For many decades, the folate antagonist methotrexate (MTX) is being used as first-line chemotherapeutic agent for the treatment of (pediatric) leukemia [Citation1] and also constitutes the cornerstone in the treatment of adult rheumatoid arthritis (RA) [Citation2], and juvenile idiopathic arthritis (JIA) [Citation3]. Treatment of RA and JIA is based on low dosages of MTX (7.5–30 mg/wk) as opposed to high-dose initiation MTX treatment (up to 10 g/m2) in leukemia. Maintenance therapy of childhood leukemia often includes a low-dose oral MTX. The mechanism of action of MTX and its cellular pharmacology in RA and cancer are overlapping [Citation1,Citation2,Citation4]. Following cellular uptake of MTX via the reduced folate carrier (RFC), one of the critical factors for the therapeutic effects of MTX involves the intracellular conversion into MTX-polyglutamates (MTX-PGn). This process is catalyzed by the enzyme folylpolyglutamate synthetase (FPGS), which attaches multiple glutamate moieties (n = 2–5 depending on the MTX dose and duration of infusion/treatment) [Citation5]. MTX polyglutamylation results in enhanced intracellular retention because long-chain polyanionic MTX-PGn cannot be exported from cells by ABC drug efflux transporters [Citation6]. Non-polyglutamated MTX is poorly retained and extruded from cells by ABC transporters and rapidly cleared from plasma. Furthermore, long-chain MTX-PGn enhance pharmacological efficacy by more potent inhibition of intracellular target enzymes, including dihydrofolate reductase (DHFR) and thymidylate synthase (TS) being involved in DNA synthesis. This mechanism confers the anti-proliferative effect of MTX (). The mechanism of action of low-dose MTX treatment used in RA relates to the inhibition of key enzymes in purine de novo synthesis pathway (i.e. 5-aminoimidazole-4-carboxamide ribonucleotide transformylase; ATIC) by MTX-PGn and the downstream release of the anti-inflammatory purine adenosine [Citation2, Citation4, Citation7]. Together, these mechanisms underscore the crucial relevance of polyglutamylation for the therapeutic effects of MTX.
Figure 1. Therapeutic drug monitoring during low-dose MTX treatment of RA as a tool for personalized therapy. After RA diagnosis, a prediction model based on known determinants of MTX efficacy is employed (blue box). After initializing treatment with MTX, RBC-MTX-PGn measurements can be performed to monitor MTX efficacy and toxicity. Commonly, a steady state is reached after approximately 3 months (purple box). Furthermore, measurements of MTX-PGn and FPGS activity in PBMCs could be used for further improvement of MTX therapeutic drug monitoring. Finally, FPGS splice variants could be added to the prediction model. Crosses indicate possible laboratory measurements (RBC/PBMC MTX-PGn and FPGS activity) to assess MTX underdosing, toxicity, and efficacy. Abbreviations: PBMC, peripheral blood mononuclear cells; MTX-PGn, methotrexate polylglutamates; RBC, red blood cell; FPGS, folylpolylglutamate synthetase; DAS, disease activity score; HAQ, Health Assessment Questionnaire; ABC, adenosine triphosphate binding cassette transporter; ABCB1, ABC subtype B1; ABCC3, ABC subtype C3; BMI, body mass index.
