Abstract
We conducted an analysis of the complete mitochondrial genome of Rhipicephalus haemaphysaloides, a tick species known for transmitting various bacteria and viruses. The mitochondrial genome of R. haemaphysaloides has a length of 14,739 bp and consists of 13 protein-coding genes (PCGs), 22 transfer RNA genes (tRNAs), 2 ribosomal RNA genes (rRNAs), and 2 control regions. By utilizing the maximum likelihood method, we established the phylogenetic relationship among R. haemaphysaloides and other species within the Rhipicephalus genus of the Ixodidae family. This analysis revealed that R. haemaphysaloides and other Rhipicephalus species belong to the same clade, further affirming the taxonomic placement of R. haemaphysaloides within the Rhipicephalus genus. Furthermore, we compared the mitochondrial genomes of R. haemaphysaloides isolates from Changning, Yunnan Province, China, with isolates from Yangxin, Ganzhou, and Yingtan, Hubei Province, China. In summary, our investigation offers genetic proof endorsing the taxonomic categorization and phylogenetic placement of Ixodidae by assessing the entire mitochondrial genome of R. haemaphysaloides.
Introduction
Rhipicephalus haemaphysaloides Supino, 1897 () is an obligate parasitic arthropod that primarily parasitizes hares, hedgehogs, rodents, and domestic animals such as cattle, sheep, goats, horses, and pigs (Mansfield et al. Citation2009). It is mainly found in hot and humid Southeast Asian countries, including China, India, Malaysia, Indonesia (Diyes et al. Citation2017), Sri Lanka (Dilrukshi et al. Citation2004), and Thailand (Tantrawatpan et al. Citation2022). R. haemaphysaloides belongs to the family Ixodidae and the genus Rhipicephalus. Family Ixodidae is considered the second largest medium for disease transmission, after mosquitoes (Li et al. Citation2018). It is known as one of a significant vector that can impact both human and animal health (Dantas-Torres et al. Citation2012), as they can carry bacteria, viruses, protozoa (Li et al. Citation2018; Sharifah et al. Citation2020). They transmit diseases through blood-sucking bites or contact with the host’s body fluids, blood, or animal products (Huang et al. Citation2020). The pathogens they carry can cause symptoms such as nausea, headache, fever, cytopenias (Wang et al. Citation2019), and diseases such as meningitis and hemorrhagic fever (Bonnet et al. Citation2022), and even death (Tran et al. Citation2022). The small genome, stable genetic composition, and maternal inheritance are remarkable characteristics found within the mitochondria of insects (Yang et al. Citation2022). These unique attributes have proven to be invaluable in studies related to insect species identification and phylogenetic research. Nevertheless, the current exploration of R. haemaphysaloides has predominantly concentrated on morphological aspects, leaving limited room for comprehensive investigations into its complete mitochondrial genome. Consequently, it is of utmost significance to delve into the mitochondrial genome of R. haemaphysaloides to acquire a deeper understanding.
Materials and methods
Sample collection and DNA extraction
Adult R. haemaphysaloides specimens were collected from Changning City, located in Yunnan province, China (24°51′01″N, 99°35′55″E). These specimens were brought back to the laboratory and preserved in absolute ethanol and stored in a refrigerator set to −20 °C. After collection (n = 12), one specimen was used for DNA extraction, and the remainder of the ticks were held as voucher specimens. Subsequently, the collected tick specimens were deposited at the Parasitological Museum at Dali University in Yunnan, China. The collection number assigned to these samples is DLU230415 (URL: http://www.dali.edu.cn/jcyxy/xkpt/jcyxsyjxzx/6431.htm). Contact person: Xing Yang, [email protected]. The total genomic DNA was extracted using the CTAB technique following standard protocols. The isolated DNA was then stored in 75% ethanol at a temperature of −20 °C.
