Abstract
The complete mitochondrial genome of Squamanita imbachii I. Saar, is unveiled in this research for the first time. It covers 76,643 base pairs (bp) and exhibits a guanine-cytosine (GC) content of 23%. The genome includes 14 conserved protein-coding genes, 1 DNA polymerase gene, 2 ribosomal RNA gene (RNS and RNL), 25 transfer RNA (tRNA) genes and 18 open reading frames (ORFs). Phylogenetic analysis, utilizing a mitochondrial gene dataset from 15 taxa across seven families within the Agaricales order, was conducted employing the maximum-likelihood (ML) approach. This analysis identified a close phylogenetic relationship between S. imbachii and Floccularia luteovirens (Alb. & Schwein.) Pouzar 1957, positioning both within the Squamanitaceae family.
Introduction
Squamanita is a genus in the family Squamanitaceae (Vizzini et al. Citation2019; Kalichman et al. Citation2020; Liu et al. Citation2021), which may represent one of the most unique basidiomycetes fungi in the world as nearly all the species are parasitic on other living fungi. Saar et al. (Citation2022) split Squamanita into two monophyletic groups, viz. Squamanita and Dissoderma (A.H. Sm. & Singer) Singer 1973. Fourteen and nine species are accepted in Squamanita and Dissoderma worldwide, respectively (Species Fungorum Citation2024).
Squamanita imbachii has been documented in Europe and North America (Liu et al. Citation2021; Saar et al. Citation2022). Liu et al. (Citation2021) sequenced a specimen (HKAS107325A) collected from Liguria, Italy, resulting in the generation of four genes (ITS: MW258856; LSU: MW258908; TEF1-α: MW324508; 18S: MW258935 & MW258886) and noted that this species acts as a parasite on Amanita excelsa species complex (Fr.) Bertill. 1866. Saar et al. (Citation2022) confirmed the identity of HKAS107325A as S. imbachii. In this study, we used this dried specimen archived in the Herbarium of Cryptogams, Kunming Institute of Botany, Chinese Academy of Sciences (http://www.kib.ac.cn/) ().
Figure 1. Image of Squamanita imbachii (HKAS107325A) sequenced in this work Liu et al. (Citation2021).

Materials and methods
Genomic DNA extraction and sequencing
The CTAB method (Doyle and Doyle Citation1987) was utilized to extract the entire genomic DNA from the desiccated S. imbachii specimen. Standard protocols were followed for whole-genome sequencing on an Illumina sequencing platform (HiSeq PE150). Paired-end libraries with 150 bp were created, resulting in approximately 6 GB of raw data.
Assembly and annotation
The assembly process was carried out employing GetOrganelle v. 1.7.5 with default settings (Jin et al. Citation2020). Initial annotation was automated using the MFannot tool (Beck and Lang Citation2010), relying on genetic code 4 (Mold, Protozoan, and Coelenterate Mitochondrial genetic code). Verification of rRNA and tRNA genes was performed using RNAweasel (Beck and Lang Citation2010) and tRNAscan-SE 2.0 (Lowe and Chan Citation2016), respectively. To ensure accurate annotation, the intron and exon boundaries of Protein-Coding Genes (PCGs) were individually validated by aligning gene sequences with homologous alleles sequences (obtained via Extract Regions in Geneious Prime) from previously annotated mitogenomes of Squamanitaceae using the MAFFT program in Geneious Prime 2020.0.3 (BioMatters, Ltd., Auckland, New Zealand). OGDraw v. 1.2 (Lohse et al. Citation2013) was employed to generate a physical circular map of S. imbachii. Sequencing depth and coverage was calculated following the procedure outlined in Ni et al. (Citation2023).
Phylogenetic analysis
We constructed a phylogenetic tree for 15 Agaricineae species to validate the phylogenetic status of S. imbachii. Amanita phalloides (Vaill. ex Fr.) Link 1833 and A. basii Guzmán & Ram. Guill. 2001 from Pluteineae were selected as the outgroup. Fifteen common conserved PCGs sequences were extracted, aligned, and concatenated using Geneious Prime 2020.0.3. Partitioned substitution model (ATP6: LG; ATP8: MTZOA; ATP9: HIVB; COB: MTZOA; COX1: CPREV; COX2: MTZOA; COX3: MTZOA; NAD1: MTZOA; NAD2: LG; NAD3: MTZOA; NAD4L: LG; NAD4: LG; NAD5: LG; NAD6: CPREV; RPS3: CPREV) evaluation and phylogenetic tree construction for maximum likelihood (ML) analysis were performed using RAxML.8.2.12 (Stamatakis Citation2006).
