Abstract
Helicteres hirsuta Lour. 1790 is a precious medicinal plant species, especially for treating chronic liver diseases. Genomic data on H. hirsuta are limited. Therefore, this current study aimed to characterize the chloroplast genome of H. hirsuta and reconstruct the phylogenetic relationship among Helicteroideae taxa. Consequently, the complete chloroplast genome of H. hirsuta was 163,404 bp in length and contained 113 unique genes (79 protein-coding genes, 30 tRNA genes, and four rRNA genes). Notably, two introns of clpP gene of H. hirsuta were lost in comparison to that of other Helicteroideae species. The phylogenetic tree based on chloroplast genomes of eleven Helicteroideae species revealed that H. hirsuta was closely related to Reevesia species. In conclusion, our study described the first complete chloroplast genome of H. hirsuta, which is essential for tracing evolutionary history in the Helicteroideae subfamily.
Introduction
Helicteres Pluk. ex L. 1753, a genus belonging to the Helicteroideae subfamily of Malvaceae, is characterized by stamens and pistils on an androgynophore, united sepals, and oblong fruits with hairs (Turner Citation1995). In Vietnam, twelve species and one variety of Helicteres were reported, in which two novel endemic Helicteres species (i.e. H. binhthuanensis V.S.Dang 2020 and H. daknongensis V.S.Dang & D.T.Bui 2020) have been recently described (Dang et al. Citation2020; Hoang et al. Citation2020). Helicteres hirsuta Lour. 1790, a species within this genus, has been recognized for its efficacy as a folk medicine in treating cirrhosis and liver cancer (Loureiro Citation1790; Thang Hoang et al. Citation2021). Several studies have investigated extracts derived from the leaves, stems, and roots of H. hirsuta, which contain phenolic, flavonoid, and saponin compounds. These compounds are recognized for their diverse bioactivities, including antioxidant, antimicrobial, anticancer, antidiabetic, and against malaria (Pham et al. Citation2017; Quang et al. Citation2020; Hieu et al. Citation2021; Nhat et al. Citation2022). Recent studies have screened the chemical composition of H. hirsuta, identified isolated compounds and secondary metabolites, and explored their biological activities (Nguyen et al. Citation2019; Tra et al. Citation2019; Le and Hoang Citation2020; Wang et al. Citation2021).
Chloroplast (cp) genomes have been used as “super-barcodes” in inference of evolutionary phylogeny of angiosperms due to their distinctive features of highly conserved structure, small size, and a non-homologous recombination (Wu et al. Citation2021; Zhang et al. Citation2021). Until now, the absence of cp genomic data for Helicteres has limited the research of the evolution and phylogenetic relationships of this genus and related taxa. Therefore, in this study, the sequenced and characterized complete chloroplast genome of H. hirsuta was reported. The results of this study will provide a valuable genomic resource that can facilitate future investigations into the identification of species, population genetics, and evolutionary history of H. hirsuta.
Materials and methods
Fresh and healthy leaf sample was collected from a single H. hirsuta individual from Tay Ninh province, Vietnam (011°20'29.9" N, 106°17'33.0" E) (). The specimen of H. hirsuta was deposited at NTT Hi-Tech Institute under the voucher number NTTU-20231101-P023 (contact person: Dr. Hoang Dang Khoa Do, email: [email protected]). Fresh leaves were stored in silica gel at room temperature at NTT Hi-Tech Institute, Nguyen Tat Thanh University for further experiments. No permissions are needed during the collection of H. hirsuta samples.
Figure 1. Morphological characters of Helicteres hirsuta. (A, B) – inflorescence, axillary or terminal, cymose 2–5 flowers; (C, D) – flower, short pedicel, calyx tubular to campanulate, densely villous to hirsute, calyx lobes 5, unequal, 5 petals, unequal in length, whitish pink or purplish, darker at base of limb, lower 3 petals slightly longer than upper pair; (E) – fruits, ovoid to ellipsoid, with 5 longitudinal lobes, densely villous, apex short-beaked, black when mature; (F) – underside of leaves, and (G) – upperside of leaves, blades narrowly lanceolate to narrowly oblanceolate, abaxially densely yellow brown puberulent. Photograph credits: Nguyen Hoang Danh (A – G).