Sequence, assembly, and annotation analysis
The sequencing process for the mitochondrial genome of R. haemaphysaloides was carried out using the Illumina NovaSeq platform at Harbin Botai Biotechnology Co, Ltd, China. The sequencing process generated 2.7GB of raw data, due to the presence of low-quality data, the raw data underwent a filtering process to extract clean data. Subsequently, quality control measures were applied to the clean data, we got 21,875,512 clean paired reads. The A5-miseq software (Coil et al. Citation2015) was then utilized for genome assembly on the clean data post quality control. To conduct sequence annotations, the MITOS web server (Meng et al. Citation2019) was employed. DNAstar 11 (Burland Citation2000) software was utilized for the calculation of base composition, codon usage frequency, AT-skew, and GC-skew of each coding gene in the mitochondrial genome of R. haemaphysaloides. Finally, we used OGDRAW v1.3.1 software (Greiner et al. Citation2019) to map the complete mitochondrial genome of R. haemaphysaloides.
Phylogenetic analysis
The phylogenetic analysis was performed using the maximum likelihood method with a bootstrap value of 1000 and the General Time Reversible model. The tree was built on the concatenated datasets of 13 PCGs. Additionally, 20 previously reported mitochondrial genomes of Ixodidae were included. The mitochondrial genome of Limulus polyphemus was included as an outgroup. The MEGA11.0 (Tamura et al. Citation2021) software was utilized to conduct the phylogenetic analysis.
Results
Mitochondrial genome analysis of R. haemaphysaloides (changning isolate)
The sequence of R. haemaphysaloides’ mitochondrial genome measures 14,739 base pairs (bp) in length, exhibiting a mean coverage of trimmed sequencing data at 57.92×. This genome encompasses a total of 37 distinct genomes. These genomes consist of 13 PCGs, 22 tRNAs, 2 rRNAs, and 2 control regions (). Among the PCGs, nad5 has the longest gene length (1657 bp), while trnS1 has the shortest gene length (53 bp). Fourteen tRNAs,(trnM(cat), trnK(ctt), trnW(tca), trnD(gtc), trnR(tcg), trnG(tcc), trnA(tgc), trnN(gtt), trnS(tct), trnE(ttc), trnI(gat), trnT(tgt),trnS2(tga), and trnC(gca)), nine of the 13 PCGs (nad2, cox1, cox2, atp8, atp6, cox3, nad3, nad6, cob) are present on the heavy strand, while two rRNAs (rrnL, rrnS), eight tRNAs ((trnY(gta), trnL2(taa), trnV(tac), trnQ(ttg), trnF(gga), trnH(gtg), trnL1(tag), trnP(tgg)), four of the 13 PCGs (nad1, nad5, nad4, nad4l) are located on the light strand.
Within the complete mitochondrial genome of R. haemaphysaloides, there are instances of gene overlaps and intergenic intervals between adjacent genes. Specifically, there are 18 intergenic regions, with an overall length of 228 bp. These intergenic regions range in length from 1 to 49 bp, with the longest interval found between the genes cox1 and cox2 at 49 bp, followed by rrnS and the control region between rrnS and trnI at 37 bp. Additionally, there are 11 gene overlapping regions, totaling 43 bp, with lengths ranging from 1 to 14 bp. The largest gene overlap is observed between the genes nad6 and trnP.
Among the 13 PCGs, ATT is the initiation codon for nad2, cox1, cox2, nad3, and nad5, ATA is the initiation codon for nad1 and nad6, and ATC is the initiation codon for atp8. The remaining five PCGs all start with ATG as the initiation codon. Interestingly, TAA acts as the stop codon for nine of the 13 PCGs, while the genes cox2, cox3, and nad5 have the incomplete stop codon T. Concerning the base composition of the mitochondrial genome of R. haemaphysaloides, the proportions of A, G, C, and T are 37.47%, 10.10%, 12.99%, and 39.45%, respectively. The A + T content is 76.92% and G + C content is 23.08%, indicating a clear preference for AT bases. Moreover, both the AT-skew and GC-skew values are negative, suggesting that the amounts of bases A and G in the entire genome sequence are lower than those of T and C, respectively.
