Abstract
Amorphophallus paeoniifolius (Dennst.) Nicolson, 1885, often known as elephant foot yam, is a tropical tuber crop that originates from south-east Asia and belongs to the Araceae family. It is known for its high production potential and popularity as a medicinal plant. However, the phylogeny and genes for this species are still unavailable. In this study, the first complete chloroplast genome of A. paeoniifolius was reported and phylogenetic analysis was conducted with Araceae species. The chloroplast genome was 176,258 bp in length with 34.80% overall GC content and includes a large single-copy (LSC) region (93,951 bp), a small single-copy (SSC) region (15,013 bp), and a pair of inverted repeat (IRs) regions (33,647 bp). The chloroplast genome has 130 genes, which include 85 protein-coding genes, 37 tRNA genes, and eight rRNA genes. A maximum-likelihood (ML) phylogenetic analysis indicated that all Amorphophallus species formed a single monophyletic clade with a high bootstrap value and A. paeoniifolius was closely related to A. konjac, A. albus, A. krausei, and A. titanum. The chloroplast genome reported in this study will be useful for further taxonomic and evolutionary studies of Amorphophallus.
Introduction
Amorphophallus Bl. is a genus of perennial herb belonging to the Araceae family, which consists of ∼200 species and occurs in subtropical regions of Asia, West Africa, and islands in the Pacific Ocean (Li et al. Citation2010). Some Amorphophallus species can be important as edible and medicinal species, among which Amorphophallus paeoniifolius (Dennst.) Nicolson, 1885 is a tuberous, robust indigenous herbaceous medicinal plant usually cultivated as a vegetable and readily available tuber plant (Hama Gharib and Salman Citation2023). A. paeoniifolius or elephant foot yam, also commonly called Suran or Jimikand, is a commercially important vegetable crop due to its high production potential that is cultivated commercially in some tropical and subtropical areas, including India, China, Malaysia, Sri Lanka, Indonesia, Thailand, the Philippines, and in tropical regions of Africa (Hama Gharib and Salman Citation2023). A. paeoniifolius has high medicinal value because of its tuber presence of different bioactive molecules, including lupeol, quercetin, gallic acid, β-sitosterol, and betulinic acid (Nataraj et al. Citation2012; Dey et al. Citation2017) and is used ethnomedicinally for the treatment of gastrointestinal disturbances such as piles (hemorrhoids), abdominal pain, and constipation (Dey et al. Citation2017). Meanwhile, it is also used for treatment of other ailments like anemia, splenopathy, elephantiasis, tumors, hemorrhages, cough, bronchitis, asthma, etc. in traditional practices (Dey et al. Citation2017). On the other hand, it has been demonstrated to exhibit antibacterial, antifungal, cytotoxic, as well as analgesic activities in pharmacological experiments (Hama Gharib and Salman Citation2023; Nayak et al. Citation2023).
Current research about Amorphophallus mainly focuses on pharmacological effects (Hama Gharib and Salman Citation2023; Nayak et al. Citation2023), konjac glucomannan characteristics (Devaraj et al. Citation2019), karyotype analysis (Zhao et al. Citation2021), and genetic diversity analysis (Gao et al. Citation2018; Rao et al. Citation2020; Tang et al. Citation2020). The genome size of Amorphophallus is quite large and there is a large variation in the genomic sequences of Amorphophallus species, which makes the whole genome sequencing of Amorphophallus quite challenging (Liu et al. Citation2019). Up to now, only two species of nuclear genomes have been reported (Frisse et al. Citation2022; Gao et al. Citation2022; Li et al. Citation2023). In contrast, the chloroplast genomes are smaller and more conserved, and much easier to achieve in Amorphophallus species (Liu et al. Citation2019). The chloroplast genome sequence is a vital genetic resource for unveiling the origin and phylogenetic relationships of plant species, particularly in species identification and classification (Wang et al. Citation2023). In recent years, the chloroplast genomes of some Amorphophallus species have been published, such as A. konjac (Li et al. Citation2024), A. yunnanensis (Yin and Gao Citation2023a), and A. krausei (Yin and Gao Citation2023b). Although A. paeoniifolius has high medicinal and edible value, there are still few reports on its evolution and genetic background, and its chloroplast genome is unknown. Thus, in this study, the complete chloroplast genome of A. paeoniifolius was sequenced and described, and the phylogeny of family Araceae was constructed to explore the interspecific relationships.
