- Camus MF, Alexander-Lawrie B, Sharbrough J, Hurst GDD. 2022. Inheritance through the cytoplasm. Heredity. 129(1):31–43. doi:10.1038/s41437-022-00540-2.
- Doyle JJ, Doyle JL. 1987. A rapid DNA isolation procedure for small quantities of fresh leaf tissue. Phytochemical Bulletin. 19:11–15.
- Forsythe ES, Sharbrough J, Havird JC, Warren JM, Sloan DB. 2019. CyMIRA: the cytonuclear molecular interactions reference for Arabidopsis. Genome Biol Evol. 11(8):2194–2202. doi:10.1093/gbe/evz144.
- Freudenthal JA, Pfaff S, Terhoeven N, Korte A, Ankenbrand MJ, Förster F. 2020. A systematic comparison of chloroplast genome assembly tools. Genome Biol. 21(1):254. doi:10.1186/s13059-020-02153-6.
- Greiner S, Lehwark P, Bock R. 2019. OrganellarGenomeDRAW (OGDRAW) version 1.3.1: expanded toolkit for the graphical visualization of organellar genomes. Nucleic Acids Res. 47(W1):W59–W64. doi:10.1093/nar/gkz238.
- Hamilton JP, Godden GT, Lanier E, Bhat WW, Kinser TJ, Vaillancourt B, Wang H, Wood JC, Jiang J, Soltis PS, et al. 2020. Generation of a chromosome-scale genome assembly of the insect-repellent terpenoid-producing Lamiaceae species, Callicarpa americana. GigaScience. 9(9):1–11. doi:10.1093/gigascience/giaa093.
- Hou N, Wang G, Li C-R, Luo Y. 2017. Characterization of the complete chloroplast genomes of three Chirita species (C. brachytricha, C. eburnea & C. liboensis) endemic to China. Conservation Genet Resour. 10(4):597–600. doi:10.1007/s12686-017-0873-3.
- Hsieh CL, Xu WB, Chung KF. 2022. Plastomes of limestone karst gesneriad genera Petrocodon and Primulina, and the comparative plastid phylogenomics of Gesneriaceae. Sci Rep. 12(1):15800. doi:10.1038/s41598-022-19812-2.
- Jiang J, Sun W, Hu X, Li Y, Hu F, Wang Y, Wang Q. 2019. Research status of the breeding of rare and endangered plant Petrocosmea qinlingensis. Molec Plant Breed. 17:3024–3029.
- Kyalo CM, Li ZZ, Mkala EM, Malombe I, Hu GW, Wang QF. 2020. The first glimpse of Streptocarpus ionanthus (Gesneriaceae) phylogenomics: analysis of five subspecies’ chloroplast genomes. Plants. 9(4):4. doi:10.3390/plants9040456.
- Li S, Liao R, Xin ZB, Huang ZJ, Maciejewski S, Wen F. 2021. The complete chloroplast genome sequence of Achimenes cettoana (Gesneriaceae). Mitochondrial DNA B Resour. 6(3):705–707. doi:10.1080/23802359.2020.1847607.
- Liu S, Ni Y, Li J, Zhang X, Yang H, Chen H, Liu C. 2023. CPGView: a package for visualizing detailed chloroplast genome structures. Mol Ecol Resour. 23(3):694–704. doi:10.1111/1755-0998.13729.
- Lu Z, Qin H, Jin X, Zhang Z, Yang Q, Hong D, Li D, Li K, Yuan L, Zhou Z. 2021. On the necessity, principle, and process of updating the List of National Key Protected Wild Plants. Biodiversity Science. 29(12):1577–1582. doi:10.17520/biods.2021394.
- Maheswari P, Kunhikannan C, Yasodha R. 2021. Chloroplast genome analysis of Angiosperms and phylogenetic relationships among Lamiaceae members with particular reference to teak (Tectona grandis L.f). J Biosci. 46(2):43. doi:10.1007/s12038-021-00166-2.
- Mogensen HL. 1996. The hows and whys of cytoplasmic inheritance in seed plants. Ame J Botany. 83(3):383–404. doi:10.1002/j.1537-2197.1996.tb12718.x.
- Nakamura T, Yamada KD, Tomii K, Katoh K. 2018. Parallelization of MAFFT for large-scale multiple sequence alignments. Bioinformatics. 34(14):2490–2492. doi:10.1093/bioinformatics/bty121.
- Ogutcen E, Christe C, Nishii K, Salamin N, Möller M, Perret M. 2021. Phylogenomics of Gesneriaceae using targeted capture of nuclear genes. Mol Phylogenet Evol. 157:107068. doi:10.1016/j.ympev.2021.107068.
- Kück P, Longo GC. 2014. FASconCAT-G: extensive functions for multiple sequence alignment preparations concerning phylogenetic studies. Front Zool. 11(1):81. doi:10.1186/s12983-014-0081-x.