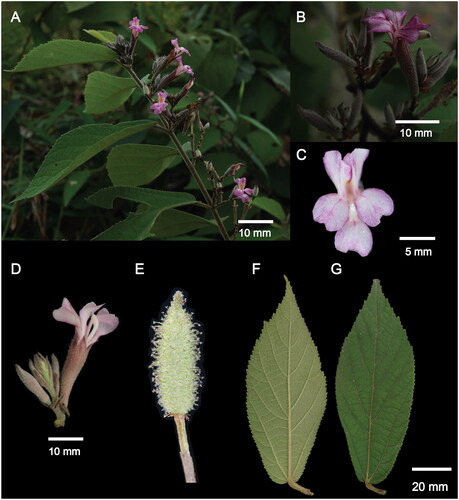
Genomic DNA was extracted from dried leaves using the modified CTAB protocol (Porebski et al. Citation1997). Subsequently, the Genomic Purification Kit was used to purify DNA samples before being used to prepare sequencing libraries using NEBNext Ultra II DNA Library Prep kit (#E7103, New England Biolabs). Approximately 5 Gb of data of paired-end reads (150 bp) was generated using the Illumina MiniSeq platform at KTest Science Co Ltd. (HCMC, Vietnam).
GetOrganelle v1.7.7.0 (Jin et al. Citation2020) and NOVOPlasty v.4.3.1 (Dierckxsens et al. Citation2016) were used to de novo assemble the cp genome of H. hirsuta. Both tools used Reevesia pycnantha Y.Ling 1951 (NC_059003) as a reference sequence. To calculate coverage depth of cp genome, all raw reads were re-mapped to the assembled chloroplast genome and visualized using Geneious Prime v2023.2.1. The annotation process was conducted using GeSeq tool (Tillich et al. Citation2017). The gene content was manually checked using Geneious Prime v2023.2.1. The circular cp genome map was illustrated using OGDRAW v.1.3.1 (Greiner et al. Citation2019).
Fifteen complete cp genomes of Helicteroideae species were downloaded from GenBank database (https://www.ncbi.nlm.nih.gov/), including twelve Reevesia taxa and three Durio species. One Tilioideae species (Tilia oliveri Szyszył. 1890, NC_028590) was used as an outgroup. To reconstruct the phylogenetic tree, 79 protein-coding regions were extracted and aligned using Geneious Prime v2023.2.1. Then, jModeltest 2 was employed to estimate the best model of nucleotide substitution. For reconstructing phylogenetic tree, Bayesian inference (BI) tree was performed by MrBayes v.3.2.7a (Huelsenbeck and Ronquist Citation2001) and maximum likelihood (ML) tree was constructed using IQ-TREE v2.2.2.6 (Nguyen et al. Citation2015). In ML analysis, IQ-TREE was used with 1,000 bootstrap replicates under the TMV + INVGAMMA model (Posada Citation2008). The BI analysis was conducted with 1,000,000 generations. The resulting ML and BI trees were modified and visualized using FigTree v.1.4.4 (http://tree.bio.ed.ac.uk/software/figtree/).
Results
The chloroplast genome of H. hirsuta was 163,404 bp in length with a 71× depth of coverage (Figure S1). The final assembled and annotated H. hirsuta cp genome was uploaded to GenBank under accession number OR806929. The cp genome exhibited a typical quadripartite structure, comprising a pair of inverted repeat (IR) regions (26,291 bp) separated by a large single-copy (LSC) region (91,223 bp) and a small single-copy (SSC) region (19,599 bp) (). The GC content percentages of the LSC, SSC, and IR regions were 34.4%, 31.8%, and 42.3%, respectively. The overall GC content of cp genome was 36.6%.
Figure 2. Chloroplast genome map of Helicteres hirsuta. In the inside circle, the sky-blue line represents a pair of IR regions, and the dark blue line illustrates LSC and SSC regions. An arrow inside the circle indicates transcriptional direction (clockwise) of genes which are inside the circle. An arrow outside the circle indicates transcriptional direction (counterclockwise) of genes which are outside the circle. The light grey line inside the circle represents the AT content, and the dark grey area illustrates the GC content. At the bottom, the genes of different functional groups are illustrated with various colors.