Materials and methods
The fresh leaves of Amorphophallus paeoniifolius were obtained from Konjac Genetic Resources Garden of Kunming University, Yunnan Province (, 24.97406°N, 102.79605°E). The sample was deposited in the Herbarium of Yunnan Urban Agricultural Engineering and Technological Research Center, Kunming University (voucher number: YBMY20230620, Li-Fang Li, [email protected]). Whole genomic DNA was extracted using the DNeasy Plant Mini kit (Qiagen, Hilden, Germany) following the manufacturer’s instruction. Then, the high-quality DNA was used to construct a 350 bp insert-size paired-end sequencing library using the Nextera XT DNA library preparation kit (Illumina, San Diego, CA). The constructed library was sequenced using the Illumina NovaSeq 6000 platform, and the coverage was measured using samtools depth. The quality control of the sequencing raw reads was performed using NGS QC Tool Kit v2.3.3 (Patel and Jain Citation2012). Then, the high-quality reads were assembled into the chloroplast genome using SPAdes 3.14.1 (Bankevich et al. Citation2012). Annotation of the chloroplast genome of A. paeoniifolius was performed using PGA (Qu et al. Citation2019) with A. coaetaneus (OQ404947) as a reference genome. Finally, a circular map of the complete chloroplast genome and cis/trans-splicing genes maps in A. paeoniifolius were drawn by CPGView (Liu et al. Citation2023). To reveal the phylogenetic relationship of A. paeoniifolius in Araceae, the chloroplast genomes of 17 other species in the Araceae family, including eight Amorphophallus species and one Styracaceae species were downloaded from NCBI. A total of 26 Araceae species were used for phylogenetic analysis and Styrax obassia was used as an outgroup. The whole chloroplast genomes were then aligned using MAFFT with default parameter (Katoh and Standley Citation2013). The best suitable model was estimated by ModelFinder and the maximum-likelihood (ML) tree was constructed using IQTREE v1.6.12 (Nguyen et al. Citation2015; Kalyaanamoorthy et al. Citation2017). The bootstrap support value was calculated from 1000 iterations. Tree visualization was performed using FigTree v. 1.4.3.
Figure 1. Morphological characteristics of Amorphophallus paeoniifolius: (A) leaf, (B) petiole detail, (C) flower, and (D) spadix exposed. These photos were taken by the author Lei Yu and Lifang Li at the greenhouse of Kunming University. A. paeoniifolius leaf solitary or paired, the lamina is highly dissected and the leaflets are oval, obovate, elliptic, and elongate elliptic. The petiole is dark green and covered with pale patches. The surface of the entire petiole is rough and has verrucous convex. The inflorescence is short pedunculate and sits more or less on the soil surface. The spadix is sessile, shorter or longer than spathe.
