ABSTRACT
Introduction
Circulating tumor and disseminated cancer cells can be detected after surgical removal of the primary tumor in non-metastatic patients. Despite being considered the prime targets of adjuvant therapy, they have not been implemented into clinical decision making yet.
Areas covered
Here we review the recent progress in understanding the biology of circulating tumor cells and disseminated cancer cells as well as the technical challenges associated with quantification, isolation, and preclinical model development. We highlight the first examples of clinical studies in which metastasis founder cells have been used as surrogate markers in adjuvant cancer patients and address the current hurdles in implementing these in routine clinical application. Finally, we provide a perspective on how the combination of technologies to detect and isolate metastasis founders, single-cell multi-omics, development of preclinical models, and their drug responses in specific niches could improve personalized adjuvant treatment strategies.
Expert opinion
The specific target cells of adjuvant cancer treatment are metastasis founder cells that remain in the body of the patients after surgical removal of the primary tumor. We, therefore, believe that the success of adjuvant therapies will be improved by implementing circulating and disseminated cancer cells in future clinical decision making.
1. Introduction
Precision oncology defines the strategy to match individual cancer patients with the best available therapy. The drug selection is based on molecular, cellular, or functional criteria that are thought to determine treatment responses. In metastasized patients, therapies targeting driver mutations that confer strong growth advantage, e.g., BRAF mutation in melanoma, or EGFR mutation in lung cancer patients initially shows strong efficacy, high response rates, and significant reduction of tumor mass. However, unfortunately in most patients relapses occur [Citation1–]. Most targeted approaches in metastatic patients therefore successfully prolong patient survival by eliminating a predominant cancer cell clone, while therapy-induced selection pressure finally leads to acquisition of resistance mechanisms or growth advantage of rare subclones within the heterogeneous pool of cancer cells [Citation4].
Consequently, the clinical success of personalized treatments is anticipated to be greater in early therapeutic settings when only a few cancer cells need to be eliminated. For instance, targeting minimal residual disease by adjuvant therapies to prevent metastatic relapses. In recent years, we have learned that only a scarce population of cancer cells within a primary tumor (PT) has the potential to survive all steps along the complex invasion–metastasis cascade and succeed in finally colonizing in distant organs [Citation5]. So-called circulating tumor cells (CTCs) in blood as well as disseminated cancer cells (DCCs) which can be detected in distant organs of non-metastasized cancer patients, have already overcome many hurdles in the metastatic cascade and their presence can be correlated with poor outcome in most cancers [Citation6,Citation7]. Despite this knowledge and the fact that two-thirds of cancer deaths are attributed to metastasis [Citation8], patient stratification for precision oncology in adjuvant therapy setting currently still relies on molecular information from PT cells, cells that in most patients have been removed by surgery. To successfully translate state of the art precision medicine approaches to adjuvant therapy, strategies have to be developed that include analysis of the earliest metastatic seeds, i.e., single CTC and DCC, optimally to prevent colonization in distant organs and outgrowth of metastases. However, the integration of single-cell analysis in personalized adjuvant treatment concepts is still hindered due to a range of clinical, biological, and technical challenges such as (i) routine tissue availability for quantification and isolation of single DCC and translation of multi-omics precision medicine tools to single cells; (ii) using CTCs/DCCs or their derivates as surrogate markers in adjuvant clinical studies; and (iii) establishment of preclinical CTC/DCC models for functional assays and drug testing.
Here, we review recent works to provide a better understanding of metastasis founder cell biology with direct implications for improving precision medicine in the adjuvant therapy setting. Finally, we offer a perspective on how PT-based personalized therapy concepts could be adapted to rare CTC-/DCC-based approaches for tailored treatment strategies targeting metastasis founder cells.
2. Identification of metastasis founder cells
Detection and quantification of DCCs have first been reported in the 1980s and 1990s [Citation9,Citation10] and their presence has been associated with reduced disease-free or overall survival in many different epithelial cancers [Citation6]. Even with most advanced high-resolution imaging technologies, it is currently not possible to detect single DCC within organs. As a result, studies on DCCs have been mainly conducted in accessible mesenchymal tissues, such as bone marrow (BM) or lymph nodes (LN). In these organs, DCCs can be detected by specific staining against epithelial antigens, i.e., Epithelial Cell Adhesion Molecule (EpCAM, CD326) or cytokeratins, in 10–60% of patients with 1–10 cells per 2 million bone marrow cells [Citation6]. Unfortunately, neither automated nor FDA approved systems currently exist for the detection of DCCs. Although several tools have been described [Citation11], the consensus protocol for detection of DCCs still relies on cytology staining and manual cell isolation by micromanipulation hampering its integration in routine diagnostic workflows [Citation12].