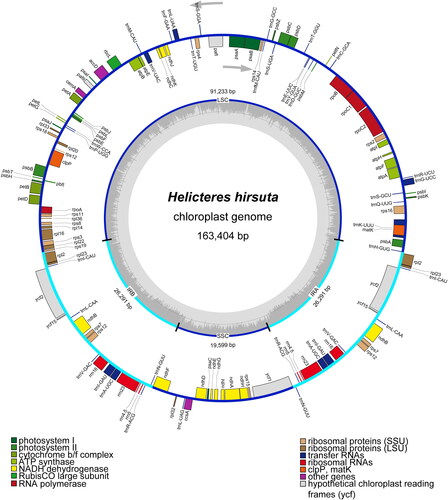
In H. hirsuta cp genome, 113 unique genes were annotated, consisting of 79 protein-coding genes (PCGs), 30 tRNA genes, and four rRNA genes (). Of those 113 genes, 18 genes were duplicated, primarily due to their presence in the IR regions, including seven PCGs, seven tRNA genes, and four rRNA genes. Nine PCGs (i.e. atpF, ndhA, ndhB, petB, petD, rpl2, rpl16, rpoC1, and rps16) comprised single intron, while pafI contained two introns (Figure S2). In addition, six tRNA genes (i.e. trnA-UGC, trnG-UCC, trnI-GAU, trnK-UUU, trnL-UAA, and trnV-UAC) contained single intron. The rps12 gene was a trans-splicing gene (Figure S3). Notably, the clpP gene of H. hirsuta was not composed of two introns and three exons compared to other available cp genomes of Helicteroideae species.
Table 1. List of genes was annotated in the chloroplast genome of Helicteres hirsuta.
Based on current sampling size, both ML and BI trees inferred from 79 protein-coding genes exhibited high resolution with strongly supported values (bootstrap ≥ 99 and posterior probability = 1) (). The monophyly of Helictereae tribe was revealed with high support values. Additionally, H. hirsuta was sister to the clade formed by Reevesia taxa.
Figure 3. Phylogenetic trees were constructed with 79 protein-coding genes of 16 Helicteroideae species cp genomes using ML and BI methods. Node labels indicate the posterior probability and bootstrap values. The scale bar represents nucleotide substitutions per site. Sequence of Tilia oliveri cp genome was used as an outgroup and sequence of Helicteres hirsuta cp genome represented by bold colors. The sequences used for tree construction are as follows: D. oxleyanus (NC_064728, (Wong et al. Citation2022)), D. zibethinus (NC_036829, (Wang et al. Citation2021)), H. hirsuta (OR806929, this study), R. botingensis (NC_054169, (Wang et al. Citation2021)), R. lofouensis (NC_063748, unpublished), R. orbicularifolia (NC_063747, unpublished), R. pubescens (NC_063749, unpublished), R. pycnantha (NC_059003, unpublished), R. rotundifolia (NC_065015, unpublished), R. thyrsoidea (OL701986, (Wang et al. Citation2021)), R. xuefengensis (NC_063750, unpublished), T. oliveri (NC_028590, (Cai et al. Citation2015)), Reevesia glaucophylla (OP868812, Geng et al. Citation2023), Reevesia membranacea (OP868813, Geng et al. Citation2023), Reevesia lognipetiolata (OP868809, Geng et al. Citation2023), Reevesia tomentosa (OP868811, Geng et al. Citation2023), and Durio dulcis (NC_073110, unpublished).
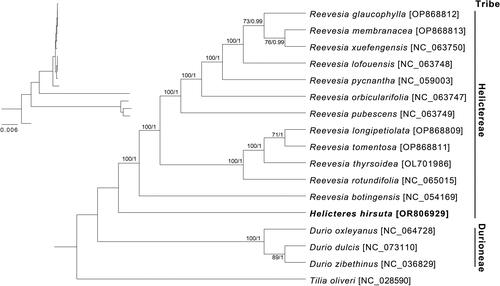
Discussion and conclusion
The cp genome of H. hirsuta was successfully sequenced and assembled in this study. The structure and gene content of cp genome was similar to other published cp genomes in the Malvaceae family (Wang et al. Citation2022; Percy et al. Citation2023; Tan et al. Citation2023). Interestingly, we found a loss of two introns of clpP gene in cp genome of H. hirsuta. Previously, mutations on clpP gene were observed and revealed different intron losses and RNA-editing sites (Williams et al. Citation2019). Additionally, the loss of one or two introns of clpP was reported in species of Fabaceae, Oleaceae, Passifloraceae, and Pinaceae (Lee et al. Citation2007; Dugas et al. Citation2015; Cauz-Santos et al. Citation2017; Ni et al. Citation2017). In Helicterodieae, the loss of two introns of clpP was only found in Helicteres, but not in the other selected Reevesia and Durio species used in this study, suggesting a specific alternation in chloroplast genome of this genus. Phylogenetic analysis revealed a close relationship between Helicteres and Reevesia genera, which was also found in previous studies using nrITS and ndhF sequences (Alverson et al. Citation1999; Nyffeler and Baum Citation2000). However, for elucidating phylogeny among Helicteres and related taxa, more samples within Helicterodieae and Malvaceae should be included. In conclusion, this study provided the first complete cp genome in Helicteres which can be a reference sequence for further studies on chloroplast genomes. Additionally, the current data on chloroplast genome revealed the relationship between Helicteres and related species, which is useful for future phylogenetic studies in the Malvaceae family.
