Abstract
This study is the first to sequence the complete mitochondrial genome (mitogenome) of Perforatus perforatus Bruguière, 1789 (Balanomorpha: Balanidae). The 15,536-bp long P. perforatus mitogenome contained a typical set of animal mitochondrial genes, along with one control region. The P. perforatus mitogenome had an inverted gene block (trnP-ND4L-ND4-trnH-ND5-trnF) between trnS(gct) and trnT. This inverted gene block had been detected six species in three subfamilies of the Balanidae family (Balaninae, Acastinae and Megabalaninae), but our results show that it is also present in Concavinae, in which P. perforatus is included. The phylogenetic tree based on the concatenated sequences of the 13 protein-coding genes and two rRNA genes showed that P. perforatus is closely associated with Acasta sulcate and Balanus trigonus within Balanidae.
Introduction
As international trade and human movement increase, the emergence of invasive organisms is also increasing. Marine invasive species can disrupt the ecosystem in the given areas by affecting the ecological succession (Gallardo et al. Citation2016) and ecological pyramid (Giakoumi et al. Citation2019; Kim et al. Citation2020). Particularly, invasive barnacle species disrupt local marine ecosystems by competing for space and resources with the native species (Carlton and Newman Citation2009). Moreover, they can cause economic damage to marine infrastructure facilities when population size increases (Foster and Willan Citation1979; Kim et al. Citation2019). The European common barnacle, Perforatus perforatus, was frequently observed in European waters, but was first discovered in the ports of Guryongpo, Yangpo, and Gampo in 2006 (Kim and Hong Citation2010), and is now frequently found in certain ports and bays in the East and South Seas of Korea (Choi et al. Citation2013; Kim et al. Citation2020). Currently, 52 species of barnacles have been reported in Korea (National Institute of Biological Resources Citation2022), but only 11 species have had their mitochondrial genomes (mitogenomes) reported. In this study, we present the first complete mitogenome of P. perforatus, covering both the species and its subfamily, Concavinae. Additionally, we conducted a phylogenetic analysis within Balanoidea, a barnacle superfamily. The complete mitogenome sequence of the species will be useful for the understanding of evolutionary characteristics of the species and families in the superfamily Balanoidea and subsequent population-level studies to illustrate expansion characteristics of the species.
Materials and methods
In 2021, an individual of P. perforatus was collected from Mulchi-port, Yangyanggun, Gangwondo Province, Korea (; 38°9’23” N, 128°36’32” E) and was subsequently identified by one of our authors (Hyun Kyong Kim) using several diagnostic characters: parietes conical; dull purple or pinkish; radii white and narrow; scutum triangular; occludent margin straight; articular ridge distinct; and tergum with distinct growth lines and spur longer than wide (Kim Citation2019). DNA was extracted from a portion of the whole specimen using the DNeasy Blood & Tissue Kit (Qiagen, Germany). The voucher specimen and leftover DNA were deposited at Honam National Institute of Biological Resources in Korea under the voucher number HNIBRIV1595 (http://en.hnibr.re.kr/; contact: Hyun Kyong Kim, [email protected]). Library was prepared using the TruSeq Nano DNA Kit (Illumina, USA) and sequencing was performed on the HiSeq 2500 platform (Illumina, USA), generating 51,828,270 reads. The de novo assembly was achieved with SPAdes 3.13.0 (Bankevich et al. Citation2012), under the condition of ‘-cov-cutoff auto’ and produced a contig of 15,536 bp with the sequencing depth of 282,505 (Figure S1). Circularity of the mitogenome was further validated with MitoZ v3.4. (Meng et al. Citation2019), a tool designed for mitochondrial DNA analysis.
Figure 1. Image of Perforatus perforatus collected from Mulchi-port, Yangyanggun, Gangwondo Province, Korea (38°9’20” N, 128°36’35” E). The photo was taken by Hyun Kyong Kim with a digital camera (model 5D mark IV; canon, Tokyo, Japan), and produced with helicon focus software (scale bar: 5 mm).