In contrast, CTCs can be accessed by a minimally invasive blood draw that can be taken serially and has led to the use of the term ‘liquid biopsy’. Although not as progressed along the metastatic cascade as DCCs, during the last decade CTCs have been extensively investigated. Semiautomated workflows have been established to reliably detect CTCs in patients with epithelial cancers [Citation13,Citation14], which in the case of the CellSearch system has led to FDA approval for quantification of CTCs in breast, colorectal, and prostate cancers [Citation15]. CTC quantification could be applied as a valuable marker in metastatic patients for risk stratification and monitoring of therapeutic efficacy with a detection threshold of 2–5 CTCs per 7.5 ml blood across cancer types [Citation16–19]. Although the presence of CTCs in early cancer, typically taken before surgical removal of the PT, is also correlated with reduced progression-free and overall survival, their abundance is even lower compared to patients with metastases [Citation20]. For instance, in metastatic stage IV breast cancer, greater than or equal to 5 CTCs/7.5 ml blood can be detected in 50% of patients [Citation21], while only in 20% of stage I–III patients more than a single CTC/7.5 ml blood can be found [Citation22].
3. Molecular characterization of metastasis founder cells
Since not all early CTC or DCC positive patients are doomed to develop metastases, it will be important to identify and fully characterize the fraction of actual metastasis founder cells. While cancer stem cells (CSC) are well described as a small subset of cells responsible for sustaining tumorigenesis by self-renewal and differentiation in the context of primary tumors [Citation23] less is known about stem-like phenotypes of CTCs or DCCs, since it is still unknown whether single cells or clusters predominantly initiate metastases and to what extent other cell types within the metastatic microenvironment influence the process. To that end, molecular characterization of single CTC/DCC can provide important insights. To date, molecular analysis of early CTCs/DCCs has been mainly focused on genomic heterogeneity and evolution of cancer progression. In light of the data showing that most DCCs present fewer and discordant genomic abnormalities compared to their matched PT, it is fair to presume that they seed early during PT development and evolve at distant organs independently [Citation24–28]. Several studies have shown that even actionable targets are disparate in early CTCs/DCCs and PT. In esophageal cancer, HER2 expression in DCCs has been shown to significantly differ from matched PT, and interestingly, HER2 gain on single DCCs, but not in PT, correlated with a high risk for early death [Citation26,29]. In melanoma, the presence of BRAFV600E mutation was inconsistent between PT and LN-derived DCCs [Citation28]. Discordance between DCCs and matched PT has also been reported for the expression of estrogen receptor as well as the presence of PI3K mutations in breast cancer [Citation30–32]. With regard to HER2 expression, discordance between PT has been observed for both DCCs and CTCs in non-metastatic breast cancer patients [Citation33–35] indicating that adjuvant therapies could be improved by implementation of routine CTC/DCC-based stratification.
Currently, the majority of the studies investigating molecular features of single CTC/DCC focus on the analysis of either genomic abnormalities or gene expression data [Citation36]. As reported in recent tumor tissue-based precision medicine studies multi-omics approaches such as combined genomics and transcriptomics analysis can more precisely predict individual patient’s responses to therapies [Citation37–39]. However, despite progress in establishing multi-omics approaches for single cells [Citation40], considerable effort is still needed to translate these technologies and especially their integrative bioinformatics data analysis into comprehensive diagnostic workflows for rare single cells, such as CTCs and DCCs.
4. Metastasis founder cells as surrogate markers for adjuvant treatment success
In addition to the discussed technological hurdles, improved and especially expedited assessment of clinical outcomes for novel personalized treatment strategies tailored to metastasis founder cells are needed. Currently, adjuvant studies based on classical clinical endpoints such as survival or disease progression can take decades depending on cancer type. First studies corroborate the utility of CTCs as well as DCCs as surrogate markers for assessment of adjuvant therapy success. Gruber et al. repetitively quantified DCCs in BM of breast cancer patients during surgery for up to 24 months after PT removal. They observed that the majority of initially DCC-positive patients became DCC-negative in the course of adjuvant treatment and that persistence of DCCs after treatment was associated with disease recurrence [Citation41]. Xenidis et al. quantified CK-19 mRNA-positive CTC by RT-PCR in blood samples of patients with early breast cancer before and after adjuvant chemotherapy. 51% of initially DCC-positive patients became DCC-negative after adjuvant chemotherapy and those patients that remained or subsequently became DCC-positive post-chemotherapy had an increased risk for clinical relapse [Citation42]. These promising data indicate that CTCs/DCCs have the potential to be used as a tool for assessing the efficacy of adjuvant therapies, to shorten the duration of clinical trials for testing new drugs and for early detection of non-responsive patient populations. Furthermore, better stratification of patients benefiting from adjuvant therapies is important to avoid overtreatment and unnecessary therapy-related side effects. In this context, in a retrospective study the success of adjuvant radiotherapy was associated with CTC positivity in breast cancer [Citation43]. In line with this study, the detection of DCCs in BM of patients with early breast cancer was associated with the success of regional radiation and systemic hormone therapy for locoregional relapse [Citation44,Citation45].
Moreover, few trials have shown that secondary adjuvant therapies can be guided by quantification or even by treatment relevant information of CTCs/DCCs. Geogoulias et al. quantified chemotherapy-resistant CTCs in stage I–III breast cancer patients and showed that subsequent trastuzumab treatment can eliminate CTCs and reduce the risk for disease recurrence [Citation46]. In a second study, repetitive quantification of DCCs in BM of early breast cancer patients provided evidence that secondary adjuvant therapy can be guided by DCC quantification [Citation47]. In the future, additional studies are needed to link the presence of CTCs/DCCs or better their molecular features to the treatment outcome of patients with adjuvant therapies and to develop tailored strategies for specific eradication of these metastatic progenitors.