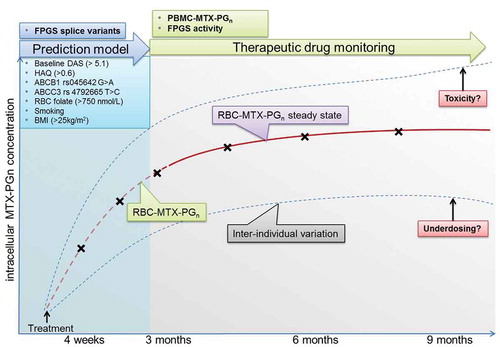
Although MTX is relatively safe and effective, still about 30%–40% of RA patients discontinue MTX treatment due to intolerance or inefficacy early in the treatment (primary failure) or do not respond any more later, after initial response (secondary failure) to the drug after 3–6 months of therapy (MTX non-response or MTX resistance) [Citation2, Citation4]. Non-responders are then often switched to expensive biological (antibody-based) disease modifying anti-rheumatic drugs (bDMARDs) [Citation8]. The reasons/mechanisms for non-response have not been fully clarified but may include inter-individual differences in MTX uptake and/or metabolism, non-compliance or underdosing. In fact, a recent study indicated that in the United States, 63% of RA patients were under-dosed at MTX dosages <15 mg/wk [Citation9] and thus treatment can be considered as ineffective.
As early effective treatment is mandatory to prevent irreversible joint destruction and long-term disabilities, therapeutic drug monitoring (TDM) of MTX may be helpful in guiding rheumatologists to personalize treatment by identifying RA patients who will or will not respond to MTX.
Genetic studies explored whether individual single nucleotide polymorphisms (SNPs) of genes or combinations of SNPs encoding MTX transport proteins and key enzymes in folate metabolism are correlated with MTX (non)-response [Citation10]. Although selected SNPs were identified (i.e. adenosine monophosphate deaminase 1 (AMPD1) 34C > T, ATIC 347C > G, inosine triphosphatase (ITPA) 94A > C, methylenetetrahydrofolate dehydrogenase 1 (MTHFD1) 1958G > A) and used to build a pharmacogenetic prediction model for MTX response [Citation11], genetic associations were not always consistent [Citation12] or could not be replicated in various international cohorts [Citation13].
Biochemical studies to discover predictive markers for MTX response in RA have been hampered by the lack of sensitivity and accuracy of analytical methods in cell types relevant in the pathogenesis of RA. With recent technological advancements, revisitation of biochemical markers of MTX response constitutes an open field of exploration. Early biochemical/pharmacokinetic studies were initiated to measure MTX levels in plasma or blood cells for correlations with MTX response. However, as MTX in its monoglutamate form is poorly retained and extruded from cells and rapidly cleared from plasma, TDM of plasma MTX is hardly possible in low-dose MTX treatment in RA [Citation14]. Hence, for the assessment of clinically-active levels of MTX, it is more relevant to measure MTX-PGn levels in blood cells rather than MTX concentrations in plasma. Following these considerations, MTX-PGn levels have been analyzed in red blood cells (RBC) of RA patients and used as potential marker for therapy response, tacitly assuming that MTX-PGn levels in RBCs may serve as a surrogate marker for MTX-PGn levels in immune-competent cells [Citation15, Citation16]. In these cross-sectional observational studies, a large inter-patient variability up to 10-fold in RBC MTX-PGn accumulation was observed which reached a steady state after 3 months treatment. MTX-PGn levels in RBCs had no relationship with reduction of disease activity [Citation16].
Recently, three prospective studies were completed [Citation17–Citation19] revealing that RA patients administered the same oral dose of MTX had large inter-individual variation of MTX-PGn accumulation and kinetics of accumulation that reached steady state after 3 months of therapy. Furthermore, a dose–response effect was observed between increasing the MTX dose and the accumulation of total and longer chain MTX-PGn in RBCs of RA patients, also reaching a steady state after 3 months [Citation17, Citation18] (). Finally, these studies demonstrated that an increase in RBC MTX-PGn concentration, in particular MTX-PG3 levels and total MTX-PGn level, was associated with a better therapeutic response, that is, a decreased disease activity score (DAS28) over 9 months. Collectively, these studies also identified other determinants impacting therapy response in RA patients: increased age, gender (female), smoking, lower BMI, higher baseline RBC folate status, gene polymorphic variants of ABC drug efflux transporters ABCB1 and ABCC3, and a FPGS gene polymorphic variant (SNP rs4451422) [Citation20] (, blue box inset). Along with clinical variables such as baseline DAS28 score, these parameters have been included in a MTX-prediction model that demonstrated an area under the curve (AUC) of 0.8 (R. de Jonge, unpublished results).