Results
The complete mitochondrial genome sequence of S. imbachii has been deposited in GenBank (accession no. PP417754; ). The circular genome (76,643 bp) comprised 14 conserved protein-coding genes (PCGs), 2 rRNA gene (RNS and RNL), 25 transfer RNA (tRNA) genes, 1 DNApolymerase gene (likely a pseudo-gene, introduced by plasmid integration) and 18 open reading frames (ORFs). Among these, 13 ORFs originate from the COX1 and COB genes, which are intron-encoded. Within the COX1 gene, eight out of nine ORFs belong to the LAGLIDADG type, while one belongs to the GIY type. Among the introns the involved encoded ORFs, seven belong to group I, one belongs to group II, and one remains unassigned. Regarding the COB gene, two out of four ORFs are classified as LAGLIDADG type, one as GIY type, and three out of four introns involved encoded ORFs belong to group I, while one belongs to group II. Becides, RNL gene also include two introns belong to group I and group II, respectively. The 14 conserved PCGs respectively encoded the seven ubiquinone reductase subunits of NADH (NAD1, NAD2, NAD3, NAD4, NAD4L, NAD5, and NAD6), three cytochrome oxidase subunits (COX1, COX2, and COX3), three ATP synthase subunits (ATP6, ATP8, and ATP9), and apocytochrome b (COB). The 25 tRNA genes (tRNAAla(TGC), tRNACys(GCA), tRNAAsp(GTC), tRNAGlu(TTC), tRNAPhe(GAA), tRNAGly(TCC), tRNAHis(GTG), tRNAIle(GAT), tRNALys(TTT), tRNALeu(TAA), tRNALeu(TAG), tRNAMet(CAT), tRNAAsn(GTT), tRNAPro(TGG), tRNAGln(TTG), tRNAArg(TCG), tRNAArg(TCT), tRNASer(GCT), tRNASer(TGA), tRNAThr(TGT), tRNAVal(TAC), tRNATrp(CCA), tRNATyr(GTA) ranged from 71 to 85 bp, and covered all 20 standard amino acids. The maximum, minimum and average sequencing depth was 8027, 6110, and 7914.37, respectively (Figure S1). The overall base composition is as follows: 37.81% A, 38.84% T, 11.30% C, and 12.05% G, with a GC content of 23%.
Figure 2. Circular map of the mitochondrial genomes of S. imbachii. Genes are represented by different colored blocks. Colored blocks outside each ring indicate that the genes are on the direct strand, while colored blocks within the ring indicate that the genes are located on the reverse strand. The inner grayscale bar graph shows the GC content of the mitochondrial sequences. The circle inside the GC content graph marks the 50% threshold. Label intron-containing genes with *.

Squamanita imbachii and F. luteovirens exhibited a close relationship in the phylogenetic tree and both of them belonged to Squamanitaceae ().
Figure 3. Phylogenetic relationships among 15 species based on concatenated mitochondrial protein-coding genes (PCGs) using partitioned models. The 15 PCGs included subunits of the respiratory chain complexes (COB, COX1, COX2, and COX3), ATPase subunits (ATP6, ATP8, and ATP9), NADH: quinone reductase subunits (NAD1, NAD2, NAD3, NAD4, NAD4L, NAD5, and NAD6), and ribosomal protein (RPS3). Support values are shown at the corresponding nodes. Accession numbers of mitochondrial sequences used in the phylogenetic analysis are listed after the species names.
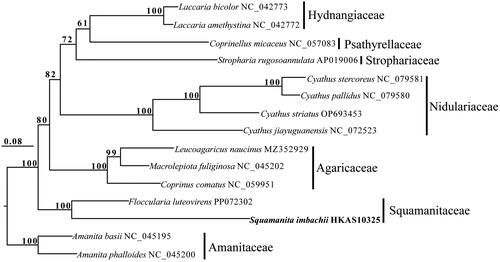
Discussion and conclusion
In this study, we conducted sequencing and characterization of the first mitochondrial genome from the rare genus Squamanita. Phylogenetic analysis revealed that S. imbachii and F. luteovirens are affiliated with Squamanitaceae based on mitochondrial protein-coding gene, a finding consistent with Liu et al. (Citation2021) that was based on multiple nuclear genes. Notably, our current phylogenetic tree () reveals that the family Squamanitaceae does not cluster with Nidulariaceae. Given the maternally inherited nature of the mitochondrial genome, evolutionary histories infering based on mitochondrial genomes often differ from those based on biparentally inherited nuclear genomes. Further sequencing and the generation of additional mitochondrial genomes from a broader range of representative taxa within Suborder Pluteineae would enhance our understanding of the evolutionary relationships among these remarkable mycoparasitic basidiomycetes fungi.