5. Preclinical models representing patient-derived metastasis founder cells
A major barrier for a better understanding of the biology of metastasis founder cells and the development of improved adjuvant treatments constitutes the lack of functional data from representative preclinical models. Beyond the correlation between DCC detection and the clinical outcome of patients, there is still only little functional evidence as to whether DCCs bear metastasis-inducing capacity in general, and if so which DCC populations in particular. Consequently, experimental data addressing seeding, metastatic colonization and drug target/pathway identification rely mainly on mouse models and/or human cell lines derived from PT or metastases that genetically and presumably also functionally differ from human DCCs [Citation25,Citation48,Citation49]. Reports addressing specific model generation from patient-derived DCCs are rare, given the cultivation and expansion of DCCs is challenging on account of their low abundance and the difficulties associated with isolation of viable DCCs. In the case of BM, short-term culture of cytokeratin positive cells from early-stage prostate, breast, colon, and kidney cancer patients has been demonstrated [Citation50,Citation51]. Only selective immortalization of micrometastatic carcinoma cells using integration of SV40 DNA has enabled long-term cultivation of BM-derived DCCs from prostate, breast, lung, and colon cancer patients without overt metastases [Citation52]. In addition, our group was recently able to successfully develop stable DCC-derived models from LN of non-metastatic melanoma patients at early stages of metastatic progression using a combination of in vitro spheroid culture and transplantation into NSG mice [Citation28]. Interestingly, model generation was possible only if DCCs were derived from small colonies, while not possible for LN with single DCC [Citation28].
Efforts in generating DCC-derived models might benefit from recent technical developments made in the field of liquid biopsy through optimizing the isolation and culturing conditions of CTCs from metastatic patients. Within the last decades a range of methods have been developed to specifically isolate CTCs [Citation53] and to cultivate these rare cells by optimized in vitro culture conditions such as spheroid and organoid cultures, or by in vivo transplantation into immunodeficient mice [Citation54–58]. To date, the generation of CTC-derived models has been reported for melanoma, breast, colorectal, lung, gastroesophageal, pancreatic, and prostate cancer patients () [Citation54–69]. CTC models from metastatic melanoma patients that recapitulate the patient’s disease and responses to therapies have been developed, highlighting the power of such models as potential ‘avatars’ for testing personalized treatments in the future [Citation60]. Furthermore, microfluidic devices have been shown to facilitate CTC expansion from metastatic patients as in vitro spheres, thus allowing rapid testing of CTC models for drug sensitivity by panels of single drugs and drug combinations [Citation58,Citation70]. However, generation of CTC-derived models from early-stage patients mirroring the target cells of adjuvant therapy has only been shown to be successful in short-term cultures, presumably owing to their even lower abundance [Citation71].
Table 1. Overview of studies reporting established CTC-derived preclinical models from advanced patients
6. Emerging technologies to expand and model patient-derived metastasis founder cells
Increasing the number of available CTCs for analysis could significantly increase the likelihood for generating models from non-metastatic patients. Recently, it has been calculated that the probability to detect sufficient number of CTCs is increasing with analyzing larger blood volumes [Citation72]. This hypothesis has been utilized by isolating CTCs from leukapheresis products instead of venous blood draw [Citation67,Citation73]. A small prospective study in 23 cancer patients showed an increase in CTC detection rate from 28% in peripheral blood to 72% in leukapheresis product [Citation73]. To that end, technologies that use biomedical devices for intravenous CTC capture have been developed [Citation74].
Additionally, a considerable amount of evidence indicates that the metastatic niche (e.g. hypoxia, immune cells, vasculature, and extracellular matrix) strongly influences the seeding capacity and phenotype of DCCs, and consequently their outgrowth as metastatic colonies [Citation75]. Exciting recent developments in establishing in vitro or ex vivo systems mimicking human organs can recapitulate metastatic niche microenvironment for investigating DCC biology and drug responses, e.g., natural matrices, synthetic hydrogels, and scaffolds, iPS-based niches, organ on a chip technologies, tissue engineering, or tissue slices from different organs might serve as ideal systems in the future [Citation76–84].
7. Conclusion
Detection of early DCCs and CTCs has been shown to correlate with systemic progression of most solid cancers and therefore at least some of these cells constitute founder of metastasis and target cells of adjuvant therapies. With state of the art technologies CTCs/DCCs can be isolated and their molecular analysis clearly indicates that information derived from these rare cells may potentially support the identification of novel and more therapeutically relevant targets. Their quantification and molecular information has been shown to serve as surrogate markers for adjuvant treatment and improve patient stratification. However, the hurdles in obtaining the respective tissues and lack of automated procedures to quantify, isolate, and analyze these rare cells and to develop representative preclinical models of metastatic founder cells has so far hindered their routine clinical application.
