Abstract
Cornus hongkongensis Hemsl. 1888, native to Hong Kong, belongs to the subgenus Syncarpea within the Cornus genus of the Cornaceae family. The complete chloroplast genome of C. hongkongensis spans 156,954 bp, comprising four subregions: a large single-copy region (86,290 bp), a small single-copy region (18,394 bp), and a pair of inverted repeats (26,135 bp). Within the chloroplast genome of C. hongkongensis, we identified 113 unique genes, including 80 protein-encoding genes, four ribosomal RNA (rRNA) genes, and 30 transfer RNA (tRNA) genes. Phylogenetic analysis based on the complete chloroplast genome of 30 related taxa of the Cornus genus indicates that C. hongkongensis has not formed a monophyletic lineage. Analyses of sequence divergence found three intergenic regions including rps19-rpl22, ccsA-ndhD, and atpH-atpI, exhibiting a high degree of variations. The first chloroplast genome of C. hongkongensis was reported in this work contributes to the enrichment of genomic data for the genus Cornus.
Introduction
Cornus hongkongensis Hemsl. 1888, a member of the subgenus Syncarpea within the Cornus genus of the Cornaceae family, is a small evergreen tree or shrub with distinctive four-flowered flowers native to regions including Hong Kong, Jiangxi, Fujian, and Zhejiang, typically thriving at altitudes ranging from 250 to 1200 m (Jijun et al. Citation2017). Renowned for its ecological and economic significance, this species is a visually captivating tree, harmoniously combining colorful flowers, leaves, and fruits, enhancing its ornamental appeal (Jijun et al. Citation2017). The fruit of C. hongkongensis is not only nutritionally rich and sweet but also suitable for winemaking. Moreover, its medicinal properties include promoting of menstrual regularity, enhancing of blood circulation, and anti-inflammatory effects. These attributes establish it as a rare and valuable wild fruit tree germplasm resource (Yu-Hong et al. Citation2003). Furthermore, its hardwood and robust root system make it an exceptional choice for specialized timber applications, soil stabilization, and water retention. Consequently, it plays a pivotal role in afforestation, landscape beautification, and soil fertility restoration (Kaveriappa et al. Citation1997).
As a result, the demand for C. hongkongensis in ornamental gardens has steadily increased (Dan-Qi and Cheng-Xiang Citation2008). Notably, C. hongkongensis, a species within this category found in Hong Kong, lacks a publicly available chloroplast genome, hindering a precise phylogenetic understanding. To address this knowledge gap, our study aims to construct a high-quality assembled chloroplast genome, offering deeper molecular insights into germplasm relationships, genetic diversity, and phylogeny within this botanical context.
Materials and methods
Fresh leaves of C. hongkongensis () were collected with permission from the Guidong Botanical Garden, located in Chenzhou City, Hunan Province, China (latitude 24.98N, longitude 112.89E). The specimen was deposited at the Institute of Medicinal Plant Development, Chinese Academy of Medical Sciences, Peking Union Medical College, Beijing (contact person: Haimei Chen, [email protected]) under the voucher number Implad20230711004. Subsequently, the sequencing library with 200–400 bp insert sizes was constructed and sequenced on the DNBSEQ-T7RS platform (MGI) at Grandomics (Beijing, China). This process yielded a total of 10.8 Gb of raw data. The raw reads were then filtered using Trimmomatic (Bolger et al. Citation2014) to remove adaptors and low-quality sequences. As a result, a total of 10.6 GB of paired-end data were retrieved. The clean data were employed to de novo assemble the plastid genome using GetOrganelle (Jin et al. Citation2020) with default parameters. To assess the quality of the assembly, the clean data were mapped onto the assembly result using minimap2 (Li Citation2018). The aligned