The analysis between mitochondrial genomes of R. haemaphysaloides (Changning, Yangxin, Ganzhou, and Yingtan isolates)
The analysis showed that the complete mitochondrial genome of R. haemaphysaloides isolated from Changning in Yunnan province is shorter than those of the Yangxin, Ganzhou, and Yingtan isolates in Hubei province. From , the R. haemaphysaloides from Yangxin, Ganzhou, and Yingtan in Hubei province and Changning in Yunnan province all have two control regions. ATG, ATT, ATC were used in the cox2, atp8, and nad1 as the start codon respectively (Yangxin, Ganzhou, Yingtan isolates), while Changning isolated was used ATT, ATC, ATA as the start condon of cox2, atp8, and nad1 gene separately. As for the nad4, the Changning isolate utilized TAG as the stop codon, whereas the Yingtan, Ganzhou, and Yangxin isolates employed TAA as the stop codon.
Table 1. Rhipicephalus haemaphysaloides gene content, length, coding strand, initiation, stop codons of mitochondrial genomes of different isolate.
Phylogenetic analysis
The phylogenetic tree revealed that R. haemaphysaloides isolated from Yangxin, Ganzhou, and Yingtan in Hubei province formed a cluster, which then grouped with the R. haemaphysaloides isolated from Changning in Yunnan province (). The results shown that R. haemaphysaloides (Changning isolates) belong to the Rhipicephalus genus.
Figure 3. Phylogenetic tree of Rhipicephalus haemaphysaloides and 20 previously published ixodidae tick species in GenBank based on the nucleotides of 13 PCGs of mitochondrial genomes used the maximum likelihood method by MEGA11.0, the numbers at the nodes are bootstrap values computed using 1,000 replications and General Time Reversible model. The following sequences were used: Dermacentor reticulatus/Russia Tomsk region (Kartashov et al. Citation2020), Rhipicephalus australis/Australia Queensland, Bunya (Burger et al. Citation2014), Rhipicephalus camicasi/Saudi Arabia Riyadh (Chandra et al. Citation2022), Rhipicephalus decoloratus/South Africa Uitspanning (Mans et al. Citation2019), Rhipicephalus evertsi/South Africa Uitspanning (Mans et al. Citation2019), Rhipicephalus linnaei/Egypt Luxor Governorate, Esna City (Šlapeta et al. Citation2022), Rhipicephalus sanguineus/China (Cao et al. Citation2023), Hyalomma asiaticum/China Changsha (Cao et al. Citation2023), Hyalomma marginatum/Turkey (Ciloglu et al. Citation2021), Hyalomma rufipes/China Hebei (Lang et al. Citation2022), Limulus polyphemus/USA (Lavrov et al. Citation2000).
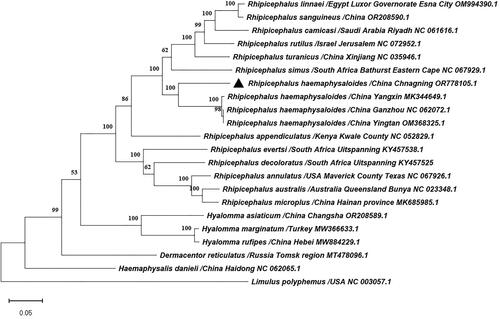
Discussion and conclusions
These findings suggest that geographic location (Simões and Pascual Citation2018) may play a role in mitochondrial genome variation in R. haemaphysaloides. In conclusion, this study provides valuable insights for further research on species identification, evolution, and phylogenetics of R. haemaphysaloides.
Ethical approval
This study was approved by the Administration Committee of Experimental Animals, Dali University, Yunnan Province, P.R. China.
Authors’ contributions
Shaobo Tang conceived the study and wrote the manuscript. Xiaoyun Zhang carried out the experiments and analyzed the data. Dandan Jiang and Chunhong Du contributed to the collection of Rhipicephalus haemaphysaloides and discussions, Xing Yang is responsible for the interpretation of experimental data, critical revision of important knowledge content and final approval of the version to be published.
Disclosure statement
No potential conflict of interest was reported by the authors.
Data availability statement
The data that support the findings of this study are openly available in GenBank of NCBI at https://www.ncbi.nlm.nih.gov. The accession number of the complete mitochondrial genome is OR778105. The associated BioProject, SRA, and Bio-Sample numbers were PRJNA1054497, SRR27313610, and SAMN38923201, respectively.