8. Expert opinion
The success of personalized cancer therapy will be markedly improved if the molecular, cellular, or functional information used for therapy selection would precisely mirror the features of cells responsible for disease progression. Therefore, it is essential for adjuvant therapy strategies to address the earliest seeds constituting rare metastasis founder cells rather than a heterogeneous pool of surgically removed PT cells only. Identification and more specifically targeting of metastasis founder cells would bear the potential to evolve the currently rather empirical concept of adjuvant treatment into a real precision medicine approach in order to more efficiently prevent metastatic relapses.
Early CTCs and DCCs have been shown to represent metastasis founder cells and their presence correlates with disease progression in most solid cancers. However, in order to implement early CTCs and DCCs in routine clinical decision-making practice there is yet much to be learned. What are the molecular and actionable features of CTCs/DCCs? How do these cells functionally respond to therapies within their metastatic niche microenvironment? Which of the detectable CTCs/DCCs bear metastasis-inducing capacity in patients? To answer these questions and translate bulk tumor tissue-based diagnostic tools into rare CTC/DCC approaches, improved methodologies for detection and isolation of, ideally viable, CTCs, and especially DCCs are needed. Here, much could be learned from the efforts and technological advancements in the field of liquid biopsy in metastasized patients which have to be transferred to earlier disease settings. Routine sampling of BM and LN should be integrated into automated workflows for detection and isolation of DCCs with standard diagnostic precision and accuracy ().
Figure 1. Perspective for implementation of CTCs and DCCs in adjuvant precision medicine. Routine sampling of BM and LN should be performed and technologies for identification and isolation of CTCs/DCCs should be improved. Existing multi-omics technologies including genome, DNA methylome, transcriptome, small RNAs, and proteome data as well as corresponding bioinformatics analysis tools addressing single metastasis founder cells will support the discovery of improved biomarkers. In parallel, first preclinical CTC/DCC models should become available for discovery of novel adjuvant therapies and/or repurposing of approved and investigational drugs in the context of adjuvant treatment. Molecular and functional data should be linked to clinical information to develop and initiate innovative clinical trials
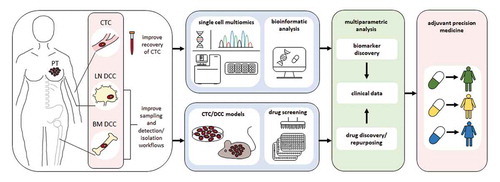
To better understand the biology of metastasis founder cells and to accurately predict therapy responses, the workflows for identification and isolation of early CTCs and DCCs should be combined with multi-omics data analysis. Single-cell technologies to obtain comprehensive information including genome, DNA methylome, transcriptome, small RNAs, and proteome data of single cells as well as corresponding bioinformatics analysis tools already exist, however they are yet to be applied to metastasis founder cells. This will help to identify the CTCs/DCCs that potentially colonize and discover specific biomarkers for patient stratification and activated pathways that can be therapeutically targeted. Additionally, much needed DCC- and early CTC-derived models could be potentially established using protocols developed for CTC expansion in the metastatic setting in combination with approaches to increase sample volume and thereby DCC/CTC number. As an alternative, considering recent advancements in the field of genome editing [Citation85,Citation86], one approach could also be to systematically introduce the earliest genetic, epigenetic and transcriptomic alterations identified by comprehensive molecular analysis of DCCs and early CTCs into normal nonmalignant cells by CRISPR-based technologies. In principle one could also reversely transform more advanced colonizing DCC models into their earlier and more immature counterparts by per se correcting specific molecular alternations which drive the transition of non-colonizing to colonizing DCCs by CRISPR technologies. Patient-derived or artificial preclinical models could then be subjected to high-throughput drug screens with follow-up hit validation in more complex systems representing progenitors in the context of their metastatic niche microenvironment (e.g., iPS niches, hydrogels, tissue slices), or alternatively perform small-to-medium scale drug screens directly in those complex multicellular systems for precision medicine and drug repurposing. Similar to recent studies on bulk tumor tissues [Citation37,Citation87–90], functional information could be combined with multi-omics data from single DCCs/CTCs to identify new drug targets and to predict responses to existing drugs. Ultimately, these findings have to be thoroughly examined and validated within innovatively designed clinical studies aimed at improving therapeutic strategies for adjuvant cancer patients in a truly precision medicine approach. If such comprehensive molecular and functional information could be linked to surrogate markers of adjuvant target cells in clinical settings, better stratification, improved personalized therapies and in case of resistance early therapy adjustment could be achieved. If these challenges can be tackled successfully, we anticipate that within a five year period sampling, identification and isolation of CTCs/DCCs will be improved, multi-omics data and first functional information for biomarker and drug discovery would be generated which can be examined within clinical trials in the long term (). Although there is yet much work to be done, the necessary technologies seem to exist for tackling the hurdles that currently impede the implementation of metastasis founder cells into routine clinical workflows.
Article highlights
Patient stratification for precision oncology in adjuvant therapy setting currently relies on molecular information from primary tumor
Detection of circulating tumor cells (CTCs) and disseminated cancer cells (DCCs) predict poor outcome in non-metastasized cancer patients
Actionable drug targets are disparate in early CTCs/DCCs and primary tumors
Early CTCs as well as DCCs can be used as surrogate markers for assessment of adjuvant therapy success
Protocols have been developed to establish preclinical models from metastatic CTCs that might pave the way for successful expansion of metastasis founder cells
Linkage of multi-omics single cell data to CTCs/DCCs as surrogate markers in clinical settings might improve personalized therapy strategies for prevention of metastases development in future
Declaration of interest
The authors have no other relevant affiliations or financial involvement with any organization or entity with a financial interest in or financial conflict with the subject matter or materials discussed in the manuscript apart from those disclosed.