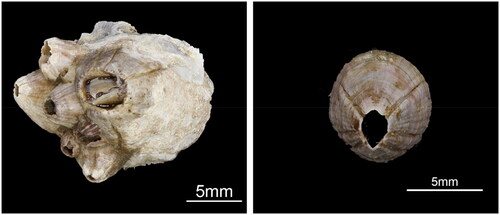
Gene annotation was conducted via the MITOS Web Server (Bernt et al. Citation2013). For improved annotation, mitogenome sequences of all species in the Balanidae family registered in GenBank were downloaded and aligned together with P. perforatus. Annotation of P. perforatus was performed by following the procedure recommended in Cameron (Citation2014). The circular representation of the mitochondrial genome was created using GenomeVx, an online tool for mitochondrial visualization (Conant and Wolfe Citation2008). For the phylogenetic analysis, 15 species registered as of February 2023 were selected using NCBI blastn with the P. perforatus mitogenome as the query, employing the following settings: TaxID limited to the superfamily Balanoidea (TaxID 2899671), sequence length ranging from 14,000 to 17,000, sequence similarity threshold set above 80%, and number of sequences limited to one per species.
Two Chthamaloidea species (Eochionelasmus coreana and E. ohtai) were used as the outgroups. We aligned each protein-coding gene (PCG) using RevTrans 2.0 (Wernersson and Pedersen Citation2003) and each rRNA using MAFFT 7.245 (Katoh and Standley Citation2013). Poorly aligned and highly divergent sequences were eliminated using Gblocks 0.91b (Castresana Citation2000) and then all sequences were concatenated. Phylogenetic analysis was performed via the maximum likelihood (ML) method with 1,000 bootstrap replications (Felsenstein Citation1985) using CIPRES Portal 3.1 (Miller et al. Citation2010), based on the TVM + I + G model, which was determined using the jModelTest (Posada Citation2008). To ensure the robustness of our phylogenetic results against potential saturation effects, we performed a saturation test for each gene using DAMBE software (Table S1; Xia and Lemey Citation2009).
Results
The complete P. perforatus mitogenome was 15,536-bp long, encoding 13 PCGs, two rRNA genes, and 22 tRNA genes, along with a control region (). The start codons for 12 PCGs are ATN, whereas ND4L started with GTG. TAA was the most common stop codon, whereas TAG was present in CYTB only, and incomplete T codons were present in four PCGs (COX3, ND3, ND4, and ND4L). The ND1 gene, five tRNA genes (trnK, trnQ, trnC, trnL(tag), and trnV), and two rRNA genes (rrnL and rrnS) were located on the light strand, whereas the remaining 29 genes (12 PCGs and 17 tRNA genes) were located on the heavy strand. The A/T content was 70.0% in both PCGs and tRNA genes, 75.6% in rrnL gene, 68.8% in rrnS genes, 80.5% in the control region, and 71.1% in the whole genome (Table S2). P. perforatus exhibits an inverted gene block (trnP-ND4L-ND4-trnH-ND5-trnF) between trnS(gct) and trnT, identically to all species in Megabalaninae, Acastinae, and only Balanus trigonus in Balaninae, within the Balanidae family.
Figure 2. Mitochondrial genome map of perforatus perforatus obtained by the GenomeVx tool. tRNAs are abbreviated by the one-letter code of the corresponding amino acid with the anticodon within the parenthesis. Gene names located on the outside of the large circle are encoded on the heavy strand (5’-3’ direction) excluding control region, while gene names located inside are encoded on the light strand (3’-5’ direction). Color codes: green for PCGs, blue for tRNAs, orange for rRNAs, and white for control region.
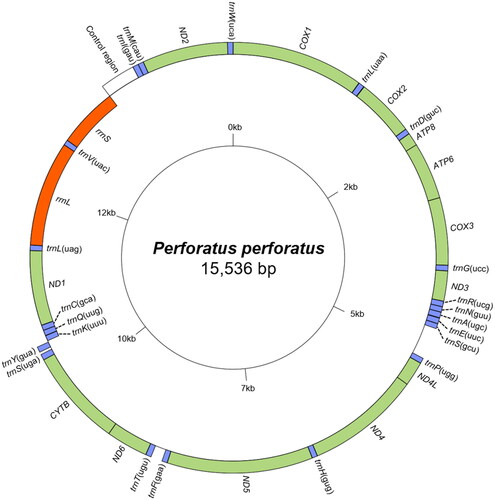
A non-monophyletic Balanidae was notably observed (). Pyrgomatidae, which are represented by two species (Pyrgopsella youngi and Nobia grandis) interrupted the monophyly of Balanidae, forming Pyrgomatidae and the subfamily Archaeobalaninae in Balanidae the sister group, with relatively higher nodal support (Bootstrap Support (BS) = 88%). P. perforatus, representing the Concavinae, was identified as a sister group to the Acasta sulcate and Balanus trigonus, but nodal support for the sister relationship was lower (BS = 45%).
