Abstract
We studied morphology and phylogeny of a Chara vulgaris (Charophytes, Charales) population sampled from the thermal “Springs of Moses” (Ayun Musa, Sinai, Egypt). Morphology of freshly sampled and cultivated materials was compared in order to quantify the influence of culturing conditions on morphological features. Cultivated material was shorter and had a smaller internode diameter than the freshly collected material. The bracteoles in the freshly collected material were distinctly longer (about 4–12 times the length of an oogonium) than those in the cultured material (about 2–4 times). Moreover, oogonia were slightly longer in the cultured material. Genetic analysis of the matK gene clearly identified the sampled specimens as Chara vulgaris. However, the freshly collected material was found to differ from “typical” Chara vulgaris populations in one morphological and one life-cycle feature. The thalli were fairly delicate, and the antheridia were shed early in both freshly collected and cultured materials, while oogonia were still in place. Most of the plants prepared and observed in this study were found to bear plenty of oogonia, whilst antheridia were often missing. Antheridia were only present on the newly formed branchlets at the stem apex. The peculiar morphology and life-cycle characteristics of this C. vulgaris population may be adaptations to this highly isolated and selective desert freshwater habitat.
Introduction
Charophytes, i.e. extent and fossil members of the order Charales plus the members of the extinct orders Sycidiales and Moellerinales (Schneider et al. Citation2015) are phylogenetically closely related to modern land plants (McCourt, Delwiche, and Karol Citation2004; Timme, Bachvaroff, and Delwiche Citation2012). They are considered to be one of the most severely threatened groups of algal macrophytes (Azzella Citation2014), and are sensitive to water pollution and eutrophication (Azzella Citation2014; Blindow Citation1992). Their susceptibility to water pollution allows them to be used effectively as environmental bioindicators (Doege et al. Citation2016; Melzer Citation1999). Chara vulgaris L. usually occurs in different freshwater habitats worldwide (Wood and Imahori Citation1965), and can occasionally be found in mineral springs and high conductivity water near the sea (e.g. Caisová and Gąbka Citation2009; Krause Citation1997).
Springs are key, and often isolated, habitats with unique characteristics (Cantonati, Gerecke, and Bertuzzi Citation2006; Shaaban, Mansour, and Saber Citation2015). They are mainly threatened by water overdraft (e.g. Cantonati et al. Citation2016), but have potential to contribute to the conservation of endangered and rare freshwater biodiversity (e.g. Cantonati, Komárek, and Montejano Citation2015; Cantonati et al. Citation2012; Saber, Ichihara, and Cantonati Citation2016). Springs are quite diverse ecotones that link aquatic and terrestrial ecosystems which can be classified using different criteria (see Glazier Citation2009; Nowicka-Krawczyk and Żelazna-Wieczorek Citation2016). In general, springs can be considered as biodiversity hotspots and act as refugia for threatened species, and are thus suitable sites to study the distribution of several taxa from different groups of organisms as driven by environmental and biogeographical factors (Bravakos et al. Citation2016; Cantonati et al. Citation2012; Rosati et al. Citation2014).
The only published information on the phycological diversity (including charophytes) in the Springs of Moses was the early work of Braun (Citation1868), Hume (Citation1906), and Von Hustedt (Citation1949). Knowledge about stoneworts in Egypt in general is limited and incomplete (e.g. Corillion and Guerlesquin Citation1971, Citation1972; Shaaban Citation1994; Shaaban, Mansour, and Saber Citation2015). Corillion and Guerlesquin (Citation1971, Citation1972), working on herbarium specimens using formerly identified taxa, showed that there were a total of 26 different species and infraspecies of Characeae including Chara vulgaris, and certain specimens were in need of further morphotaxonomic revision, such as C. vulgaris var. inconnexa (T.F.Allen) R.D.Wood morphologically close to the f. hippelliana (Vilhelm) R.D.Wood. However, some taxa have been reported from different aquatic habitats but without any information about their adaptable morphological features with respect to environmental characteristics. Shaaban (Citation1985), for instance, reported C. foetida A. Braun (heterotypic synonym for C. vulgaris L.) at Ain Radi, Siwa Oasis, the Western Desert. In a geological and paleobotanical study, Zalat (Citation1996) recorded well-preserved C. vulgaris gyrogonite assemblages from Holocene lacustrine sediments of the old Qarun Lake at the north of El-Fayoum Depression. El-Naggar (Citation1995) discussed the comparative biochemical components of vegetative and reproductive stages of C. vulgaris material collected from a freshwater streamlet near the Abu Hatab village (El-Sharkeia governorate) in 1992. This is the last published record of C. vulgaris in Egypt.