Reviewers disclosure
Peer reviewers on this manuscript have no relevant financial relationships or otherwise to disclose.
Acknowledgments
The authors thank S. Pausch, K. Weidele and S. Treitschke for help with the figure and table, and C. A. Klein (CAK) for critical reading of the manuscript. This work was supported from grants of the Deutsche Krebshilfe (70112504 Schwerpunktprogramm ‘Translationale Onkologie’, DETECT CTC subproject 6 to BP and CAK) and of the Bavarian ministry of economic affairs, energy and technology (AZ 20-3410.1-1-2 to CAK).
Additional information
Funding
References
- Chapman PB, Hauschild A, Robert C, et al. Improved survival with vemurafenib in melanoma with BRAF V600E mutation. N Engl J Med. 2011 Jun 30;364(26):2507–2516.
- Hauschild A, Grob JJ, Demidov LV, et al. Dabrafenib in BRAF-mutated metastatic melanoma: a multicentre, open-label, phase 3 randomised controlled trial. Lancet. 2012 Jul 28;380(9839):358–365.
- Maemondo M, Inoue A, Kobayashi K, et al. Gefitinib or chemotherapy for non-small-cell lung cancer with mutated EGFR. N Engl J Med. 2010 Jun 24;362(25):2380–2388.
- Klein CA. Selection and adaptation during metastatic cancer progression. Nature. 2013 Sep 19;501(7467):365–372.
- Valastyan S, Weinberg RA. Tumor metastasis: molecular insights and evolving paradigms. Cell. 2011 Oct 14;147(2):275–292.
- Riethdorf S, Wikman H, Pantel K. Review: biological relevance of disseminated tumor cells in cancer patients. Int J Cancer. 2008 Nov 1;123(9):1991–2006.
- Toss A, Mu Z, Fernandez S, et al. CTC enumeration and characterization: moving toward personalized medicine. Ann Translat Med. 2014 Nov;2(11):108.
- Dillekas H, Rogers MS, Straume O. Are 90% of deaths from cancer caused by metastases? Cancer Med. 2019 Sep;8(12):5574–5576.
- Passlick B, Izbicki JR, Kubuschok B, et al. Immunohistochemical assessment of individual tumor cells in lymph nodes of patients with non-small-cell lung cancer. J Clin Oncol. 1994 Sep;12(9):1827–1832.
- Schlimok G, Funke I, Holzmann B, et al. Micrometastatic cancer cells in bone marrow: in vitro detection with anti-cytokeratin and in vivo labeling with anti-17-1A monoclonal antibodies. Proc Natl Acad Sci U S A. 1987 Dec;84(23):8672–8676.
- Magbanua MJ, Das R, Polavarapu P, et al. Approaches to isolation and molecular characterization of disseminated tumor cells. Oncotarget. 2015 Oct 13;6(31):30715–30729.
- Fehm T, Braun S, Muller V, et al. A concept for the standardized detection of disseminated tumor cells in bone marrow from patients with primary breast cancer and its clinical implementation. Cancer. 2006 Sep 1;107(5):885–892.
- Polzer B, Medoro G, Pasch S, et al. Molecular profiling of single circulating tumor cells with diagnostic intention. EMBO Mol Med. 2014 Nov;6(11):1371–1386.
- Lin E, Cao T, Nagrath S, et al. Circulating tumor cells: diagnostic and therapeutic applications. Annu Rev Biomed Eng. 2018 Jun;4(20):329–352.
- Allard WJ, Matera J, Miller MC, et al. Tumor cells circulate in the peripheral blood of all major carcinomas but not in healthy subjects or patients with nonmalignant diseases. Clin Cancer Res off J Am Assoc Cancer Res. 2004 Oct 15;10(20):6897–6904.
- Cohen SJ, Punt CJ, Iannotti N, et al. Relationship of circulating tumor cells to tumor response, progression-free survival, and overall survival in patients with metastatic colorectal cancer. J Clin Oncol. 2008 Jul 1;26(19):3213–3221.
- Cristofanilli M, Budd GT, Ellis MJ, et al. Circulating tumor cells, disease progression, and survival in metastatic breast cancer. N Engl J Med. 2004 Aug 19;351(8):781–791.
- Hiraiwa K, Takeuchi H, Hasegawa H, et al. Clinical significance of circulating tumor cells in blood from patients with gastrointestinal cancers. Ann Surg Oncol. 2008 Nov;15(11):3092–3100.
- Thalgott M, Rack B, Maurer T, et al. Detection of circulating tumor cells in different stages of prostate cancer. J Cancer Res Clin Oncol. 2013 May;139(5):755–763.
- Rack B, Schindlbeck C, Juckstock J, et al. Circulating tumor cells predict survival in early average-to-high risk breast cancer patients. J Natl Cancer Inst. 2014 May 15;106(5):dju066.