The most critical questions remaining are which analytical options should be considered to further improve MTX personalized therapy by using MTX-TDM and MTX-response prediction models? First, it has to be recognized that RBCs are not the primary target cells for MTX in RA and it is unknown whether MTX-PGn levels in RBCs actually mimic MTX-PGn levels accumulating in immune-effector cells (e.g. T cells, monocytes/macrophages) that represent the ultimate target cells in RA. Moreover, RBCs do not have nuclei and intracellular organelles to control folate and MTX homeostasis in contrast to immune-effector cells. Thus, conceivably analysis of MTX-PGn levels in peripheral blood mononuclear cells (PBMCs) of RA patients during MTX treatment might be of greater clinical relevance, but on the other hand more analytically challenging. Building on the expertise with MTX-PGn analysis and quantification in RBCs by stable isotope dilution liquid chromatography/mass spectrometry (LC-MS/MS) with 13C515N-labeled MTX-PG1-7 internal standards [Citation17, Citation21], we were able to modify this method for the analysis of MTX-PGn in low amounts (<5 x 106) of PBMCs of RA patients [Citation22]. The availability of a sensitive and quantitative LC-MS/MS method to determine MTX-PGn levels in PBMCs allows implementation in future MTX-TDM studies and may help to define whether MTX-PGn analysis in PBMCs is of added value for predicting MTX response.
Second, the impact of the FPGS activity for MTX-polyglutamylation in RA is still poorly understood. In leukemia, loss of FPGS catalytic activity along with diminished MTX-PGn accumulation in leukemic blast cells has been recognized as a common cause of non-response and poor overall survival of leukemia patients treated with high-dose MTX [Citation5,Citation6,Citation23]. Recently, it was demonstrated that aberrant pre-mRNA splicing of FPGS could constitute a molecular basis for the loss of FPGS activity and consequently decreased MTX-PGn accumulation in leukemic cells [Citation24]. In an RA setting, FPGS activity analysis in RBCs is meaningless as mature RBCs have marginal FPGS activity. FPGS activity measurements in PBMCs of RA patients would be more meaningful but have not been performed, on one hand because of the lack of a sensitive (non-radioactive) assay, on the other hand the presumed low FPGS activity associated with non/low-proliferating PBMCs, would require large amounts of cells for accurate measurements. Indeed FPGS activity in bone-marrow cells is very low and even using a sensitive radio-active assay was not measurable in human PBMCs [Citation25]. To meet these shortcomings, we adapted the above-mentioned LC-MS/MS method for MTX-PGn analyses in PBMCs to quantify the FPGS activity reaction product MTX-Glu2 in extracts of 5 × 106 RA patient’s PBMCs [Citation22]. The feasibility of FPGS analysis in PBMCs of RA patients combined with molecular analysis for FPGS pre-mRNA splicing aberrations could then provide insight into the contributing role of FPGS in variations of MTX-PGn accumulation and MTX response in RA.
Altogether, the availability of analytical tools to measure MTX-PGn levels in RBCs and PBMCs, and measure FPGS catalytic activity in PBMCs of RA allows longitudinal MTX therapeutic drug monitoring in RA patients. Given the large inter-individual variability in MTX-PGn accumulation at standard dosages of MTX, TDM may guide optimization of MTX dosages. Identification of cut-off MTX-PGn levels for optimal response may lead to earlier titration (in cases of under dosing) to optimal intracellular MTX-PGn levels and more efficient and safe MTX use. On top of this, TDM may also identify patient overdosing allowing recommendations for dose reductions to minimize unnecessary toxicities. Expanded knowledge of the novel determinants of intracellular MTX-PGn accumulation may improve MTX drug dosing, route of administration, and individualized MTX treatment to reach the optimal target dose for maximal efficacy and minimal toxicity. The novel determinants may serve as standalone prediction markers or can complement other prediction markers to guide the rheumatologist in efficacy assessments of MTX-containing treatment regimens to individual RA patients.