Additional information
Funding
References
- Bonnet SI, Vourc’h G, Raffetin A, Falchi A, Figoni J, Fite J, Hoch T, Moutailler S, Quillery E. 2022. The control of Hyalomma ticks, vectors of the Crimean–Congo hemorrhagic fever virus: where are we now and where are we going? PLoS Negl Trop Dis. 16(11):e0010846. doi:10.1371/journal.pntd.0010846.
- Burger TD, Shao R, Labruna MB, Barker SC. 2014. Molecular phylogeny of soft ticks (Ixodida: Argasidae) inferred from mitochondrial genome and nuclear rRNA sequences. Ticks Tick Borne Dis. 5(2):195–207. doi:10.1016/j.ttbdis.2013.10.009.
- Burland TG. 2000. DNASTAR's Lasergene sequence analysis software. Methods Mol Biol. 132:71–91. doi:10.1385/1-59259-192-2:71.
- Cao ML, Nie Y, Fu YT, Li R, Yi XL, Xiong J, Liu GH. 2023. Characterization of the complete mitochondrial genomes of five hard ticks and phylogenetic implications. Parasitol Res. 122(8):1907–1913. doi:10.1007/s00436-023-07891-7.
- Chandra S, Alanazi AD, Slapeta J. 2022. Mitochondrial genome of Rhipicephalus cf. camicasi Morel, Mouchet et Rodhain, 1976 from a camel (Camelus dromedarius Linnaeus) in Riyadh, Saudi Arabia. Folia Parasitol (Praha). 69:1–4. doi:10.14411/fp.2022.005.
- Ciloglu A, Ibis O, Yildirim A, Aktas M, Duzlu O, Onder Z, Simsek E, Yetismis G, Ellis VA, Inci A. 2021. Complete mitochondrial genome characterization and phylogenetic analyses of the main vector of Crimean-Congo haemorrhagic fever virus: Hyalomma marginatum Koch, 1844. Ticks Tick Borne Dis. 12(5):101736. doi:10.1016/j.ttbdis.2021.101736.
- Coil D, Jospin G, Darling AE. 2015. A5-miseq: an updated pipeline to assemble microbial genomes from Illumina MiSeq data. Bioinformatics. 31(4):587–589. doi:10.1093/bioinformatics/btu661.
- Dantas-Torres F, Chomel BB, Otranto D. 2012. Ticks and tick-borne diseases: a one health perspective. Trends Parasitol. 28(10):437–446. doi:10.1016/j.pt.2012.07.003.
- Dilrukshi P, Yasawardene A, Amerasinghe PH, Amerasinghe FP. 2004. Human otoacariasis: a retrospective study from an area of Sri Lanka. Trans R Soc Trop Med Hyg. 98(8):489–495. doi:10.1016/j.trstmh.2003.12.008.
- Diyes GCP, Apanaskevich DA, Rajakaruna RS. 2017. Lifecycle of Rhipicephalus haemaphysaloides under laboratory conditions. Med Vet Entomol. 31(3):327–332. doi:10.1111/mve.12238.
- Greiner S, Lehwark P, Bock R. 2019. OrganellarGenomeDRAW (OGDRAW) version 1.3.1: expanded toolkit for the graphical visualization of organellar genomes. Nucleic Acids Res. 47(W1):W59–W64. doi:10.1093/nar/gkz238.
- Huang T, Zhang J, Sun C, Liu Z, He H, Wu J, Geriletu. 2020. A novel arthropod host of brucellosis in the arid steppe ecosystem. Front Vet Sci. 7: 566253. doi:10.3389/fvets.2020.566253.
- Kartashov MY, Shvalov AN, Tupota NL, Romanenko VN, Moskvitina NS, Ternovoi VA, Loktev VB. 2020. Complete mitogenome of the ixodid tick Dermacentor reticulatus (Acari: Ixodida). Mitochondrial DNA B Resour. 5(3):3366–3368. doi:10.1080/23802359.2020.1821811.
- Lang J, Shan Y, Zhang M, Liu J, Wang F. 2022. The complete mitochondrial genome of Hyalomma rufipes (Acari: Ixodidae) from China and comparative analysis of mitogenomes in genus Hyalomma. Int J Acarol. 48(2):87–97. doi:10.1080/01647954.2022.2030794.