reads were extracted, and the depth and coverage were calculated using Samtools (v1.12; Danecek et al. Citation2021). The annotations for the plastid genome of C. Hongkongensis were generated by CPGAVAS2 (Shi et al. Citation2019) with the reference annotation of Cornus capitata (NC_060994.1) and adjusted manually using Apollo (Misra and Harris Citation2005). The map of the plastid genome, cis-spliced genes, and trans-spliced genes of C. hongkongensis was drawn by CPGview (Liu et al. Citation2023). The phylogenetic relationships among C. hongkongensis and 29 other Cornus species were analyzed based on the complete plastome sequences. Two Alangium species, namely, Alangium alpinum (MG525003.1) and Alangium chinense subsp. strigosum (OR197702.1) were chosen as outgroups. The complete plastomes of 32 species were aligned by MAFFT (Katoh and Standley Citation2013) with default parameters. The poorly aligned positions and divergent regions of the alignment were eliminated using Gblocks (v0.91b; Talavera and Castresana Citation2007). The maximum-likelihood (ML) tree was constructed in IQtree (v2.1.2 (Minh et al. Citation2020)) with ultrafast bootstrap (1000 replicates) and best-fit model TVM + F + R3. The intergenic spacer (IGS) regions of 25 Cornus cp genomes were extracted with our custom script. Then, Distmat program that was implemented in EMBOSS (v6.3.1) (Rice et al. Citation2000) was used to determine the pairwise distance of the intergenic regions with the Kimura 2-parameters (K2p) evolution model.
Figure 1. Photograph of C. hongkongensis leaf, flower, and fruit. (A) Leaf. This image was taken by Lichai Yuan at Guidong Botanical Garden, Chenzhou city, Hunan province, China. The leaf blade displays an elliptic, oblong-elliptic, or obovate-oblong shape with a thinly to thickly leathery. The leaf base is cuneate or broadly cuneate to rounded, and the apex is shortly acuminate to caudate. Typically, there are 3 or 4 (or occasionally 5) veins that ascend in a curved pattern. (B) Flower. This image was taken by Jun Liu at Hangzhou City, Zhejiang Province, China. Capitate cymes are globose, and the bracts are yellowish or white, broadly elliptic, broadly ovate, or orbicular to obovate. (C) Fruit. This image was taken by Yan Liu. The compound fruit, which is globose in shape, turns red or yellowish-red at maturity.
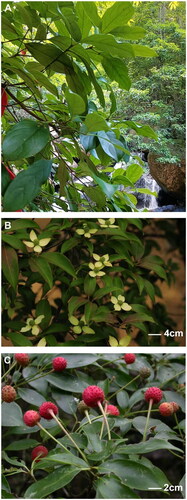
Results
A total of 751,194 paired reads were assembled to the complete plastid genome of C. hongkongensis, revealing a median depth of 1413, with a minimum depth of 540 and a max depth of 2213 (Figure S1). The assembled plastid genome has been deposited to the NCBI (National Center for Biotechnology Information, https://www.ncbi.nlm.nih.gov/) under the accession number OR545260. The plastome exhibits a quadripartite structure with 156,954 bp in length, consisting of a pair of inverted repeats (IRs, 26,135 bp), a large single-copy (LSC, 86,290 bp) region, and a small single-copy (SSC, 18,394 bp) (). The overall GC content of C. hongkongensis plastome was 38%, with the LSC, SSC, and IRs regions exhibiting GC contents of 36%, 32%, and 43%, respectively. A total of 132 genes (114 unique genes) were annotated, including 85 protein-coding genes (PCGs, 80 are unique), 37 transfer RNA genes (tRNAs, 30 are unique), eight ribosomal RNA genes (rRNAs, four are unique). Among them, a total of 15 unique genes (including rps16, atpF, rpoC1, petB, petD, rpl16, rpl2, ndhB, ndhA, trnK-UUU, trnG-UCC, trnL-UAA, trnV-UAC, trnI-GAU, and trnA-UGC) containing one intron and two unique genes containing two introns (including ycf3 and clpP) (Figure S2). The rps12 is a trans-spliced gene as shown in Figure S3. Sixteen genes were duplicated in IR regions, including seven PCGs (rpl2, rpl23, ycf15, ycf2, ndhB, rps7, and rps12), six tRNA genes (trnN-GUU, trnR-ACG, trnA-UGC, trnI-GAU, trnV-GAC, and trnL-CAA), and four rRNA genes (rrn16, rrn23, rrn4.5, and rrn5).