- Bidard FC, Peeters DJ, Fehm T, et al. Clinical validity of circulating tumour cells in patients with metastatic breast cancer: a pooled analysis of individual patient data. Lancet Oncol. 2014 Apr;15(4):406–414.
- Janni WJ, Rack B, Terstappen LW, et al. Pooled analysis of the prognostic relevance of circulating tumor cells in primary breast cancer. Clin Cancer Res off J Am Assoc Cancer Res. 2016 May 15;22(10):2583–2593.
- Visvader JE, Lindeman GJ. Cancer stem cells: current status and evolving complexities. Cell Stem Cell. 2012 Jun 14;10(6):717–728.
- Holcomb IN, Grove DI, Kinnunen M, et al. Genomic alterations indicate tumor origin and varied metastatic potential of disseminated cells from prostate cancer patients. Cancer Res. 2008 Jul 15;68(14):5599–5608.
- Hosseini H, Obradovic MMS, Hoffmann M, et al. Early dissemination seeds metastasis in breast cancer. Nature. 2016 Dec 22;540(7634):552–558.
- Stoecklein NH, Hosch SB, Bezler M, et al. Direct genetic analysis of single disseminated cancer cells for prediction of outcome and therapy selection in esophageal cancer. Cancer Cell. 2008 May;13(5):441–453.
- Weckermann D, Polzer B, Ragg T, et al. Perioperative activation of disseminated tumor cells in bone marrow of patients with prostate cancer. J Clin Oncol. 2009 Apr 1;27(10):1549–1556.
- Werner-Klein M, Scheitler S, Hoffmann M, et al. Genetic alterations driving metastatic colony formation are acquired outside of the primary tumour in melanoma. Nat Commun. 2018 Feb 9;9(1):595.
- Hoffmann M, Pasch S, Schamberger T, et al. Diagnostic pathology of early systemic cancer: ERBB2 gene amplification in single disseminated cancer cells determines patient survival in operable esophageal cancer. Int J Cancer. 2018 Feb 15;142(4):833–843.
- Ditsch N, Mayer B, Rolle M, et al. Estrogen receptor expression profile of disseminated epithelial tumor cells in bone marrow of breast cancer patients. Recent Results Cancer Res Fortschritte der Krebsforschung Progres Dans les Recherches Sur le Cancer. 2003;162:141–147.
- Fehm T, Krawczyk N, Solomayer EF, et al. ERalpha-status of disseminated tumour cells in bone marrow of primary breast cancer patients. BCR. 2008;10(5):R76.
- Magbanua MJM, Rugo HS, Hauranieh L, et al. Genomic and expression profiling reveal molecular heterogeneity of disseminated tumor cells in bone marrow of early breast cancer. NPJ Breast Cancer. 2018;4:31.
- Krawczyk N, Banys M, Neubauer H, et al. HER2 status on persistent disseminated tumor cells after adjuvant therapy may differ from initial HER2 status on primary tumor. Anticancer Res. 2009 Oct;29(10):4019–4024.
- Rack B, Zombirt E, Trapp E, et al. Comparison of HER2 expression in primary tumor and disseminated tumor cells in the bone marrow of breast cancer patients. Oncology. 2016;90(4):232–238.
- Wulfing P, Borchard J, Buerger H, et al. HER2-positive circulating tumor cells indicate poor clinical outcome in stage I to III breast cancer patients. Clin Cancer Res off J Am Assoc Cancer Res. 2006 Mar 15;12(6):1715–1720.
- Ellsworth DL, Blackburn HL, Shriver CD, et al. Single-cell sequencing and tumorigenesis: improved understanding of tumor evolution and metastasis. Clini Transl Med. 2017 Dec;6(1):15.
- Lee JK, Liu Z, Sa JK, et al. Pharmacogenomic landscape of patient-derived tumor cells informs precision oncology therapy. Nature Genet. 2018 Oct;50(10):1399–1411.
- Rodon J, Soria JC, Berger R, et al. Genomic and transcriptomic profiling expands precision cancer medicine: the WINTHER trial. Nat Med. 2019 May;25(5):751–758.
- Yu KH, Snyder M. Omics profiling in precision oncology. Mol Cell Proteomics. 2016 Aug;15(8):2525–2536.
- Chappell L, Russell AJC, Voet T. Single-cell (multi)omics technologies. Annu Rev Genomics Hum Genet. 2018 Aug;31(19):15–41.
- Gruber I, Fehm T, Taran FA, et al. Disseminated tumor cells as a monitoring tool for adjuvant therapy in patients with primary breast cancer. Breast Cancer Res Treat. 2014 Apr;144(2):353–360.
- Xenidis N, Ignatiadis M, Apostolaki S, et al. Cytokeratin-19 mRNA-positive circulating tumor cells after adjuvant chemotherapy in patients with early breast cancer. J Clin Oncol. 2009 May 1;27(13):2177–2184.
- Goodman CR, Seagle BL, Friedl TWP, et al. Association of circulating tumor cell status with benefit of radiotherapy and survival in early-stage breast cancer. JAMA Oncol. 2018 Aug 1;4(8):e180163.