Author contributions
Collected sample, HDKD and HDN; methodology, MTV, HDKD and HDN; experiments, HDN; writing original draft preparation, HDN and HDKD; writing review and editing MTV and HDKD. All the authors have read and agreed to the published version of the manuscript.
Ethical approval
No ethical approval was required for this study because this study includes no human, animal, or endangered plant samples, and the sampling site was not in the natural reserve. No permissions are needed during the collection of Helicteres hirsuta samples.
Supplemental Material
Download JPEG Image (122 KB)Supplemental Material
Download JPEG Image (256.8 KB)Supplemental Material
Download JPEG Image (249.2 KB)Supplemental Material
Download MS Word (12 KB)Disclosure statement
No potential conflict of interest was reported by the author(s).
Data availability statement
The sequence of Helicteres hirsuta chloroplast genome is available in GenBank at https://www.ncbi.nlm.nih.gov/under accession number OR806929. The BioProject, SRA, and Bio-Sample numbers were PRJNA1044269, SRR26928593, and SAMN38378229, respectively.
Additional information
Funding
References
- Alverson WS, Whitlock BA, Nyffeler R, Bayer C, Baum DA. 1999. Phylogeny of the core Malvales: evidence from ndhF sequence data. Am J Bot. 86(10):1474–1486. doi:10.2307/2656928.
- Cai J, Ma PF, Li HT, Li DZ. 2015. Complete plastid genome sequencing of four Tilia species (Malvaceae): a comparative analysis and phylogenetic implications. PLoS One. 10(11):e0142705. doi:10.1371/journal.pone.0142705.
- Cauz-Santos LA, Munhoz CF, Rodde N, Cauet S, Santos AA, Penha HA, Dornelas MC, Varani AM, Oliveira GC, Bergès H, et al. 2017. The chloroplast genome of Passiflora edulis (Passifloraceae) assembled from long sequence reads: structural organization and phylogenomic studies in malpighiales. Front Plant Sci. 8:334. doi:10.3389/fpls.2017.00334.
- Dang VS, Dang MQ, Hoang NS. 2020. Helicteres binhthuanensis V.S.Dang (Malvaceae, Helicteroideae), a new species from southern Vietnam. PhytoKeys. 166(29):87–95. doi:10.3897/phytokeys.166.57647.
- Dierckxsens N, Mardulyn P, Smits G. 2016. NOVOPlasty: de novo assembly of organelle genomes from whole genome data. Nucleic Acids Res. 45(4):e18. doi:10.1093/nar/gkw955.
- Dugas DV, Hernandez D, Koenen EJM, Schwarz E, Straub S, Hughes CE, Jansen RK, Nageswara-Rao M, Staats M, Trujillo JT, et al. 2015. Mimosoid legume plastome evolution: IR expansion, tandem repeat expansions and accelerated rate of evolution in clpP. Sci Rep. 5(1):16958. doi:10.1038/srep16958.
- Geng L-Y, Jiang T-Y, Chen X, Li Q, Ma J-H, Hou W-X, Tang C-Q, Wang Q, Deng Y-F. 2023. Plastome structure, phylogeny and evolution of plastid genes in Reevesia (Helicteroideae, Malvaceae). 28 December 2023, PREPRINT (Version 1) available at Research Square [doi:10.21203/rs.3.rs-3774053/v1].
- Greiner S, Lehwark P, Bock R. 2019. OrganellarGenomeDRAW (OGDRAW) version 1.3.1: expanded toolkit for the graphical visualization of organellar genomes. Nucleic Acids Res. 47(W1):W59–W64. doi:10.1093/nar/gkz238.