Adaptive phenotypic plasticity in land plants (e.g. Callahan, Dhanoolal, and Ungerer Citation2005; Merila and Hendry Citation2014; Palacio-López et al. Citation2015) and algae (e.g. Leliaert et al. Citation2014; Saber et al. Citation2016; Verbruggen Citation2014) has been well discussed and documented. In general, many algal populations are known to exhibit substantial phenotypic variations, either as a result of genetically controlled polymorphism or environmentally induced plasticity (e.g. De Senerpont Domis et al. Citation2003; Lambert et al. Citation2013; Leliaert et al. Citation2014; Logares et al. Citation2007; Lurling Citation2003). For charophytes, comparable high morphological variability also exists within many morphospecies, and in particular local populations likely reflect special phenotypic adaptations to different natural ecosystems (Bociag et al. Citation2013; Boegle et al. Citation2007; Lambert et al. Citation2013; Sakayama et al. Citation2009; Schneider et al. Citation2015; Urbaniak Citation2010; Urbaniak and Combik Citation2013; Wood and Imahori Citation1965). Recent studies have indicated a high morphological variability within genetically closely related Chara taxa (Schneider et al. Citation2015, 2016). Little is known about the extent to which charophytes, particularly the cosmopolitan species C. vulgaris, show distinguished patterns of adaptive phenotypic plasticity in isolated and hydrochemically stable habitats, such as springs and their artificial counterpart “wells” (Barinova and Romanov Citation2015; Hu et al. Citation2009; Langangen Citation2008).
To our knowledge, few studies have discussed the effects of environmental parameters on specific morphotaxonomic features of Characeae, and few of them compared the differences in thallus morphology of specimens cultivated indoors with naturally occurring specimens. For instance, Andrews, McInroy, and Raven (Citation1984) pointed out that the optimum conditions for rhizoid development and shoot growth of Chara hispida and Nitella flexilis are obtained using a substrate of 425–710 μm sand particles and a solution of inorganic salts dissolved in distilled water. Sato et al. (Citation2014) investigated the diverse effects of light intensity on C. braunii under laboratory conditions and observed that the thalli grow under high light illumination without the formation of reproductive organs, but under low light intensity thalli formed many antheridia without the corresponding oogonia. They speculated that thalli saved their energetic resources and allocated them to the formation of lone antheridia because oogonia formation requires more energy. This adaptive mechanism is also likely to occur in nature. Sanjuan et al. (Citation2017) tested the influence of irradiance and temperature on intraspecific gyrogonite variations of C. vulgaris and C. globularis and indicated that gyrogonites tended to increase their sizes as irradiance and temperature increased, but in extreme conditions (1000 μmol PAR m−2 s−1 and 35 °C) an opposite trend was recorded with gyrogonites becaming significantly smaller.
Here, we focus on the detailed morphological and phylogenetic characterization of a C. vulgaris population from isolated desert springs. We also compare the morphology of freshly sampled materials with that of cultured materials, in order to study which morphological and life-cycle traits may be influenced by the environment.
Materials and methods
Study area
Ayun Musa (29° 52′ 21.38″ N, 32° 39′ 00.41″ E), also called the Springs of Moses (Map ), is located on the main road of the east coast of the Gulf of Suez, about 20 km south of the Ahmed Hamdi Tunnel in South Sinai, close (c. 40 km) to Ras Sudr City, and about 250 km from Saint Catherine City, South Sinai. The Gulf of Suez coastal zones belong to a climatically hyper-arid region with cool winters and hot summers.