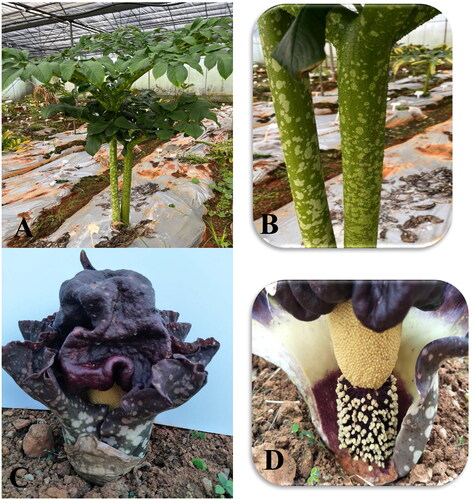
Results
The total length of the chloroplast genome of A. paeoniifolius was 176,258 bp, with an average coverage of 1252× (Supplemental Figure 1) and an overall GC content of 34.8%. The complete chloroplast genome sequence of A. paeoniifolius was submitted to the NCBI GenBank database under the accession number of OR995732. The assembled genome exhibited a typical quadripartite structure, consisting of a large single-copy region (LSC: 93,951 bp), a small single-copy (SSC: 15,013 bp), and two inverted repeating regions (IRs: 33,647 bp) (). In the A. paeoniifolius chloroplast genome, 130 functional genes were predicted, including 85 protein-coding genes, eight ribosomal RNA (rRNA) genes, and 37 transfer RNA (tRNA) genes, of which 78 protein-coding genes, 29 tRNA genes, and four rRNA genes are unique, respectively. Of these genes, seven protein-coding genes (ndhB, rpl23, rpl2, rps7, rps12, ycf1, and ycf2), seven tRNAs (trnM-CAU, trnV-GAC, trnI-GAU, trnA-UGC, trnR-ACG, trnN-GUU, and trnL-CAA), and four rRNAs (rrn16, rrn23, rrn4.5, and rrn5) are duplicated in the IR regions. Furthermore, in the chloroplast genome of A. paeoniifolius, nine protein-coding genes (rps16, atpF, rpoC1, petB, petD, rpl16, rpl2, ndhB, and ndhA) and six tRNAs (trnK-UUU, trnG-UCC, trnL-UAA, trnV-UAC, trnI-GAU, and trnA-UGC) contained a single intron, while clpP and ycf3 each contained two introns. In addition, 13 cis-splicing genes and one trans-splicing gene (rps12) were discovered (Supplemental Figures 2 and 3).
Figure 2. The circular map of the chloroplast genome of Amorphophallus paeoniifolius, generated by CPGview (http://www.1kmpg.cn/cpgview/). From the center outward, the first track shows the dispersed repeats including direct repeats (red) and palindromic repeats (green). The second track shows the long tandem repeats as short blue bars. The third track shows the short tandem repeats or microsatellite sequences as short bars with different colors. The colors, the type of repeat they represent, and the description of the repeat types is as follows. Black: complex repeat; green: p1 (repeat unit size = 1); yellow: p2 (repeat unit size = 2); purple: p3 (repeat unit size = 3); blue: p4 (repeat unit size = 4); orange: p5 (repeat unit size = 5); red: p6 (repeat unit size = 6). The quadripartite structure including LSC, SSC, and a pair of IR regions is shown on the fourth track. The GC content of the genome is represented in the fifth track by dark gray. The base frequency at each site along the genome will be shown between the fourth and fifth tracks. Genes belonging to different functional groups are encoded by color on the sixth track. The legends in different colors at the left bottom represent genes with different functions. The transcription directions for the inner and outer genes are clockwise and anticlockwise, respectively.
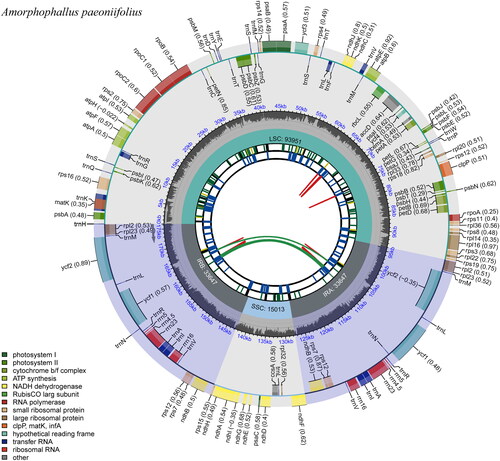
The phylogenetic analysis showed that eight Amorphophallus species formed a single monophyletic clade with the highest probability (). This tree suggested a close relationship among A. konjac, A. albus, A. krausei, A. titanum, and A. paeoniifolius. The phylogenetic tree also indicated that the genera Amorphophallus was shown to be closely related to Caladium bicolor, Caladium humboldtii, and Syngonium angustatum.