Declaration of interest
The authors have no relevant affiliations or financial involvement with any organization or entity with a financial interest in or financial conflict with the subject matter or materials discussed in the manuscript. This includes employment, consultancies, honoraria, stock ownership or options, expert testimony, grants or patents received or pending, or royalties.
Reviewer disclosures
Peer reviewers on this manuscript have no relevant financial or other relationships to disclose.
Additional information
Funding
References
- Bertino JR. Karnofsky memorial lecture. Ode to methotrexate. J Clin Oncol. 1993 Jan;11(1):5–14. PubMed PMID: 8418242.
- Brown PM, Pratt AG, Isaacs JD. Mechanism of action of methotrexate in rheumatoid arthritis, and the search for biomarkers. Nat Rev Rheumatol. 2016 Dec;12(12):731–742. PubMed PMID: 27784891.
- Bulatovic M, Heijstek MW, Van Dijkhuizen EH, et al. Prediction of clinical non-response to methotrexate treatment in juvenile idiopathic arthritis. Ann Rheum Dis. 2012 Sep;71(9):1484–1489. PubMed PMID: 22473625.
- van der Heijden JW, Dijkmans BA, Scheper RJ, et al. Drug Insight: resistance to methotrexate and other disease-modifying antirheumatic drugs–from bench to bedside. Nat Clin Pract Rheumatol. 2007 Jan;3(1):26–34. PubMed PMID: 17203006.
- Raz S, Stark M, Assaraf YG. Folylpoly-gamma-glutamate synthetase: a key determinant of folate homeostasis and antifolate resistance in cancer. Drug Resist Updat. 2016 Sep;28:43–64. PubMed PMID: 27620954.
- Assaraf YG. Molecular basis of antifolate resistance. Cancer Metastasis Rev. 2007 Mar;26(1):153–181. PubMed PMID: 17333344.
- Cronstein BN, Sitkovsky M. Adenosine and adenosine receptors in the pathogenesis and treatment of rheumatic diseases. Nat Rev Rheumatol. 2017 Jan;13(1):41–51. PubMed PMID: 27829671; PubMed Central PMCID: PMC5173391.
- Smolen JS, Landewe R, Bijlsma J, et al. EULAR recommendations for the management of rheumatoid arthritis with synthetic and biological disease-modifying antirheumatic drugs: 2016 update. Ann Rheum Dis. 2017 Jun;76(6):960–977. PubMed PMID: 28264816.
- Rohr MK, Mikuls TR, Cohen SB, et al. Underuse of methotrexate in the treatment of rheumatoid arthritis: a national analysis of prescribing practices in the US. Arthritis Care Res (Hoboken). 2017 Jun;69(6):794–800. PubMed PMID: 27863180.
- Wessels JA, de Vries-Bouwstra JK, Heijmans BT, et al. Efficacy and toxicity of methotrexate in early rheumatoid arthritis are associated with single-nucleotide polymorphisms in genes coding for folate pathway enzymes. Arthritis Rheum. 2006 Apr;54(4):1087–1095. PubMed PMID: 16572443.
- Wessels JA, van der Kooij SM, le Cessie S, et al. A clinical pharmacogenetic model to predict the efficacy of methotrexate monotherapy in recent-onset rheumatoid arthritis. Arthritis Rheum. 2007 Jun;56(6):1765–1775. PubMed PMID: 17530705.
- Stamp LK, Roberts RL. Effect of genetic polymorphisms in the folate pathway on methotrexate therapy in rheumatic diseases. Pharmacogenomics. 2011 Oct;12(10):1449–1463. PubMed PMID: 22008049.