The Springs of Moses, which comprised 12 springs in the past, while today only seven contain water while the others dried out and disappeared, can be classified as basin-like limnocrenic depressions (c. 12–15 m deep) in the sandy ground. Obvious signs of anthropogenic impacts were noted during the sampling survey. The spring openings are partially shaded and surrounded by stones as a kind of protection and for water retention (Figs. A–B). However, the water levels inside the springs are usually low, and waters are “still to stagnant” throughout the year, with relatively minor seasonal water-level fluctuations, with maximum water levels of c. 50 cm (Saber, personal observation). Ayun Musa are located at an elevation of about 17 m a.s.l. Geologically, the area is generally flat, with some minor and scattered topographic highs. The stratigraphic column of this area, as inferred from some drilled wells, is characterized by thick Paleozoic rocks (Lashin Citation2013). The Lower Cretaceous aquifer is the main source of underground water (Abd El-Rahman Citation2001). The heat of the Springs of Moses is probably derived from high heat flow and deep circulation controlled by faults associated with the opening of the Red Sea and the Gulf of Suez rifts (Kaiser and Ahmed Citation2013; Morgan, Boulos, and Swanberg Citation1983; Sturchio and Arehart Citation1996). No hydrochemical analyses could be conducted in the frame of our study, but the physical and chemical characteristics of the Ayun Musa thermal springs and other nearby artesian wells were assessed from the available literature. The Springs of Moses are saline (mineral) springs close to the coast. The high conductivity and mineral content (T.D.S. = 2600 mg. l−1) is determined primarily by chloride (983 mg. l−1) and sodium (652 mg. l−1), and secondarily by sulphates (378 mg. l−1; Sturchio and Arehart Citation1996). With regard to algal nutrients, unfortunately only data on silica (17.8 mg. l−1; Sturchio and Arehart Citation1996) and sulphates are available, and both are available in high amounts. The springs can further be defined as thermal, i.e. with average temperatures (31–34 °C) exceeding mean annual air temperature, which in the study area is around 22°C (Zahran and Willis Citation2009). However, following the exact definition, they are not “hot springs”, which should possess average temperature exceeding human-body temperature (e.g. Glazier Citation2009).
Sampling, culturing, and morphological analyses
A dense population of Chara vulgaris (Fig. C) was sampled on 13th November 2009 from three springs and mainly used for morphotaxonomic identification. A new collection was sampled in the same seasonal period on 23rd November 2015, and was characterized by the same morphological diagnostic features considered for the first collection. The latter specimens were chiefly used for indoor cultivation. There were no morphological variations among Chara populations sampled in the two collections, and they strictly belonged to one morphotype. The material was placed in clean sterile polyethylene terephthalate (P.E.T.) bottles, and transported chilled to the laboratory. In the lab, thallus pieces were cleaned well with distilled water to isolate unialgal strains of C. vulgaris. For indoor cultivation, the method described by El-Naggar (Citation1995) and Sakayama, Hara, and Nozaki (Citation2004) was followed with slight modifications. Shoot tips of freshly sampled material, composed of the topmost four nodes and three internodes, were carefully cut from the freshly collected plants, separated from their reproductive structures, and cultivated. The internode number three was planted in an upright position in a glass vessel (35 x 15 x 50 cm length/width/height) provided with silica sand as substrate and leaving only the topmost two smallest internodes to start the experiment. The vessel at the beginning of the experiment was filled with tap water with almost neutral pH 7.03, electrical conductivity 780 μS. cm−1, T.D.S. 400 mg. l−1, and major ions Ca2+, Na+, Cl-, and SO42− were 48.9, 85.9, 95.1, 69.9 mg l−1, respectively. The final sand-cultured medium, somewhat similar to waters of the Springs of Moses, was characterized by a pH value of 7.2, specific conductivity of 3.1 mS. cm−1, and a T.D.S. value of 2100 mg. l−1. Water was added when required to keep the cultivated plants submerged (twice during the cultivation period was sufficient). The inoculated glass vessel was kept on the windowsill of the lab and depended on the normal daylight illumination, relatively resembling growth conditions in the Springs of Moses and followed daily until stem elongation and formation of all life stages (c. 70 days): new internodes, branchlets, oogonia, antheridia, and oospores on the newly formed branchlets (Fig. D). The average air temperature (25 °C) and relative air humidity (45.2%) during the cultivation process were recorded daily around mid-day. At the same time, a part of the freshly collected material was fixed and preserved by adding formaldehyde to a final concentration of 4% (v/v).