Figure 3. Maximum-likelihood (ML) phylogenetic tree of Amorphophallus paeoniifolius (in red) and 26 other complete chloroplast genome sequences. The best-fit model according to the Bayesian information criterion (BIC) was GTR + F + R4. Numbers at each node represent the bootstrap values for 1000 replicates. A. paeoniifolius is marked in red. The following sequences were used: Amorphophallus paeoniifolius OR995732 (this study) Amorphophallus konjac OR438675 (Li et al. Citation2024), Amorphophallus konjac MK611803.1 (Hu et al. Citation2019), Amorphophallus albus OP531918 (Shan et al. Citation2023), Amorphophallus krausei OR416863 (Yin and Gao Citation2023b), Amorphophallus titanum MN046883 (Abdullah et al. Citation2021), Amorphophallus coaetaneus OQ404947 (Gao et al. Citation2023), Amorphophallus yunnanensis OR400247 (Yin and Gao Citation2023a), Caladium bicolor ON707031 (Ye et al. Citation2022), Caladium humboldtii ON707032 (Ye et al. Citation2022), Syngonium angustatum MN046894 (Henriquez et al. Citation2020a), Arisaema franchetianum MN046885 (Henriquez et al. Citation2020a), Arisaema bockii MZ380241 (Yi et al. Citation2021), Pinellia pedatisecta MN046890 (Henriquez et al. Citation2020a), Pinellia ternata MT193722 (Cai et al. Citation2020), Typhonium blumei MT872311 (Low et al. Citation2021), Philodendron hederaceum OM937109 (Nah et al. Citation2024), Sauromatum giganteum MN626718 (Kim et al. Citation2020), Alocasia navicularis MN046882 (Henriquez et al. Citation2020a), Pistia stratiotes MN885890 (Quan and Chen Citation2020), Calla palustris MN046887 (Henriquez et al. Citation2020a), Montrichardia arborescens MN046889 (Henriquez et al. Citation2020a), Anubias heterophylla MN046884 (Henriquez et al. Citation2020a), Monstera adansonii, MN046888 (Henriquez et al. Citation2020a), Stenospermation multiovulatum MN046893 (Henriquez et al. Citation2020b), Anthurium andraeanum OP938256 (Wan et al. Citation2023), and Styrax obassia MZ285733 (Song et al. Citation2020).
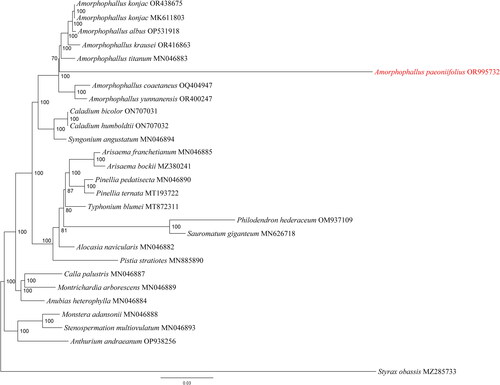
Discussion and conclusions
In this study, the chloroplast genome of A. paeoniifolius was sequenced by the NGS methods. The genome exhibited typical quadripartite structures, including a LSC, a SSC, and a pair of IRs, like most other angiosperms. The GC content of the whole genome was 34.8%, which was similar to those of other species in Amorphophallus (Abdullah et al. Citation2021; Yin and Gao Citation2023a, Citation2023b; Li et al. Citation2024). There are 130 genes in A. paeoniifolius chloroplast genome, meanwhile, 128 up to 133 such genes are reported present in other Amorphophallus plants, including 133 for A. coaetaneus (Gao et al. Citation2023), 128 for A. yunnanensis (Yin and Gao Citation2023a), 132 for A. krausei (Yin and Gao Citation2023b), 130 for A. titanium (Abdullah et al. Citation2021), and 131 for A. konjac (Li et al. Citation2024). The ML tree showed that sampled species of the genus Amorphophallus formed a monophyletic group with high support value, which was consistent with previous studies (Yin and Gao Citation2023a, Citation2023b; Li et al. Citation2024). In conclusion, our study not only enriches the genomic information of A. paeoniifolius, but also lays the foundation for understanding the genetic diversity, evolution, and phylogeny within the genus Amorphophallus.
Author contributions
Lifang Li conceived the project and wrote the manuscript. Min Yang, Ying Qi, Yajun Yu, and Penghua Gao conceived the project and collected the sample. Shaowu Yang, Yongteng Zhao, Jianwei Guo, and Jiani Liu performed the data analysis. Feiyan Huang conceived, designed the study, and edited the paper. Lei Yu conceived the study, interpreted the data, and revised the manuscript critically. All authors read and approved the final manuscript.