Figure 3. The phylogenetic tree of two families in balanoidea (Balanidae and pyrgomatidae) within balanoidea. The tree was obtained using concatenated nucleotide sequences of 13 protein-coding genes and two rRNAs using the maximum likelihood method. The numbers at each node indicate the bootstrap values. The scale bar indicates the number of substitutions per site. Two chionelasmatidae species (Eochionelasmus coreana and E. ohtai) in chthamaloidea were used as outgroups. Red dots indicate the species with an inverted sequence block (trnP-ND4L-ND4-trnH-ND5-trnF) between trnS(gct) and trnT. The following sequences were used: Acasta sulcate KJ754818 (Unpublished); Balanus trigonus MW646099 (Liu et al. Citation2021); Perforatus perforatus OR327030 (This study); Megabalanus tintinnabulum MN481499 (Feng et al. Citation2019); Megabalanus ajax KF501046 (Shen et al. Citation2016); Megabalanus volcano AB167539 (Unpublished); Megabalanus coccopoma OK631889 (Zhang et al. Citation2023); Semibalanus cariosus MT528637 (Nunez et al. Citation2021); Semibalanus balanoides MT528636 (Nunez et al. Citation2021); Balanus balanus KM660676 (Shen et al. Citation2016); Pyrgopsella youngi MN615273 (Unpublished); Nobia grandis KF720334 (Shen et al. Citation2016); Armatobalanus allium KJ754817 (Unpublished); Amphibalanus amphitrite KF493890 (Shen et al. Citation2015); Fistulobalanus albicostatus MK617531 (Song et al. Citation2020); Striatobalanus amaryllis KF493890 (Tsang et al. Citation2015); Eochionelasmus coreana MT491209 (Lee et al. Citation2021); and Eochionelasmus ohtai MF939636 (Kim et al. Citation2017).
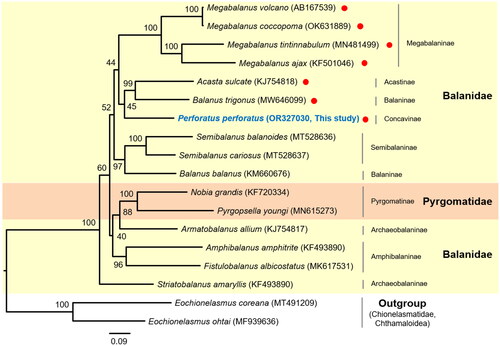
Discussion and conclusion
We assembled and annotated the mitogenome sequence of P. perforatus for the first time. A non-monophyletic Balanidae detected in this study has also been found in previous studies using mitogenome sequences (Bae et al. Citation2021; Ji et al. Citation2021; Mao et al. Citation2021, Citation2024). Moreover, phylogenetic analysis using several nuclear gene fragments, along with mitochondrial COX1 gene has also shown non-monophyletic Balanidae (Pérez-Losada et al. Citation2014). Thus, our research and previous studies suggest the necessity for further investigation into taxonomic classifications and further scrutinized phylogenetic analysis using a diverse type of molecular markers for Balanidae and allied groups.
Our findings highlight the presence of an inverted gene block also in the subfamily Concavinae, along with six species belonging to three subfamilies in the family Balanidae to which current P. perforatus is included. Recently, Zhang et al. (Citation2023) scrutinized mitogenome arrangement in Balanomorpha including Balanidae to which Concavinae is included and found a diverse type of gene rearrangement within Balanomorpha. However, phylogenetic tree did not exclusively reflect these types of rearrangement (Zhang et al. Citation2023). Thus, further extensive mitogenome data could be required to improve our understanding on the genomic rearrangements and evolutionary relationships.
Current mitogenome sequence can be used for subsequent mitogenome-based molecular phylogenetic study along with available public data. Moreover, the sequences can be used for the tracing the origin of invasive species (Farrington et al. Citation2015), population genetics (Jeong et al. Citation2021), and the development of environmental DNA detection markers (Jerde et al. Citation2011).
Ethics statement
This study did not involve human participants or animals. This research does not involve experiments that require ethical review. This species is not endangered or collected in nature reserves, so it did not need any specific permissions.