Both freshly collected and cultured materials were observed and measured for the following taxonomically relevant morphometric features (a total of 50 measurements using a stage micrometer for each character and 25 different specimens were investigated): stem length and diameter, and so on for the other cortex structure; shape and arrangement of spine cells; stipulodes; internodes and their length ratio compared to branchlets; number and width of branchlets; shape, length, and width of terminal cells; number of bract-cells and their length relative to the length of the oogonia; and arrangement, shape, and dimensions of sex organs. Oospore details could be assessed only for the cultured material, since oospores did not occur in materials sampled in the field. Light microscope (LM) images were obtained by using a BEL® photonics microscope equipped with a Canon Powershot G12 digital camera. Plates were digitally manipulated using Adobe Photoshop (version CS 4, Adobe Systems Inc.). The nomenclature used to describe the morphology of the specimens followed Krause (Citation1997), and Caisová and Gąbka (Citation2009). Voucher specimens were deposited in the collections of the Phycology Unit (No. 341) of the Botany Department, Faculty of Science, at Ain Shams University, Cairo (Egypt).
DNA extraction, amplification, sequencing, and phylogenetic analysis
Genomic DNA from Chara material was isolated after Ballot et al. (Citation2014). Instead of horizontal vortexing, a bead-beating step (3 × 30 s, 6700 rpm) in a Precellys 24 bead beater (Bertin Technologies, Saint Quentin, France) was used to disrupt the cells. Amplification and sequencing of the matK gene region was conducted using newly designed primers charaBT2F (datatggcaacaycaaaagac) and charaBT2R (atacagaccatgcagcytt). Sequences were analysed and aligned using Seqassem (version 04/2008) and Align (version 03/2007) MS Windows-based manual sequence alignment editor (SequentiX - Digital DNA Processing, Klein Raden Germany) to obtain DNA sequence alignments, which were then corrected manually. Segments with highly variable and ambiguous regions and gaps, making proper alignment impossible, were excluded from the analyses. A matK set containing 546 positions was used for phylogenetic analyses. For comparison, several other samples of C. vulgaris, as well as other Chara species, were included in the phylogenetic analysis (Table ). Nitellopsis obtusa (AY170447) was used as an outgroup taxon in the matK tree. The data-set was analysed using the maximum likelihood (ML) algorithm in MEGA version 6 (Tamura et al. Citation2013). The method selected T92+G as the best-fitting evolutionary model for the matK gene region. ML analyses were performed with 1000 bootstrap replicates in MEGA version 6 (Tamura et al. Citation2013).
Statistical analysis
The data were statistically analysed using SPSS version 22.0 (IBM SPSS Statistics for Windows, Version 22.0. Armonk, NY: IBM Corp, 2013). The student’s independent t-test and Waller–Duncan test were used to analyse the statistical significance of differences between the morphotaxonomic features in the freshly sampled and cultured materials. All statements of significance were based on P ≤ 0.05. The results were expressed as mean ± standard deviation (S.D.).
Results
Morphology of freshly sampled material
The thallus of freshly sampled Chara vulgaris from the Springs of Moses was green in colour, slender, delicate, up to 50 cm tall, diplostichous, and aulacanthous (Tables , Fig. ). Cortical cells were distinctly spirally coiled, 13–17 cells. Spine cells were variable, solitary, scattered, short, papilliform, or elongated up to a length of one axis diameter, especially on older stems. Stipulodes were short, oblong-ovate, in two rows. Internodes were variable in length (2.5–8 cm long) compared to the branchlets (mostly 1–4 times as long as the branchlets), 472–602 μm in diameter. Branchlets: eight in a whorl, 378–448 μm wide, terminating with one sharply conical cell (Fig. ). Bracteoles four, well-developed, clearly much longer than the oogonium (about 4–12 times the length of the oogonium), with three corticated segments and ecorticated end parts commonly present and distinctly longer than the corticated parts (Fig. ). Plants were monoecious, bearing three to four pairs of gametangia per branchlet, and the antheridia shed early while oogonia were still in place (Fig. ). Oogonia without crown cells: 366–590 μm long x 355–437 μm wide, with 10–11 turns. Crown cells: 83–118 μm long x 71–80 μm wide. Antheridia: very rare, solitary, spherical, orange in colour, 366–375 μm in diameter. They could hardly be observed. Mature oospores were absent.