- Dervieux T, Wessels JA, Kremer JM, et al. Patterns of interaction between genetic and nongenetic attributes and methotrexate efficacy in rheumatoid arthritis. Pharmacogenet Genomics. 2012 Jan;22(1):1–9. PubMed PMID: 22044941.
- Hoekstra M, Haagsma C, Neef C, et al. Bioavailability of higher dose methotrexate comparing oral and subcutaneous administration in patients with rheumatoid arthritis. J Rheumatol. 2004 Apr;31(4):645–648. PubMed PMID: 15088287.
- Dervieux T, Furst D, Lein DO, et al. Pharmacogenetic and metabolite measurements are associated with clinical status in patients with rheumatoid arthritis treated with methotrexate: results of a multicentred cross sectional observational study. Ann Rheum Dis. 2005 Aug;64(8):1180–1185. PubMed PMID: 15677700; PubMed Central PMCID: PMCPMC1755602.
- Stamp LK, O’Donnell JL, Chapman PT, et al. Methotrexate polyglutamate concentrations are not associated with disease control in rheumatoid arthritis patients receiving long-term methotrexate therapy. Arthritis Rheum. 2010 Feb;62(2):359–368. PubMed PMID: 20112376.
- de Rotte MC, den Boer E, de Jong PH, et al. Methotrexate polyglutamates in erythrocytes are associated with lower disease activity in patients with rheumatoid arthritis. Ann Rheum Dis. 2015 Feb;74(2):408–414. PubMed PMID: 24297383.
- Calasan MB, den Boer E, de Rotte MC, et al. Methotrexate polyglutamates in erythrocytes are associated with lower disease activity in juvenile idiopathic arthritis patients. Ann Rheum Dis. 2015 Feb;74(2):402–407. PubMed PMID: 24288013.
- Takahashi C, Kaneko Y, Okano Y, et al. Association of erythrocyte methotrexate-polyglutamate levels with the efficacy and hepatotoxicity of methotrexate in patients with rheumatoid arthritis: a 76-week prospective study. RMD Open. 2017;3(1):e000363.
- den Boer E, de Rotte MC, Pluijm SM, et al. Determinants of erythrocyte methotrexate polyglutamate levels in rheumatoid arthritis. J Rheumatol. 2014 Nov;41(11):2167–2178. PubMed PMID: 25225283.
- den Boer E, Meesters RJ, van Zelst BD, et al. Measuring methotrexate polyglutamates in red blood cells: a new LC-MS/MS-based method. Anal Bioanal Chem. 2013 Feb;405(5):1673–1681. PubMed PMID: 23239179.
- Muller IB, Heydari P, Lin M, et al. AB0236 Development and validation of a sensitive lc-ms/ms-based method for analysis of enzymatic activity of folylpolyglutamate synthetase and methotrexate polyglutamates in peripheral blood mononuclear cells of rheumatoid arthritis patients. Ann Rheum Dis. 2018;77:1300.
- Rots MG, Pieters R, Peters GJ, et al. Role of folylpolyglutamate synthetase and folylpolyglutamate hydrolase in methotrexate accumulation and polyglutamylation in childhood leukemia. Blood. 1999 Mar 1;93(5):1677–1683. PubMed PMID: 10029597.
- Wojtuszkiewicz A, Assaraf YG, Hoekstra M, et al. The association of aberrant folylpolyglutamate synthetase splicing with ex vivo methotrexate resistance and clinical outcome in childhood acute lymphoblastic leukemia. Haematologica. 2016 Jul;101(7):e291–4. PubMed PMID: 27036162; PubMed Central PMCID: PMC5004475.
- van der Wilt CL, Cloos J, de Jong M, et al. Screening of colon tumor cells and tissues for folylpolyglutamate synthetase activity. Oncol Res. 1995;7(6):317–321. PubMed PMID: 8527866.