- Lavrov DV, Boore JL, Brown WM. 2000. The complete mitochondrial DNA sequence of the horseshoe crab Limulus polyphemus. Mol Biol Evol. 17(5):813–824. doi:10.1093/oxfordjournals.molbev.a026360.
- Li L-H, Zhang Y, Wang J-Z, Li X-S, Yin S-Q, Zhu D, Xue J-B, Li S-G. 2018. High genetic diversity in hard ticks from a China-Myanmar border county. Parasit Vectors. 11(1):469. doi:10.1186/s13071-018-3048-5.
- Mans BJ, Featherston J, Kvas M, Pillay K-A, de Klerk DG, Pienaar R, de Castro MH, Schwan TG, Lopez JE, Teel P. 2019. Argasid and ixodid systematics: implications for soft tick evolution and systematics, with a new argasid species list. Ticks Tick Borne Dis. 10(1):219–240. doi:10.1016/j.ttbdis.2018.09.010.
- Mansfield KL, Johnson N, Phipps L, Stephenson J, Fooks A, Solomon T. 2009. Tick-borne encephalitis virus–A review of an emerging zoonosis. J Gen Virol. 90(Pt 8):1781–1794. doi:10.1099/vir.0.011437-0.
- Meng G, Li Y, Yang C, Liu S. 2019. MitoZ: a toolkit for animal mitochondrial genome assembly, annotation and visualization. Nucleic Acids Res. 47(11):e63. doi:10.1093/nar/gkz173.
- Sharifah N, Heo CC, Ehlers J, Houssaini J, Tappe D. 2020. Ticks and tick-borne pathogens in animals and humans in the island nations of Southeast Asia: a review. Acta Trop. 209:105527. doi:10.1016/j.actatropica.2020.105527.
- Simões P, Pascual M. 2018. Patterns of geographic variation of thermal adapted candidate genes in Drosophila subobscura sex chromosome arrangements. BMC Evol Biol. 18(1):60. doi:10.1186/s12862-018-1178-1.
- Šlapeta J, Halliday B, Chandra S, Alanazi AD, Abdel-Shafy S. 2022. Rhipicephalus linnaei (Audouin, 1826) recognised as the “tropical lineage” of the brown dog tick Rhipicephalus sanguineus sensu lato: neotype designation, redescription, and establishment of morphological and molecular reference. Ticks Tick Borne Dis. 13(6):102024. doi:10.1016/j.ttbdis.2022.102024.
- Tamura K, Stecher G, Kumar S. 2021. MEGA11: molecular evolutionary genetics analysis version 11. Mol Biol Evol. 38(7):3022–3027. doi:10.1093/molbev/msab120.
- Tantrawatpan C, Vaisusuk K, Chatan W, Pilap W, Suksavate W, Andrews RH, Petney TN, Saijuntha W. 2022. Genetic diversity and phylogenetic analyses of ixodid ticks infesting cattle in northeast Thailand: the discovery of Rhipicephalus microplus clade C and the rarely detected R. haemaphysaloides. Exp Appl Acarol. 86(4):535–548. doi:10.1007/s10493-022-00704-z.
- Tran NTB, Shimoda H, Ishijima K, Yonemitsu K, Minami S, Kuroda Y, Tatemoto K, Mendoza MV, Kuwata R, Takano A, et al. 2022. Zoonotic infection with Oz virus, a novel Thogotovirus. Emerg Infect Dis. 28(2):436–439. doi:10.3201/eid2802.211270.
- Wang Z-D, Wang B, Wei F, Han S-Z, Zhang L, Yang Z-T, Yan Y, Lv X-L, Li L, Wang S-C. 2019. A new segmented virus associated with human febrile illness in China. N Engl J Med. 380(22):2116–2125. doi:10.1056/NEJMoa1805068.
- Yang H, Chen T, Dong W. 2022. The complete mitochondrial genome of Parasitus fimetorum (Berlese, 1904) (Arachnida: Parasitiformes: Parasitidae). Mitochondrial DNA B Resour. 7(6):1044–1045. doi:10.1080/23802359.2022.2081944.