Ethical approval
The material involved in the article does not represent an endangered or protected species; therefore, permission is not required to collect this species. Research on this species, including the collection of plant materials has been carried out in accordance with guidelines provided by Kunming University.
Supplementary materials.docx
Download MS Word (1.8 MB)Disclosure statement
No potential conflict of interest was reported by the author(s).
Data availability statement
The assembled chloroplast genome sequence data that support the findings of this study are openly available in GenBank of NCBI (https://www.ncbi.nlm.nih.gov/) under the accession number of OR995732. The associated BioProject, SRA, and Bio-Sample numbers are PRJNA1090420, SRR28407607, and SAMN40564457, respectively.
Additional information
Funding
References
- Abdullah Henriquez CL, Mehmood F, Hayat A, Sammad A, Waseem S, Waheed MT, Matthews PJ, Croat TB, Poczai P, et al. 2021. Chloroplast genome evolution in the Dracunculus clade (Aroideae, Araceae). Genomics. 113(1 Pt 1):183–192. doi:10.1016/j.ygeno.2020.12.016.
- Bankevich A, Nurk S, Antipov D, Gurevich AA, Dvorkin M, Kulikov AS, Lesin VM, Nikolenko SI, Pham S, Prjibelski AD, et al. 2012. SPAdes: a new genome assembly algorithm and its applications to single-cell sequencing. J Comput Biol. 19(5):455–477. doi:10.1089/cmb.2012.0021.
- Cai Z, Wang H, Wang G. 2020. Complete chloroplast genome sequence of Pinellia ternata (Thunb.) Breit, a medicinal plants to China. Mitochondrial DNA B Resour. 5(3):2107–2108. doi:10.1080/23802359.2020.1765207.
- Devaraj RD, Reddy CK, Xu B. 2019. Health-promoting effects of konjac glucomannan and its practical applications: a critical review. Int J Biol Macromol. 126:273–281. doi:10.1016/j.ijbiomac.2018.12.203.
- Dey YN, Sharma G, Wanjari MM, Kumar D, Lomash V, Jadhav AD. 2017. Beneficial effect of Amorphophallus paeoniifolius tuber on experimental ulcerative colitis in rats. Pharm Biol. 55(1):53–62. doi:10.1080/13880209.2016.1226904.
- Frisse L, Martinez MA, Pirro S. 2022. The complete genome sequence of Amorphophallus titanum, the corpse flower. Biodivers Genomes. doi:10.56179/001c.37841.
- Gao Y, Dong K, Xiao P, Wu W, Yin S. 2023. Complete assembly of the chloroplast genome of Amorphophallus coaetaneus S. Y. Liu & S. J. Wei 1986 (Araceae) from southwestern China. Mitochondrial DNA B Resour. 8(7):766–770. doi:10.1080/23802359.2023.2238939.
- Gao Y, Yin S, Yang H, Wu L, Yan Y. 2018. Genetic diversity and phylogenetic relationships of seven Amorphophallus species in southwestern China revealed by chloroplast DNA sequences. Mitochondrial DNA A DNA Mapp Seq Anal. 29(5):679–686. doi:10.1080/24701394.2017.1350855.
- Gao Y, Zhang Y, Feng C, Chu H, Feng C, Wang H, Wu L, Yin S, Liu C, Chen H, et al. 2022. A chromosome-level genome assembly of Amorphophallus konjac provides insights into konjac glucomannan biosynthesis. Comput Struct Biotechnol J. 20:1002–1011. doi:10.1016/j.csbj.2022.02.009.
- Hama Gharib DS, Salman RF. 2023. Feasibility of the crude extracts of Amorphophallus paeoniifolius and Colocasia esculenta as intracanal medicaments in endodontic therapy in comparison to the 940 nm diode laser: an in vitro antimicrobial study. J Dent Sci. 18(1):145–156. doi:10.1016/j.jds.2022.06.023.
- Henriquez CL, Abdullah, Ahmed I, Carlsen MM, Zuluaga A, Croat TB, McKain MR. 2020a. Evolutionary dynamics of chloroplast genomes in subfamily Aroideae (Araceae). Genomics. 112(3):2349–2360. doi:10.1016/j.ygeno.2020.01.006.