Authors’ contributions
Conceptualization, JSJ, HKK, and IK; field work, HKK; analysis and interpretation of the data, JSJ, H-SH and JSP; writing—original draft preparation, JSJ, HKK, H-SH and IK; writing—review and editing, JSP, H-SH and IK; supervision, IK; project administration, JSJ. All authors approved the final version to be published and agree to be accountable for all aspects of the work.
Supplemental Material
Download PDF (668.5 KB)Disclosure statement
The authors report there are no competing interests to declare.
Data availability statement
The genome sequence data that support the findings of this study are openly available in the GenBank of the National Center for Biotechnology Information at https://www.ncbi.nlm.nih.gov, under the accession number OR327030. The associated BioProject, SRA, and Bio-Sample numbers: PRJNA1057449, SRR27587530, and SAMN39129815, respectively.
Additional information
Funding
References
- Bae S, Kim P, Yi CH. 2021. The complete mitochondrial genome of Balanus trigonus (Thecostraca, Balanomorpha, Balanidae) from South Korea. Mitochondrial DNA B Resour. 6(9):2736–2738. doi:10.1080/23802359.2021.1966335.
- Bankevich A, Nurk S, Antipov D, Gurevich AA, Dvorkin M, Kulikov AS, Lesin VM, Nikolenko SI, Pham S, Prjibelski AD, et al. 2012. SPAdes: a new genome assembly algorithm and its applications to single-cell sequencing. J Comput Biol. 19(5):455–477. doi:10.1089/cmb.2012.0021.
- Bernt M, Donath A, Jühling F, Externbrink F, Florentz C, Fritzsch G, Pütz J, Middendorf M, Stadler PF. 2013. MITOS: improved de novo metazoan mitochondrial genome annotation. Mol Phylogenet Evol. 69(2):313–319. doi:10.1016/j.ympev.2012.08.023.
- Cameron S. 2014. How to sequence and annotate insect mitochondrial genomes for systematic and comparative genomics research. Syst Entomol. 39(3):400–411. doi:10.1111/syen.12071.
- Carlton JT, Newman WA. 2009. Reply to Clare and Høeg 2008. Balanus amphitrite or Amphibalanus amphitrite? A note on barnacle nomenclature. Biofouling. 25(1):77–80. doi:10.1080/08927010701830194.
- Castresana J. 2000. Selection of conserved blocks from multiple alignments for their use in phylogenetic analysis. Mol Biol Evol. 17(4):540–552. doi:10.1093/oxfordjournals.molbev.a026334.
- Choi KH, Choi HW, Kim IH, Hong JS. 2013. Predicting the Invasion Pathway of Balanus perforatus in Korean Seawaters. Ocean Polar Res. 35(1):63–68. doi:10.4217/OPR.2013.35.1.063.
- Conant GC, Wolfe KH. 2008. GenomeVx: simple web-based creation of editable circular chromosome maps. Bioinformatics. 24(6):861–862. doi:10.1093/bioinformatics/btm598.
- Farrington HL, Edwards CE, Guan X, Carr MR, Baerwaldt K, Lance RF. 2015. Mitochondrial genome sequencing and development of genetic markers for the detection of DNA of invasive bighead and silver carp (Hypophthalmichthys nobilis and H. molitrix) in environmental water samples from the United States. PLoS One. 10(2):e0117803. doi:10.1371/journal.pone.0117803.
- Felsenstein J. 1985. Confidence limits on phylogenies: an approach using the bootstrap. Evolution. 39(4):783–791. doi:10.1111/j.1558-5646.1985.tb00420.x.
- Feng M, Lin S, Wang C, Sun D, Zhou Y, Bi Y, Xu K. 2019. The first mitochondrial genome of Megabalanus tintinnabulum (Sessilia: Balanidae) from China: phylogeny within Cirripedia based on mitochondrial genes. Mitochondrial DNA B Resour. 4(2):4016–4018. doi:10.1080/23802359.2019.1688104.
- Foster BA, Willan RC. 1979. Foreign barnacles transported to New Zealand on an oil platform. N Z J Mar Freshw Res. 13(1):143–149. doi:10.1080/00288330.1979.9515788.
- Gallardo B, Clavero M, Sánche MI, Vilà M. 2016. Global ecological impacts of invasive species in aquatic ecosystems. Glob Chang Biol. 22(1):151–163. doi:10.1111/gcb.13004.