Ethical approval
In accordance with guidelines and regulations provided by Kunming Institute of Botany, Chinese Academy of Sciences School of Sciences, Yunnan, China and Mae Fah Luang University, Chiang Rai, Thailand and the local government agency, DNA extraction of the dried specimen did not require any ethical approval or other relevant permissions from a national or local agency.
Author contributions
Chitrabhanu S. Bhunjun, Fu-Qiang Yu and Jian-Wei Liu designed the study. Jian-Wei Liu analyzed the data. Jian-Wei Liu and Thatsanee Luangharn wrote the manuscript while Chitrabhanu S. Bhunjun and Fuqiang Yu revised the manuscript. All authors agree to be accountable for all aspects of the work.
Disclosure statement
No potential conflicts of interest were reported by the authors.
Data availability statement
The genome sequence data that support the findings of this study are openly available in GenBank of NCBI at https://dataview.ncbi.nlm.nih.gov/under the accession no. PP417754. The associated BioProject, SRA, and Bio-Sample numbers are PRJNA811355, SRP361919, and SAMN40372582, respectively.
Additional information
Funding
References
- Beck N, Lang B. 2010. MFannot, organelle genome annotation Websever. Montréal QC: Université de Montréal.
- Doyle JJ, Doyle JL. 1987. A rapid DNA isolation procedure for small quantities of fresh leaf tissue. Phytochem Bull. 19:11–15.
- Jin JJ, Yu WB, Yang JB, Song Y, dePamphilis CW, Yi TS, Li DZ. 2020. GetOrganelle: a fast and versatile toolkit for accurate de novo assembly of organelle genomes. Genome Biol. 21(1):241. doi:10.1186/s13059-020-02154-5.
- Kalichman J, Kirk PM, Matheny PB. 2020. A compendium of generic names of agarics and Agaricales. Taxon. 69(3):425–447. doi:10.1002/tax.12240.
- Liu JW, Ge ZW, Horak E, Vizzini A, Halling RE, Pan CL, Yang ZL. 2021. Squamanitaceae and three new species of Squamanita parasitic on Amanita basidiomes. IMA Fungus. 12(1):4. doi:10.1186/s43008-021-00057-z.
- Lohse M, Drechsel O, Kahlau S, Bock R. 2013. OrganellarGenomeDRAW–a suite oftools for generating physical maps of plastid and mitochondrial genomesand visualizing expression data sets. Nucleic Acids Res. 41(Web Server issue):W575–W581. doi:10.1093/nar/gkt289.
- Lowe TM, Chan PP. 2016. tRNAscan-SE On-line: integrating search and context for analysis of transfer rna genes. Nucleic Acids Res. 44(W1):W54–W57. doi:10.1093/nar/gkw413.
- Ni Y, Li J, Zhang C, Liu C. 2023. Generating sequencing depth and coverage map for organelle genomes. protocols.io. doi:10.17504/protocols.io.4r3l27jkxg1y/v1.
- Saar I, Thorn RG, Nagasawa E, Henkel TW, Cooper JA. 2022. A phylogenetic overview of Squamanita, with descriptions of nine new species and four new combinations. Mycologia. 114(4):769–797. doi:10.1080/00275514.2022.2059639.
- Species Fungorum. 2024. http://www.speciesfungorum.org/.
- Stamatakis A. 2006. RAxML-VI-HPC: maximum likelihood-based phylogenetic analyses with thousands of taxa and mixed models. Bioinformatics. 22(21):2688–2690. doi:10.1093/bioinformatics/btl446.
- Vizzini A, Consiglio G, Marchetti M. 2019. Mythicomycetaceae fam. nov. (Agaricineae, Agaricales) for accommodating the genera Mythicomyces and Stagnicola, and Simocybe parvispora reconsidered. Fungal Syst Evol. 1(1):41–56. doi:10.3114/fuse.2019.03.05.