Table 1. Comparison of morphological and ecological characteristics of Chara vulgaris Linn. in this study and in reference literature.
Table 2. Key diagnostic features of Chara vulgaris from the Springs of Moses in freshly collected and cultured materials.
Figure (2–5). Some diagnostic characteristics of freshly sampled material of Chara vulgaris Linn. in this study. Scale bar = 1 cm (Fig. ); scale bar = 100 μm (Fig. ); scale bar = 1 mm (Fig. ); scale bar = 200 μm (Fig. ). (2) Macroscopic habitus of thallus showing long internodes. (3) Terminal branchlet cell. (4) Overview of branchlet node showing four well-developed bracteoles clearly much longer than oogonium. (5) Branchlet node with solitary dark-brown mature oogonium and absence of antheridium.
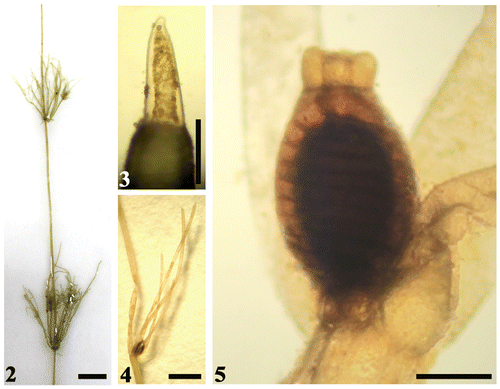
Morphology of cultured material
The morphology of cultured material showed some features that were clearly different from both the freshly collected material and other typical C. vulgaris populations reported in the literature (Tables , Figs ). Primarily, the cultured material was more delicate, and significantly shorter (20–25 cm tall) than the fresh material (40–50 cm tall). Antheridia were shed early, and usually formed only on the new branchlets at the stem apex (Figs ). They were not obviously dominant on the branchlets as the oogonia. Compared to the freshly collected material, cultured material had a thinner internode diameter (p = 0.001). The bracteoles in the freshly collected material (Fig. ) were distinctly longer (about 4–12 times the length of the oogonium) than those in the cultured one (Fig. ) (about 2–4 times) (p = 0.021). Moreover, oogonia in the cultured material were slightly longer with respect to the material collected in nature (p = 0.035).
Figure (6–31). Diagnostic features of cultured material of Chara vulgaris Linn. in this study. Scale bar = 1 cm (Figs. ); scale bar = 100 μm (Figs. , , , ); scale bar = 200 μm (Figs. , ); Scale bar = 0.5 mm (Fig. ). (6, 7) Macroscopic habitus of Chara vulgaris thallus. (8) Part of thallus stem showing antheridia usually present at the newly formed branchlets at the stem apex (arrowhead), while oogonia are only still present on old branchlets (double arrowheads). (9–11) Whorls of branchlets usually curved inwards for the newly formed ones (arrowheads). (12–14) Nodes showing obtuse stipulodes in two closely placed rows (arrowheads). (15–18) Diplostichous, aulacanthous spine cells (arrowheads indicat spines present on the primary cortical rows, while double arrows depict larger secondary rows). (19) Elongated spine to the axis diameter in older stem. (20, 21) Terminal branchlet conical cells. (22) Fertile branchlet showing mature oogonia only and shedding antheridia. (23, 24) Fertile branchlet node with immature dark-green oogonium and solitary, spherical orange antheridium. (25, 26) Dark-black mature oogonia at branchlet node. (27, 28) Brown mature oospores with marked stripes. (29) Black mature oospore with pointed apex. (30–31) Internode cross-sections showing central siphon cells and cortical cells.