- Bidard FC, Kirova YM, Vincent-Salomon A, et al. Disseminated tumor cells and the risk of locoregional recurrence in nonmetastatic breast cancer. Ann Oncol. 2009 Nov;20(11):1836–1841.
- Mignot F, Loirat D, Dureau S, et al. Disseminated tumor cells predict efficacy of regional nodal irradiation in early stage breast cancer. Int J Radiat Oncol Biol Phys. 2019 Feb 1;103(2):389–396.
- Georgoulias V, Bozionelou V, Agelaki S, et al. Trastuzumab decreases the incidence of clinical relapses in patients with early breast cancer presenting chemotherapy-resistant CK-19mRNA-positive circulating tumor cells: results of a randomized phase II study. Ann Oncol. 2012 Jul;23(7):1744–1750.
- Naume B, Synnestvedt M, Falk RS, et al. Clinical outcome with correlation to disseminated tumor cell (DTC) status after DTC-guided secondary adjuvant treatment with docetaxel in early breast cancer. J Clin Oncol. 2014 Dec 1;32(34):3848–3857.
- Harper KL, Sosa MS, Entenberg D, et al. Mechanism of early dissemination and metastasis in Her2+ mammary cancer. Nature. 2016 Dec 22;540(7634):588–592.
- Suzuki M, Mose ES, Montel V, et al. Dormant cancer cells retrieved from metastasis-free organs regain tumorigenic and metastatic potency. Am J Pathol. 2006 Aug;169(2):673–681.
- Gangnus R, Langer S, Breit E, et al. Genomic profiling of viable and proliferative micrometastatic cells from early-stage breast cancer patients. Clin Cancer Res off J Am Assoc Cancer Res. 2004 May 15;10(10):3457–3464.
- Solakoglu O, Maierhofer C, Lahr G, et al. Heterogeneous proliferative potential of occult metastatic cells in bone marrow of patients with solid epithelial tumors. Proc Natl Acad Sci U S A. 2002 Feb 19;99(4):2246–2251.
- Putz E, Witter K, Offner S, et al. Phenotypic characteristics of cell lines derived from disseminated cancer cells in bone marrow of patients with solid epithelial tumors: establishment of working models for human micrometastases. Cancer Res. 1999 Jan 1;59(1):241–248.
- Banko P, Lee SY, Nagygyorgy V, et al. Technologies for circulating tumor cell separation from whole blood. J Hematol Oncol. 2019 May 14;12(1):48.
- Baccelli I, Schneeweiss A, Riethdorf S, et al. Identification of a population of blood circulating tumor cells from breast cancer patients that initiates metastasis in a xenograft assay. Nat Biotechnol. 2013 Jun;31(6):539–544.
- Cayrefourcq L, Mazard T, Joosse S, et al. Establishment and characterization of a cell line from human circulating colon cancer cells. Cancer Res. 2015 Mar 1;75(5):892–901.
- Gao D, Vela I, Sboner A, et al. Organoid cultures derived from patients with advanced prostate cancer. Cell. 2014 Sep 25;159(1):176–187.
- Hodgkinson CL, Morrow CJ, Li Y, et al. Tumorigenicity and genetic profiling of circulating tumor cells in small-cell lung cancer. Nature Med. 2014 Aug;20(8):897–903.
- Yu M, Bardia A, Aceto N, et al. Cancer therapy. Ex vivo culture of circulating breast tumor cells for individualized testing of drug susceptibility. Science. 2014 Jul 11;345(6193):216–220..
- Brungs D, Minaei E, Piper AK, et al. Establishment of novel long-term cultures from EpCAM positive and negative circulating tumour cells from patients with metastatic gastroesophageal cancer. Sci Rep. 2020 Jan 17;10(1):539.
- Girotti MR, Gremel G, Lee R, et al. Application of sequencing, liquid biopsies, and patient-derived xenografts for personalized medicine in melanoma. Cancer Discov. 2016 Mar;6(3):286–299.
- Zhang L, Ridgway LD, Wetzel MD, et al. The identification and characterization of breast cancer CTCs competent for brain metastasis. Sci Transl Med. 2013 Apr 10;5(180):180ra48.
- Morrow CJ, Trapani F, Metcalf RL, et al. Tumourigenic non-small-cell lung cancer mesenchymal circulating tumour cells: a clinical case study. Ann Oncol. 2016 Jun;27(6):1155–1160.
- Grillet F, Bayet E, Villeronce O, et al. Circulating tumour cells from patients with colorectal cancer have cancer stem cell hallmarks in ex vivo culture. Gut. 2017 Oct;66(10):1802–1810.
- Pereira-Veiga T, Abreu M, Robledo D, et al. CTCs-derived xenograft development in a triple negative breast cancer case. Int J Cancer. 2019 May 1;144(9):2254–2265.
- Drapkin BJ, George J, Christensen CL, et al. Genomic and functional fidelity of small cell lung cancer patient-derived xenografts. Cancer Discov. 2018 May;8(5):600–615.
- Vishnoi M, Liu NH, Yin W, et al. The identification of a TNBC liver metastasis gene signature by sequential CTC-xenograft modeling. Mol Oncol. 2019 Sep;13(9):1913–1926.