- Hieu LT, Thi TTV, Son LL, Nhung NM, Diep HTN, Mechler A, Vo QV. 2021. Phenolic contents and antioxidant activity of Helicteres hirsuta extracts. LOC. 18(2):128–133. doi:10.2174/1570178617999200728205824.
- Hoang NS, Bui DT, Nguyen TNT. 2020. A new species of Helicteres (Malvaceae) from southern Vietnam. Taiwania. 65(3):321–325. doi:10.6165/tai.2020.65.321.
- Huelsenbeck JP, Ronquist F. 2001. MRBAYES: bayesian inference of phylogenetic trees. Bioinformatics. 17(8):754–755. doi:10.1093/bioinformatics/17.8.754.
- Jin JJ, Yu WB, Yang JB, Song Y, DePamphilis CW, Yi TS, Li DZ. 2020. GetOrganelle: a fast and versatile toolkit for accurate de novo assembly of organelle genomes. Genome Biol. 21(1):241. doi:10.1186/s13059-020-02154-5.
- Le DK, Hoang MH. 2020. Triterpenoids isolated from Helicteres hirsuta. J Tech Educ Sci. 2(56):12–16. doi: https://jte.edu.vn/index.php/jte/article/view/170.
- Lee HL, Jansen RK, Chumley TW, Kim KJ. 2007. Gene relocations within chloroplast genomes of Jasminum and Menodora (Oleaceae) are due to multiple, overlapping inversions. Mol Biol Evol. 24(5):1161–1180. doi:10.1093/molbev/msm036.
- Loureiro J d. 1790. Helicteres hirsuta. Flora cochinchinensis : sistens plantas in regno Cochinchina nascentes. Quibus accedunt aliae observatae in Sinensi imperio, Africa Orientali, Indiaeque locis variis. Omnes dispositae secundum systema sexuale Linnaeanum. Labore. Ulyssipone: typis, et expensis academicis. p. 530–531. doi:10.5962/bhl.title.560.
- Nguyen LT, Schmidt HA, Haeseler AV, Minh BQ. 2015. IQ-TREE: a fast and effective stochastic algorithm for estimating maximum-likelihood phylogenies. Mol Biol Evol. 32(1):268–274. doi:10.1093/molbev/msu300.
- Nguyen TT, Kretschmer N, Pferschy-Wenzig E-M, Kunert O, Bauer R. 2019. Triterpenoidal and phenolic compounds isolated from the aerial parts of Helicteres hirsuta and their cytotoxicity on several cancer cell lines. Nat Prod Commun. 14(1):7–10. doi:10.1177/1934578X1901400103.
- Nhat TL, Hong TV, Ngoc TPN, Van SL, Van HC, Huynh AVT, Quoc HN, Hoang DN, Ngoc NT, Tan VP. 2022. Chemical profiles and antibacterial, antioxidant, cytotoxic activities of acetone extract from leaves of Helicteres hirsuta Lour. J Sci Technol - IUH. 52(04):62–69. doi:10.46242/jstiuh.v52i05.4119.
- Ni Z, Ye Y, Bai T, Xu M, Xu LA. 2017. Complete chloroplast genome of Pinus massoniana (Pinaceae): gene rearrangements, loss of ndh genes, and short inverted repeats contraction, expansion. Molecules. 22(9):1528. doi:10.3390/molecules22091528.
- Nyffeler R, Baum DA. 2000. Phylogenetic relationships of the durians (Bombacaceae-Durioneae or/Malvaceae/Helicteroideae/Durioneae) based on chloroplast and nuclear ribosomal DNA sequences. Pl Syst Evol. 224(1-2):55–82. doi:10.1007/BF00985266.
- Percy DM, Sveinsson S, Ponomarev A, Yang JY, Cronk QCB. 2023. Chloroplast markers for the Malvaceae and the plastome of Henderson’s checkermallow (Sidalcea hendersonii S.Wats.), a rare plant from the Pacific Northwest. BMC Res Notes. 16(1):87. doi:10.1186/s13104-023-06357-4.
- Pham HNT, Vuong QV, Bowyer MC, Scarlett CJ. 2017. Phytochemical profiles and antioxidant capacity of the crude extracts, aqueous- and saponin-enriched butanol fractions of Helicteres hirsuta Lour. leaves and stems. Chem Pap. 71(11):2233–2242. doi:10.1007/s11696-017-0216-6.