- Giakoumi S, Katsanevakis S, Albano PG, Azzurro E, Cardoso AC, Cebrian E, Deidun A, Edelist D, Francour P, Jimenez C, et al. 2019. Management priorities for marine invasive species. Sci Total Environ. 688:976–982. doi:10.1016/j.scitotenv.2019.06.282.
- Jeong JS, Kim MJ, Park JS, Lee KH, Jo YH, Takahashi JI, Choi YS, Kim I. 2021. Tracing the invasion characteristics of the yellow-legged hornet, Vespa velutina nigrithorax (Hymenoptera: vespidae), in Korea using newly detected variable mitochondrial DNA sequences. J Asia-Pac Entomol. 24(2):135–147. doi:10.1016/j.aspen.2021.03.004.
- Jerde CL, Mahon AR, Chadderton WL, Lodge DM. 2011. “Sight-unseen” detection of rare aquatic species using environmental DNA. Conserv Lett. 4(2):150–157. doi:10.1111/j.1755-263X.2010.00158.x.
- Ji N, Ge T, Mao S, Zhang M, Mao N, Cai Y, Shen X. 2021. The first mitochondrial genome of Tetraclita kuroshioensis (Crustacea: sessilia) from China: insight into the phylogeny within Cirripedia. Mitochondrial DNA B Resour. 6(3):988–989. doi:10.1080/23802359.2021.1891984.
- Katoh K, Standley DM. 2013. MAFFT Multiple Sequence Alignment Software Version 7: improvements in performance and usability. Mol Biol Evol. 30(4):772–780. doi:10.1093/molbev/mst010.
- Kim S-J, Lee W-K, Kim R-O, Ju S-J. 2017. Complete mitochondrial genome of the hydrothermal vent barnacle Eochionelasmus ohtai (Cirripedia, Thoracica). Mitochondrial DNA B Resour. 3(1):46–47. doi:10.1080/23802359.2017.1419089.
- Kim HK, Chan BKK, Lee SK, Kim W. 2020. Biogeography of intertidal and subtidal native and invasive barnacles in Korea in relation to oceanographic current ecoregions and global climatic changes. J Mar Biol Ass. 100(7):1079–1091. doi:10.1017/S0025315420001009.
- Kim HK, Lee SK, Bs M, Kim W. 2019. Report on the current status of the distribution of invasive barnacles in marine national park areas of Korea. J National Park Res. 10(2):249–257.
- Kim HK. 2019. Taxonomic study of the thoracican barnacles (Crustacea: thecostraca: thoracica) in Korean waters: diversity, host specificity, distribution. Seoul, Repubic of Korea: Seoul naional Univ.
- Kim IH, Hong JS. 2010. Introduction of the European common barnacle Balanus perforatus Brugiére (Crustacea, Cirripedia) into Korean waters. In: 17th International Conference on Aquatic Invasive Species. San Diego, CA, USA.
- Lee W-K, Chan BKK, Ju S-J, Kim D, Kim S-J. 2021. The mitochondrial genome of hydrothermal vent barnacle Eochionelasmus coreana (Cirripedia: Thoracica) from the Indian Ocean. Mitochondrial DNA B Resour. 6(3):710–712. doi:10.1080/23802359.2020.1851153.
- Liu S, Mao S, Ge T, Cai Y, Ji N, Kong X, Shen X. 2021. The first mitochondrial genome of Balanus trigonus Darwin, 1854 (Sessilia: Balanidae) and molecular phylogeny within Cirripedia. Mitochondrial DNA B Resour. 6(6):1740–1742. doi:10.1080/23802359.2021.1930216.
- Mao N, Shao W, Mao S, Cai Y, Ji N, Shen X. 2024. Complete mitochondrial genome of Striatobalanus tenuis Hoek, 1883 (Balanomorpha: balanidae) and a novel molecular phylogeny within Cirripedia. Mitochondrial DNA B Resour. 9(1):29–32. doi:10.1080/23802359.2023.2299087.
- Mao S, Ge T, Cai Y, Ji N, Kong X, Shen X. 2021. The mitochondrial genome of Chthamalus malayensis (Sessilia: chthamalidae) and molecular phylogeny within Cirripedia. Mitochondrial DNA Part B-Resour. 6(2):643–644. doi:10.1080/23802359.2021.1878956.