- Qiu ZJ, Lu YX, Li CQ, Dong Y, Smith JF, Wang YZ. 2015. Origin and evolution of Petrocosmea (Gesneriaceae) inferred from both DNA sequence and novel findings in morphology with a test of morphology-based hypotheses. BMC Plant Biol. 15(1):167. doi:10.1186/s12870-015-0540-3.
- Qu XJ, Moore MJ, Li DZ, Yi TS. 2019. PGA: a software package for rapid, accurate, and flexible batch annotation of plastomes. Plant Methods. 15(1):50. doi:10.1186/s13007-019-0435-7.
- Ren T, Zheng W, Han K, Zeng S, Zhao J, Liu Z-L. 2016. Characterization of the complete chloroplast genome sequence of Lysionotus pauciflorus (Gesneriaceae). Conservation Genet Resour. 9(2):185–187. doi:10.1007/s12686-016-0638-4.
- Sierra J, Escobar-Tovar L, Leon P. 2023. Plastids: diving into their diversity, functions, and their role in plant development. J Exp Bot. 74(8):2508–2526. doi:10.1093/jxb/erad044.
- Stamatakis A. 2014. RAxML version 8: a tool for phylogenetic analysis and post-analysis of large phylogenies. Bioinformatics. 30(9):1312–1313. doi:10.1093/bioinformatics/btu033.
- Talavera G, Castresana J. 2007. Improvement of phylogenies after removing divergent and ambiguously aligned blocks from protein sequence alignments. Syst Biol. 56(4):564–577. doi:10.1080/10635150701472164.
- Tang J, Zhao B, Li C, Hong X. 2021. Complete chloroplast genome sequence of an endangered plant Oreocharis cotinifolia (Gesneriaceae) from Guangxi, China. Mitochondrial DNA B Resour. 6(10):2936–2938. doi:10.1080/23802359.2021.1973918.
- Tian X, Wariss HM. 2021. The complete chloroplast genome sequence of Metabriggsia ovalifolia W. T. Wang (Gesneriaceae), a national key protected plant endemic to Karst areas in China. Mitochondrial DNA B Resour. 6(3):833–834. doi:10.1080/23802359.2021.1884021.
- Tonti-Filippini J, Nevill PG, Dixon K, Small I. 2017. What can we do with 1000 plastid genomes? Plant J. 90(4):808–818. doi:10.1111/tpj.13491.
- Wang W. 1981. Notulae de Gesneriaceis sinensibus (II). Bull Botan Res. 1:36–37.
- Wang Y-Z, Liang R-H, Wang B-H, Li J-M, Qiu Z-J, Li Z-Y, Weber A. 2010. Origin and phylogenetic relationships of the Old World Gesneriaceae with actinomorphic flowers inferred from ITS and trnL-trnF sequences. Taxon. 59(4):1044–1052. doi:10.1002/tax.594005.
- Wang Y, Wen F, Hong X, Li Z, Mi Y, Zhao B. 2022. Comparative chloroplast genome analyses of Paraboea (Gesneriaceae): insights into adaptive evolution and phylogenetic analysis. Front Plant Sci. 13:1019831. doi:10.3389/fpls.2022.1019831.
- Weber A, Clark JL, Möller M. 2013. A new formal classification of Gesneriaceae. Selbyana. 31:68–94.
- Wen F, Xin ZB, Hong X, Cai L, Chen XY, Liang JJ, Wang HF, Maciejewski S, Wei YG, Fu LF. 2022. Actinostephanus (Gesneriaceae), a new genus and species from Guangdong, South China. PhytoKeys. 193:89–106. doi:10.3897/phytokeys.193.80715.
- Wick RR, Schultz MB, Zobel J, Holt KE. 2015. Bandage: interactive visualization of de novo genome assemblies. Bioinformatics. 31(20):3350–3352. doi:10.1093/bioinformatics/btv383.
- Xu X, Shi M, Shi L, Zhang W, Liu J, Duan X. 2022. The first complete chloroplast genome of Briggsia chienii W. Y. Chun and its phylogenetic position within Gesneriaceae. Mitochondrial DNA B Resour. 7(6):1120–1122. doi:10.1080/23802359.2022.2087551.
- Zhang T, Zhang X, Hu S, Yu J. 2011. An efficient procedure for plant organellar genome assembly, based on whole genome data from the 454 GS FLX sequencing platform. Plant Methods. 7(1):38. doi:10.1186/1746-4811-7-38.
- Zhang Y, Du L, Liu A, Chen J, Wu L, Hu W, Zhang W, Kim K, Lee S-C, Yang T-J, et al. 2016. The complete chloroplast genome sequences of five Epimedium species: lights into phylogenetic and taxonomic analyses. Front Plant Sci. 7:306. doi:10.3389/fpls.2016.00306.
- Zhao F, Tao A, Qian J, Xia C. 2020. Characterization of the complete chloroplast genome of Corallodiscus flabellatus (Gesneriaceae), a medicinal plant in southwest of China. Mitochondrial DNA Part B-Resour. 5(2):1489–1490. doi:10.1080/23802359.2020.1742594.