- Henriquez CL, Abdullah, Ahmed I, Carlsen MM, Zuluaga A, Croat TB, McKain MR. 2020b. Molecular evolution of chloroplast genomes in Monsteroideae (Araceae). Planta. 251(3):72. doi:10.1007/s00425-020-03365-7.
- Hu H, Liu J, Wang B, An J, Wang Q. 2019. Characterization of the complete chloroplast genome of Amorphophallus konjac (Araceae) and its phylogenetic analysis. Mitochondrial DNA Part B. 4(1):1658–1659. doi:10.1080/23802359.2019.1606683.
- Kalyaanamoorthy S, Minh BQ, Wong T, Haeseler AV, Jermiin LS. 2017. ModelFinder: fast model selection for accurate phylogenetic estimates. Nat Methods. 14(6):587–589. doi:10.1038/nmeth.4285.
- Katoh K, Standley DM. 2013. MAFFT multiple sequence alignment software version 7: improvements in performance and usability. Mol Biol Evol. 30(4):772–780. doi:10.1093/molbev/mst010.
- Kim J, Lee J, Choi S, Um S, Kim H, Chun HS, Nah G. 2020. The complete chloroplast genome of Typhonium giganteum (Araceae). Mitochondrial DNA B Resour. 5(3):2994–2995. doi:10.1080/23802359.2020.1797571.
- Li H, Zhu G, Boyce PC, Jin M, Hetterscheid WLA, Bogner J, Jacobsen N. 2010. Araceae. Flora of China. Beijing: Science Press.
- Li L, Qi Y, Gao P, Yang S, Zhao Y, Guo J, Liu J, Huang F, Yu L. 2024. The complete chloroplast genome sequence of Amorphophallus konjac (Araceae) from Yunnan, China and its phylogenetic analysis in the family Araceae. Mitochondrial DNA B Resour. 9(1):41–45. doi:10.1080/23802359.2023.2300471.
- Li L, Yang M, Wei W, Zhao J, Yu X, Impaprasert R, Wang J, Liu J, Huang F, Srzednicki G, et al. 2023. Characteristics of Amorphophallus konjac as indicated by its genome. Sci Rep. 13(1):22684. doi:10.1038/s41598-023-49963-9.
- Liu E, Yang C, Liu J, Jin S, Harijati N, Hu Z, Diao Y, Zhao L. 2019. Comparative analysis of complete chloroplast genome sequences of four major Amorphophallus species. Sci Rep. 9(1):809. doi:10.1038/s41598-018-37456-z.
- Liu S, Ni Y, Li J, Zhang X, Yang H, Chen H, Liu C. 2023. CPGView: a package for visualizing detailed chloroplast genome structures. Mol Ecol Resour. 23:1–11.
- Low SL, Yu C-C, Ooi IH, Eiadthong W, Galloway A, Zhou Z-K, Xing Y-W. 2021. Extensive Miocene speciation in and out of Indochina: the biogeographic history of Typhonium sensu stricto (Araceae) and its implication for the assembly of Indochina flora. J Syst Evol. 59(3):419–428. doi:10.1111/jse.12689.
- Nah G, Jeong JR, Lee JH, Soh SY, Nam SY. 2024. The complete chloroplast genome of Philodendron hederaceum (Jacq.) Schott 1829 (Alismatales: Araceae). Mitochondrial DNA B Resour. 9(2):262–266. doi:10.1080/23802359.2024.2311748.
- Nataraj HN, Murthy RLN, Setty R. 2012. Evaluation of gastroprotective ability of Amorphophallus paeoniifolius corms against indomethacin induced gastric ulcers. RGUHS J Pharm Sci. 2(1):67–73. doi:10.5530/rjps.2012.1.9.
- Nayak A, Sowmya BR, Gandla H, Kottrashetti V, Ingalagi P, Srinivas VSC. 2023. Determination and comparison of antimicrobial activity of aqueous and ethanolic extracts of Amorphophallus paeoniifolius on periodontal pathogens: an in vitro study. J Indian Soc Periodontol. 27(1):40–44. doi:10.4103/jisp.jisp_182_22.