Figure 2. The complete plastome map of C. hongkongensis, which was generated by CPGview. LSC, SSC, and IRs (IRa and IRb) with their length are represented on the first circle. The second circle showed the GC ratio in dark gray. The outermost circle indicates gene name color-coded by their functional classification. The transcription directions for the inner and outer genes are clockwise and anticlockwise, respectively. The functional classification of the genes is shown in the left bottom corner. The optional codon usage bias is displayed in the parenthesis after the gene name.
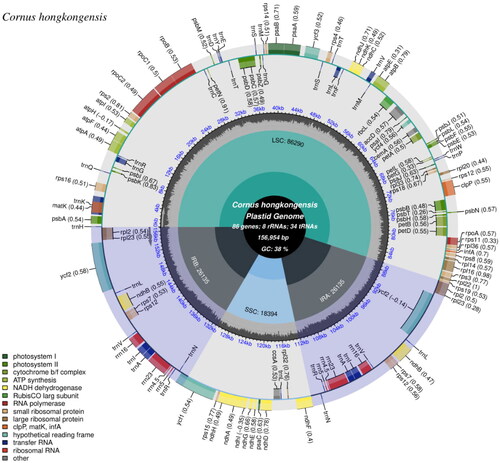
A ML phylogenetic analysis based on the complete chloroplast genome of 25 related taxa of the Cornus genus showed that the genus Cornus forms four major clades: the big-bracted (BB) group, the dwarf dogwoods (DW), the cornelian cherries (CC), and the blue- or white-fruited group (BW) (). Subgenus Syncarpea, encompassing C. hongkongensis, constitutes a monophyletic group. C. hongkongensis (OR545260) forms a distinct cluster alongside subsp. elegans, subsp. gigantea, and subsp. tonkinensis, exhibiting a robust statistical support value of 88%. While C. hongkongensis subsp. ferruginea clusters with Cornus kousa and C. hongkongensis subsp. melanotricha clusters with Cornus sunhangii.
Figure 3. A maximum-likelihood (ML) based phylogenetic tree of C. hongkongensis and related Cornus species. The cladogram was shown in the lower left corner. The scale bar represents the number of nucleotide substitutions per site. The numbers on each node indicated the ML bootstrap support with 1000 replicates. The subgenus is labeled next to the species name. The bold font indicates that genus Cornus was classified into four major clades: the big-bracted (BB) group, the dwarf dogwoods (DW), the cornelian cherries (CC), and the blue- or white-fruited group (BW). The following sequences were used: Alangium alpinum (MG525003.1) (Fu et al. Citation2017), Alangium chinense subsp. strigosum (OR197702.1) (Yang et al. Citation2022), Cornus peruviana (NC_044825.1) (Fu et al. Citation2019), Cornus oblonga (NC_044811.1) (Fu et al. Citation2019), Cornus alternifolia (NC_044812.1) (Fu et al. Citation2019), Cornus controversa (NC_030260.1), Cornus wilsoniana (NC_063837.1), Cornus bretschneideri (NC_060800.1) (Li et al. Citation2020), Cornus walteri (NC_058318.1), Cornus sanguinea (NC_044817.1) (Fu et al. Citation2019), Cornus alba (NC_059720.1) (Yuan et al. Citation2021), Cornus macrophylla (NC_044810.1) (Fu et al. Citation2019), Cornus sessilis (NC_044814.1) (Fu et al. Citation2019), Cornus chinensis (NC_044815.1) (Fu et al. Citation2019), Cornus eydeana (NC_044816.1) (Fu et al. Citation2019), Cornus officinalis (NC_042746.1), Cornus suecica (NC_044823.1) (Fu et al. Citation2019), Cornus unalaschkensis (NC_044824.1) (Fu et al. Citation2019), Cornus canadensis (NC_044822.1) (Fu et al. Citation2019), Cornus disciflora (NC_044819.1) (Fu et al. Citation2019), Cornus florida (NC_044820.1) (Fu et al. Citation2019), Cornus florida var. urbiniana (MN380671.1) (Fu et al. Citation2019), Cornus sunhangii (NC_060994.1) (Lv et al. Citation2019), Cornus capitata (MG524998.1) (Fu et al. Citation2017), Cornus hongkongensis (OR545260.1), Cornus elliptica (NC_056267.1) (Lu et al. Citation2021), Cornus kousa (NC_044818.1) (Fu et al. Citation2019), Cornus hongkongensis subsp. Gigantea (OR597583.1), Cornus hongkongensis subsp. Tonkinensis (OR597581.1), Cornus hongkongensis subsp. Elegans (OR597582.1), Cornus hongkongensis subsp. Ferruginea (OR597579.1), and Cornus hongkongensis subsp. Melanotricha (OR597580.1).