- Porebski S, Bailey LG, Baum BR. 1997. Modification of a CTAB DNA extraction protocol for plants containing high polysaccharide and polyphenol components. Plant Mol Biol Rep. 15(1):8–15. doi:10.1007/BF02772108.
- Posada D. 2008. jModelTest: phylogenetic model averaging. Mol Biol Evol. 25(7):1253–1256. doi:10.1093/molbev/msn083.
- Quang DN, Pham CT, Le LTK, Ta QN, Dang NK, Hoang NT, Pham DH. 2020. Cytotoxic constituents from Helicteres hirsuta collected in Vietnam. Nat Prod Res. 34(4):585–589. doi:10.1080/14786419.2018.1490907.
- Tan F, Banerjee AK, Deng J, Feng H, Feng Y, Shu Y, Wang J. 2023. Characterization of the complete chloroplast genome of Firmiana hainanensis (Malvaceae), an endemic and vulnerable tree species of China. Mitochondrial DNA B Resour. 8(1):57–60. doi:10.1080/23802359.2022.2160669.
- Thang Hoang D, Hien Truong TT, Viet Duc N, Anh Hoang LT, Do TT, Vinh LB, Young Yang S, Dan G, Tuan Anh L. 2021. Hepatoprotective effects of extract of Helicteres hirsuta Lour. on liver fibrosis induced by carbon tetrachloride in rats. Appl Sci. 11(18):8758. doi:10.3390/app11188758.
- Tillich M, Lehwark P, Pellizzer T, Ulbricht-Jones ES, Fischer A, Bock R, Greiner S. 2017. GeSeq – versatile and accurate annotation of organelle genomes. Nucleic Acids Res. 45(W1):W6–W11. doi:10.1093/nar/gkx391.
- Tra NT, Ha NTT, Cham BT, Anh LTT, Yen LTH, Giang BL, Anh DTT, Tuyen NV, Kiem PV. 2019. Phenolic and flavonoid compounds isolated from ethyl acetate fraction of Helicteres hirsuta Lour. Nat Prod Commun. 14(6):1934578X1985881. doi:10.1177/1934578X198588.
- Dang VS, Truong BV, Hoang NS, Nguyen VT, Nguyen QB, Pham QT, Le VT, Kieu CN, Bui VH, Naiki A. 2022. Two new species of genus Helicteres (Helicteroideae, Malvaceae) from Vietnam. AJB. 44(4):123–132. doi:10.15625/2615-9023/17544.
- Turner IM. 1995. A catalogue of the vascular plants of Malaya. Gardens’ Bulletin, Singapore. National Parks Board. 47(2): 347–655.
- Wang JH, Moore MJ, Wang H, Zhu Z, Wang HF. 2021. Plastome evolution and phylogenetic relationships among Malvaceae subfamilies. Gene. 765(10):145103. doi:10.1016/j.gene.2020.145103.
- Wang S, Ding S, Deng R, Shi W, Zhang H. 2022. The complete chloroplast genome of Callianthe picta (Malvaceae.)Mitochondrial DNA B Resour. 7(1):10–11. doi:10.1080/23802359.2021.2005487.
- Williams AM, Friso G, Van Wijk KJ, Sloan DB. 2019. Extreme variation in rates of evolution in the plastid Clp protease complex. Plant J. 98(2):243–259. doi:10.1111/tpj.14208.
- Wong XJ, Law D, Wang ZF, Ramaiya SD, Lee SY. 2022. The complete chloroplast genome sequence of Durio oxleyanus (Malvaceae) and its phylogenetic position. Mitochondrial DNA B Resour. 7(9):1709–1712. doi:10.1080/23802359.2022.2123256.
- Wu L, Wu M, Cui N, Xiang L, Li Y, Li X, Chen S. 2021. Plant super-barcode: a case study on genome-based identification for closely related species of Fritillaria. Chin Med. 16(1):52. doi:10.1186/s13020-021-00460-z.
- Zhang W, Sun Y, Liu J, Xu C, Zou X, Chen X, Liu Y, Wu P, Yang X, Zhou S. 2021. DNA barcoding of Oryza: conventional, specific, and super barcodes. Plant Mol Biol. 105(3):215–228. doi:10.1007/s11103-020-01054-3.