- Nguyen L-T, Schmidt HA, von Haeseler A, Minh BQ. 2015. IQ-TREE: a fast and effective stochastic algorithm for estimating maximum-likelihood phylogenies. Mol Biol Evol. 32(1):268–274. doi:10.1093/molbev/msu300.
- Patel RK, Jain M. 2012. NGS QC Toolkit: a toolkit for quality control of next generation sequencing data. PLOS One. 7(2):e30619. doi:10.1371/journal.pone.0030619.
- Qu XJ, Moore MJ, Li DZ, Yi TS. 2019. PGA: a software package for rapid, accurate, and flexible batch annotation of plastomes. Plant Methods. 15(1):50. doi:10.1186/s13007-019-0435-7.
- Quan G, Chen L. 2020. Characterization of the complete chloroplast genome sequence of Pistia stratiotes (Araceae) and its phylogenetic implications. Mitochondrial DNA B Resour. 5(3):2168–2169. doi:10.1080/23802359.2020.1768935.
- Rao GP, Reddy MG, Priya M, Bahadur A, Dubey DK. 2020. Characterization and genetic diversity of phytoplasmas associated with elephant foot yam (Amorphophallus paeoniifolius) in India. 3 Biotech. 10(3):83. doi:10.1007/s13205-020-2069-x.
- Shan Y, Li J, Zhang X, Yu J. 2023. The complete mitochondrial genome of Amorphophallus albus and development of molecular markers for five Amorphophallus species based on mitochondrial DNA. Front Plant Sci. 14:1180417. doi:10.3389/fpls.2023.1180417.
- Song Y, Zhao W, Xu J, Li M, Zhang Y. 2020. Chloroplast genome evolution and species identification of Styrax (Styracaceae). Biomed Res Int. 2022:5364094.
- Tang R, Liu E, Zhang Y, Schinnerl J, Sun W, Chen G. 2020. Genetic diversity and population structure of Amorphophallus albus, a plant species with extremely small populations (PSESP) endemic to dry-hot valley of Jinsha River. BMC Genet. 21(1):102. doi:10.1186/s12863-020-00910-x.
- Wan X, Ge Y, Pan G, Tian D. 2023. The complete chloroplast genome sequence of Anthurium andraeanum Linden (Araceae; Pothoideae). Mitochondrial DNA B Resour. 8(3):379–382. doi:10.1080/23802359.2023.2185081.
- Wang Y, Huang J, Xie N, Zhang D, Tong W, Xia E. 2023. The complete chloroplast genome sequence of Camellia atrothea (Ericales: Theaceae). Mitochondrial DNA B Resour. 8(4):536–540. doi:10.1080/23802359.2023.2204972.
- Ye Y, Liu J, Zhou Y, Zhu G, Tan J, Xu Y. 2022. Complete chloroplast genome sequences of four species in the Caladium genus: comparative and phylogenetic analyses. Genes. 13(12):2180. doi:10.3390/genes13122180.
- Yi S, Xu T, Song X, Wang W, Wang G, Yu W, Han B. 2021. The complete chloroplast genome of Arisaema bockii Engler and its phylogenetic analysis in the family Araceae. Mitochondrial DNA B Resour. 6(11):3283–3285. doi:10.1080/23802359.2021.1993107.
- Yin S, Gao Y. 2023a. Characterization of the complete chloroplast genome assembly of Amorphophallus yunnanensis Engler, Pflanzenr (Araceae) from southwestern China. Mitochondrial DNA B Resour. 8(12):1445–1449. doi:10.1080/23802359.2023.2294896.
- Yin S, Gao Y. 2023b. The complete chloroplast genome assembly of Amorphophallus krausei Engler, Pflanzenr 1911 (Araceae) from southwestern China. Mitochondrial DNA B Resour. 8(12):1339–1342. doi:10.1080/23802359.2023.2288889.
- Zhao C, She X, Liu E, Harijati N, Cheng T, Diao Y, Jin S, Hu Z. 2021. A mixed ploidy natural population of Amorphophallus muelleri provides an opportunity to trace the evolution of Amorphophallus karyotype. J Genet. 100(1):10. doi:10.1007/s12041-020-01255-1.