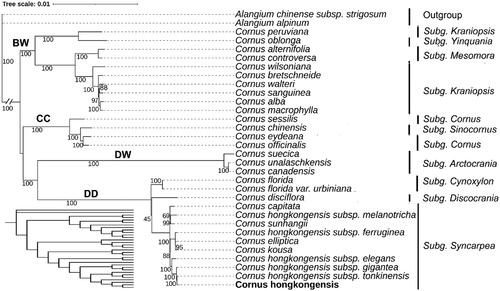
The pairwise comparison of IGS regions was conducted among the 25 Cornus species to identify hypervariable regions using the K2p model. The K2p distance, spanning from 0.00 to 44.46, was observed among the 124 IGSs of the 25 Cornus species (Table S1). Among them, the IGS regions rps19-rpl22, ccsA-ndhD, and atpH-atpI showed average distances of 11.88, 11.25, and 9.85, respectively, among the 25 Cornus species (). These specific IGS regions present potential utility as molecular markers for discerning plant phylogeny at lower taxonomic levels and for DNA barcoding within Cornus species.
Figure 4. Results of genetic distance analysis of intergenic spacer regions in Cornus species. The top 30 IGS of K2p distances are shown. The X-axis indicates the name of IGS regions. And the Y-axis shows the range of K2p distances between different pairs of species. The diamond shows the average K2p distance.
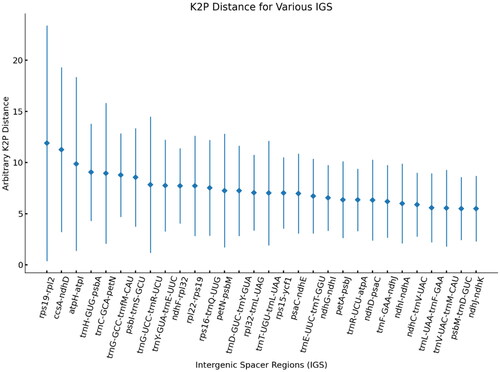
Discussion and conclusions
Cornus encompasses approximately 58 species, primarily comprising hermaphroditic shrubs and small trees. These Cornus species widely distribute in the temperate and subtropical regions of the Northern Hemisphere, with rare occurrences in tropical areas. The taxonomic composition, ranking, and relationships of subgroups within the genus have been subjects of contention for nearly a century. The phylogenetic analysis utilizing complete plastome sequences in this study supported the monophyly of most subgenera, except subg. Kraniopsis and subg. Cornus. C. peruviana belonging to Subg. Kraniopsis was clustered with C. oblonga belonging to Subg. Yinquania. Additionally, subg. Sinocornus were nested within subg. Cornus. This finding is consistent with previous studies, which integrated morphology, matK, ITS, and previously published rbcL and 26S rDNA sequences (Xiang et al. Citation2006).