- Meng G, Li Y, Yang C, Liu S. 2019. MitoZ: a toolkit for animal mitochondrial genome assembly, annotation and visualization. Nucleic Acids Res. 47(11):e63–e63. doi:10.1093/nar/gkz173.
- Miller M, Pfeiffer W, Schwartz T. 2010. Creating the CIPRES Science Gateway for inference of large phylogenetic trees. 2010 Gateway computing environments workshop. New Orleans, LA, USA. doi:10.1109/GCE.2010.5676129.
- National Institute of Biological Resources. 2022. National list of species of Korea; [accessed 2023 Apr 8]. http://kbr.go.kr/.
- Nunez JCB, Rong S, Damian-Serrano A, Burley JT, Elyanow RG, Ferranti DA, Neil KB, Glenner H, Rosenblad MA, Blomberg A, et al. 2021. Ecological Load and Balancing Selection in Circumboreal Barnacles. Mol Biol Evol. 38(2):676–685. doi:10.1093/molbev/msaa227.
- Pérez-Losada M, Høeg JT, Simon-Blecher N, Achituv Y, Jones D, Crandall KA. 2014. Molecular phylogeny, systematics and morphological evolution of the acorn barnacles (Thoracica: sessilia: balanomorpha). Mol Phylogenet Evol. 81:147–158. doi:10.1016/j.ympev.2014.09.013.
- Posada D. 2008. jModelTest: phylogenetic model averaging. Mol Biol Evol. 25(7):1253–1256. doi:10.1093/molbev/msn083.
- Shen Xin, Chan BKK, Tsang LM. 2016. The mitochondrial genome of Nobia grandis Sowerby, 1839 (Cirripedia: Sessilia): the first report from the coral-inhabiting barnacles family Pyrgomatidae. Mitochondrial DNA Part A. 27(1):339–341. doi:10.3109/19401736.2014.892106.
- Shen Xin, Tsoi K-H, Cheang C-C. 2016. The model barnacle Balanus balanus Linnaeus, 1758 (Crustacea: Maxillopoda: Sessilia) mitochondrial genome and gene rearrangements within the family Balanidae. Mitochondrial DNA Part A. 27(3):2112–2114. doi:10.3109/19401736.2014.982581.
- Shen Xin, Chu KH, Chan BKK, Tsang LM. 2016. The complete mitochondrial genome of the fire coral-inhabiting barnacle Megabalanus ajax (Sessilia: Balanidae): gene rearrangements and atypical gene content. Mitochondrial DNA Part A. 27(2):1173–1174. doi:10.3109/19401736.2014.936424.
- Shen Xin, Chan BKK, Tsang LM. 2015. The complete mitochondrial genome of common fouling barnacle Amphibalanus amphitrite (Darwin, 1854) (Sessilia: Balanidae) reveals gene rearrangements compared to pancrustacean ground pattern. Mitochondrial DNA. 26(5):773–774. doi:10.3109/19401736.2013.855745.
- Song Jun, Chen P, Tian Mei, Ji N, Cai Y, Shen Xin. 2020. The first mitochondrial genome of Fistulobalanus albicostatus (Crustacea: Maxillopoda: Sessilia) and phylogenetic consideration within the superfamily Balanoidea. Mitochondrial DNA B Resour. 5(3):2776–2778. doi:10.1080/23802359.2020.1788459.
- Tsang LM, Shen Xin, Chu KH, Chan BKK. 2015. Complete mitochondrial genome of the acorn barnacle Striatobalanus amaryllis (Crustacea: Maxillopoda): the first representative from Archaeobalanidae. Mitochondrial DNA. 26(5):761–762. doi:10.3109/19401736.2013.855739.
- Wernersson R, Pedersen AG. 2003. RevTrans: multiple alignment of coding DNA from aligned amino acid sequences. Nucleic Acids Res. 31(13):3537–3539. doi:10.1093/nar/gkg609.
- Xia X, Lemey P. 2009. Assessing substitution saturation with DAMBE. In The phylogenetic handbook. Cambridge (UK): Cambridge University Press; p. 615–630.
- Zhang M, Cai Y, Ji N, Chan BKK, Shen X. 2023. The mitochondrial genome of the globally invasive barnacle Megabalanus coccopoma Darwin 1854 (Crustacea: balanomorpha): rearrangement and phylogenetic consideration within Balanomorpha. Diversity. 15(1):117. doi:10.3390/d15010117.