- Faugeroux V, Pailler E, Oulhen M, et al. Genetic characterization of a unique neuroendocrine transdifferentiation prostate circulating tumor cell-derived eXplant model. Nat Commun. 2020 Apr 20;11(1):1884.
- Koch C, Kuske A, Joosse SA, et al. Characterization of circulating breast cancer cells with tumorigenic and metastatic capacity. EMBO Mol Med. 2020 Sep 7;12(9):e11908.
- Rivera-Baez L, Lohse I, Lin E, et al. Expansion of circulating tumor cells from patients with locally advanced pancreatic cancer enable patient derived xenografts and functional studies for personalized medicine. Cancers (Basel). 2020 Apr 20;12:4.
- Khoo BL, Grenci G, Lim JSY, et al. Low-dose anti-inflammatory combinatorial therapy reduced cancer stem cell formation in patient-derived preclinical models for tumour relapse prevention. Br J Cancer. 2019 Feb;120(4):407–423.
- Zhang Z, Shiratsuchi H, Lin J, et al. Expansion of CTCs from early stage lung cancer patients using a microfluidic co-culture model. Oncotarget. 2014 Dec 15;5(23):12383–12397.
- Stoecklein NH, Fischer JC, Niederacher D, et al. Challenges for CTC-based liquid biopsies: low CTC frequency and diagnostic leukapheresis as a potential solution. Expert Rev Mol Diagn. 2016;16(2):147–164.
- Fischer JC, Niederacher D, Topp SA, et al. Diagnostic leukapheresis enables reliable detection of circulating tumor cells of nonmetastatic cancer patients. Proc Natl Acad Sci U S A. 2013 Oct 8;110(41):16580–16585.
- Gorges TM, Penkalla N, Schalk T, et al. Enumeration and molecular characterization of tumor cells in lung cancer patients using a novel in vivo device for capturing circulating tumor cells. Clin Cancer Res off J Am Assoc Cancer Res. 2016 May 1;22(9):2197–2206.
- Peinado H, Zhang H, Matei IR, et al. Pre-metastatic niches: organ-specific homes for metastases. Nat Rev Cancer. 2017 May;17(5):302–317.
- Narkhede AA, Crenshaw JH, Crossman DK, et al. An in vitro hyaluronic acid hydrogel based platform to model dormancy in brain metastatic breast cancer cells. Acta Biomater. 2020 Apr 15;107:65–77.
- Pradhan S, Slater JH. Tunable hydrogels for controlling phenotypic cancer cell states to model breast cancer dormancy and reactivation. Biomaterials. 2019 Sep;215:119177.
- Carpenter RA, Kwak JG, Peyton SR, et al. Implantable pre-metastatic niches for the study of the microenvironmental regulation of disseminated human tumour cells. Nature Biomed Eng. 2018 Dec;2(12):915–929.
- Birey F, Andersen J, Makinson CD, et al. Assembly of functionally integrated human forebrain spheroids. Nature. 2017 May 4;545(7652):54–59.
- Mazzocchi AR, Rajan SAP, Votanopoulos KI, et al. In vitro patient-derived 3D mesothelioma tumor organoids facilitate patient-centric therapeutic screening. Sci Rep. 2018 Feb 13;8(1):2886.
- Misra S, Moro CF, Del Chiaro M, et al. Ex vivo organotypic culture system of precision-cut slices of human pancreatic ductal adenocarcinoma. Sci Rep. 2019 Feb 14;9(1):2133.
- Neal JT, Li X, Zhu J, et al. Organoid modeling of the tumor immune microenvironment. Cell. 2018 Dec 13;175(7):1972–1988 e16.
- Plummer S, Wallace S, Ball G, et al. A human iPSC-derived 3D platform using primary brain cancer cells to study drug development and personalized medicine. Sci Rep. 2019 Feb 5;9(1):1407.
- Skardal A, Devarasetty M, Forsythe S, et al. A reductionist metastasis-on-a-chip platform for in vitro tumor progression modeling and drug screening. Biotechnol Bioeng. 2016 Sep;113(9):2020–2032.
- Adli M. The CRISPR tool kit for genome editing and beyond. Nat Commun. 2018 May 15;9(1):1911.
- Matano M, Date S, Shimokawa M, et al. Modeling colorectal cancer using CRISPR-Cas9-mediated engineering of human intestinal organoids. Nat Med. 2015 Mar;21(3):256–262.
- Gao H, Korn JM, Ferretti S, et al. High-throughput screening using patient-derived tumor xenografts to predict clinical trial drug response. Nature Med. 2015 Nov;21(11):1318–1325.
- Izumchenko E, Paz K, Ciznadija D, et al. Patient-derived xenografts effectively capture responses to oncology therapy in a heterogeneous cohort of patients with solid tumors. Ann Oncol. 2017 Oct 1;28(10):2595–2605.
- Pauli C, Hopkins BD, Prandi D, et al. Personalized in vitro and in vivo cancer models to guide precision medicine. Cancer Discov. 2017 May;7(5):462–477.
- Vlachogiannis G, Hedayat S, Vatsiou A, et al. Patient-derived organoids model treatment response of metastatic gastrointestinal cancers. Science. 2018 Feb 23;359(6378):920–926.