Xiang (Citation1987) recognized six subspecies within C. hongkongensis, including subsp. gigantea, subsp. tonkinensis, subsp. elegans, subsp. ferruginea, subsp. melanotricha, and subsp. hongkongensis based on their habits and buds. Subsp. C. hongkongensis is much controversial owing to the diversity of evaluation of characters. Our phylogenetic analysis based on the whole cp genomes showed that six subspecies within C. hongkongensis were not clustered together. As a result, a reasonable system reflecting the infra-subspecies relationships is still lacking and further data will be needed to reevaluate the relationship of subspecies within C. hongkongensis. The result enriches the genomic data for the genus Cornus, which will contribute to phylogenetic and evolutionary studies in the future. In this study, we found that the K2p values were particularly high for the three IGS regions: rps19-rpl22, ccsA-ndhD, and atpH-atpI. These IGS regions can be used to distinguish Cornus species.
Author contributions
ZL and JJW made substantial contributions to the design of this work and final approval of the version to be published; LPH, XRC, and YZ analyzed the data; WXH and YL drafted this work; HMC and JJW reviewed this work critically. All authors agreed to be accountable for all aspects of this work.
Ethical approval
The sample of Cornus hongkongensis was collected with the permission from the Guidong Botanical Garden, Chenzhou city, Hunan Province, China and strictly complied with local and Chinese regulations. No ethical approval is required in this study.
Supplemental Material
Download MS Word (533.8 KB)Supplemental Material
Download MS Excel (202.7 KB)Disclosure statement
No potential conflict of interest was reported by the author(s).
Data availability statement
The genome sequence data that support the findings of this study are openly available in GenBank of NCBI at https://www.ncbi.nlm.nih.gov/ under the accession number OR545260.1. The associated BioProject, BioSample, and SRA numbers are PRJNA1033361, SAMN38031310, and SRR26559992, respectively.
Additional information
Funding
References
- Bolger AM, Lohse M, Usadel B. 2014. Trimmomatic: a flexible trimmer for Illumina sequence data. Bioinformatics. 30(15):2114–2120. doi:10.1093/bioinformatics/btu170.
- Danecek P, Bonfield JK, Liddle J, Marshall J, Ohan V, Pollard MO, Whitwham A, Keane T, McCarthy SA, Davies RM, et al. 2021. Twelve years of SAMtools and BCFtools. GigaScience. 10(2):giab008. doi:10.1093/gigascience/giab008.
- Dan-Qi X, Cheng-Xiang LU. 2008. Germplasm resource distribution and recent research advance in Dendrobenthamia. J Gansu Forest Sci Technol. 33:24–26, 68.
- Fu CN, Li HT, Milne R, Zhang T, Ma PF, Yang J, Li DZ, Gao LM. 2017. Comparative analyses of plastid genomes from fourteen Cornales species: inferences for phylogenetic relationships and genome evolution. BMC Genomics. 18(1):956. doi:10.1186/s12864-017-4319-9.
- Fu CN, Mo ZQ, Yang JB, Ge XJ, Li DZ, Xiang QJ, Gao LM. 2019. Plastid phylogenomics and biogeographic analysis support a trans-Tethyan origin and rapid early radiation of Cornales in the Mid-Cretaceous. Mol Phylogenet Evol. 140:106601. doi:10.1016/j.ympev.2019.106601.
- Jijun X, Biting HE, Zhaoyou XU, Huanwei C, Jun LI. 2017. A study on container seedling quality grading of Cornus hongkongensis Hemsl. ssp. elegans. J Fujian Forest Sci Technol. 44(4):102-106. doi:10.13428/j.cnki.fjlk.2017.04.022.
- Jin J-J, Yu W-B, Yang J-B, Song Y, Depamphilis CW, Yi T-S, Li D-Z. 2020. GetOrganelle: a fast and versatile toolkit for accurate de novo assembly of organelle genomes. Genome Biol. 21(1):241. doi:10.1186/s13059-020-02154-5.
- Katoh K, Standley DM. 2013. MAFFT multiple sequence alignment software version 7: improvements in performance and usability. Mol Biol Evol. 30(4):772–780. doi:10.1093/molbev/mst010.
- Kaveriappa KM, Phillips LM, Trigiano RN. 1997. Micropropagation of flowering dogwood (Cornus florida) from seedlings. Plant Cell Rep. 16(7):485–489. doi:10.1007/bf01092771.
- Li H. 2018. Minimap2: pairwise alignment for nucleotide sequences. Bioinformatics. 34(18):3094–3100. doi:10.1093/bioinformatics/bty191.
- Li X, Ma Q, Zhou H, Yang Y, Li H, Wang J. 2020. Characterization of the complete chloroplast genome of Cornus bretschneideri (Cornaceae). Mitochondrial DNA B Resour. 5(1):543–544. doi:10.1080/23802359.2019.1710281.
- Liu S, Ni Y, Li J, Zhang X, Yang H, Chen H, Liu C. 2023. CPGView: a package for visualizing detailed chloroplast genome structures. Mol Ecol Resour. 23(3):694–704. doi:10.1111/1755-0998.13729.
- Lu H, Tang J, Li D. 2021. The complete chloroplast genome sequences of Cornus elliptica (Cornaceae). Mitochondrial DNA B Resour. 6(3):931–932. doi:10.1080/23802359.2021.1888333.
- Lv ZY, Huang XH, Luo J, Zhang X, Deng T, Li ZM. 2019. The complete chloroplast genome sequence of Cornus sunhangii (Cornaceae). Mitochondrial DNA B Resour. 4(2):3242–3243. doi:10.1080/23802359.2019.1669090.
- Minh BQ, Schmidt HA, Chernomor O, Schrempf D, Woodhams MD, von Haeseler A, Lanfear R. 2020. IQ-TREE 2: new models and efficient methods for phylogenetic inference in the genomic era. Mol Biol Evol. 37(5):1530–1534. doi:10.1093/molbev/msaa015.
- Misra S, Harris N. 2005. Using Apollo to browse and edit genome annotations. Curr Protoc Bioinform. 9(1):9.5.1–9.5.28. doi:10.1002/0471250953.bi0905s12.
- Rice P, Longden I, Bleasby A. 2000. EMBOSS: the European molecular biology open software suite. Trends Genet. 16(6):276–277. doi:10.1016/s0168-9525(00)02024-2.
- Shi L, Chen H, Jiang M, Wang L, Wu X, Huang L, Liu C. 2019. CPGAVAS2, an integrated plastome sequence annotator and analyzer. Nucleic Acids Res. 47(W1):W65–W73. doi:10.1093/nar/gkz345.
- Talavera G, Castresana J. 2007. Improvement of phylogenies after removing divergent and ambiguously aligned blocks from protein sequence alignments. Syst Biol. 56(4):564–577. doi:10.1080/10635150701472164.
- Xiang Q-Y, Thomas DT, Zhang W, Manchester SR, Murrell Z. 2006. Species level phylogeny of the genus Cornus (Cornaceae) based on molecular and morphological evidence – implications for taxonomy and tertiary intercontinental migration. TAXON. 55(1):9–30. doi:10.2307/25065525.
- Xiang Q-Y. 1987. System and synopsis of Cornus subgen. Syncarpea (Nakai) Q. Y. Xiang (Cornaceae). Bull Bot Res. 7(2):33–52.
- Yang X, Liu C, Zeng X, Liu X, Zhao H, Feng T, Zhou Y. 2022. Sequence structure and phylogenetic analysis of the chloroplast genomes of Alangium chinense (Lour.) Harms and its different subspecies. Yaoxue Xuebao. 57:3229–3239.
- Yuan W, He S, Zhang S, Chang D, He Y. 2021. The complete chloroplast genome sequence of Cornus alba L. (Cornaceae). Mitochondrial DNA B Resour. 6(7):1997–1998. doi:10.1080/23802359.2021.1938727.
- Yu-Hong Z, Guo-Zheng T, Ming-Shu XU, Yong-Mei YI. 2003. Determination of main nutritious ingredients in the fruit of Dendrobenthamia angustata. J Hubei Inst Natl. (4):21–22.