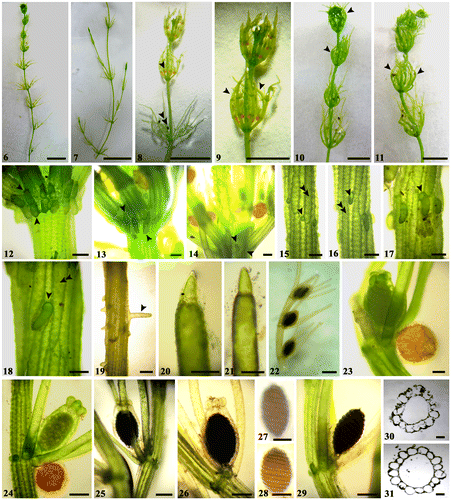
Phylogeny of the C. vulgaris population from the Springs of Moses
The cluster of Chara vulgaris was supported with a bootstrap value of 90% (Fig. ). In addition to our sample from the Springs of Moses, this cluster contained C. vulgaris samples from Greece, France, and Poland, as well as two Canadian samples obtained from GenBank (NC_008097 and DQ229107; see Table for a list of samples used in the phylogenetic analysis). The sequence of our sample was identical on the 546 positions of the matK gene with the samples from Poland and Canada, while the samples from Greece and France each had one different position. Other taxa were clearly differentiated from C. vulgaris with high bootstrap support (Fig. ).
Figure 32. Maximum likelihood tree determined on the basis of partial matK gene sequences of 22 Chara samples. Outgroup = Nitellopsis obtusa (AY170447). The specimen from this study is marked in bold. Bootstrap values above 50 are included. The scale bar indicates 5% sequence divergence.
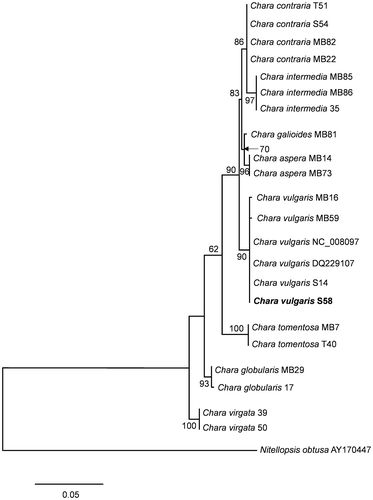
Table 3. List of Chara individuals used for the phylogenetic analysis. All samples were taken from herbarium collections. In addition, two sequences from GenBank were included in the phylogenetic analyses (see Fig. ).
Discussion
Chara vulgaris is common worldwide in all types of freshwater habitats, and occasionally in brackish waters (Blaženčić Citation2014; Caisová and Gąbka Citation2009; Krause Citation1997; Noedoost et al. Citation2015; Wood and Imahori Citation1965). Corillion and Guerlesquin (Citation1971) and Krause (Citation1997) reported that it occurred in Central Sahara, growing in wells of oases. This is in agreement with the present study. Caisová and Gąbka (Citation2009) mentioned in their recent monographic study that C. vulgaris usually prefers ambient-to-thermal habitats with low light intensity. Our results support this observation. Worldwide, C. vulgaris has been recorded from other ecologically similar spring habitats (Langangen Citation2008, Citation2016) and comparable neighbouring habitats in Israel (e.g. Negev Desert in Yehuda et al. Citation2013; the Mount Arbel National Park, Barinova and Romanov Citation2014). On the other hand, Hu et al. (Citation2009) recorded C. vulgaris in a cold spring in China.
In this study, two features of the freshly collected material were peculiar: (1) The specimens of the Sinai phenoecodeme were more slender and delicate than typical Chara vulgaris populations reported in the literature; and (2) antheridia were lost early. These traits were retained in the culture.
It is possible that the slender morphology of the Sinai C. vulgaris phenoecodeme is related to the isolated and small-sized habitat that it colonizes. Langangen (Citation2008) observed that spring-inhabiting C. vulgaris in Greece was very small and compact compared to typical C. vulgaris, and attributed this to the effect of the peculiar environment on C. vulgaris growth. Hu et al. (Citation2009) noted as well some environment-dependent morphological differentiations in C. vulgaris during their seasonal observations in a cold spring. Recent contributions by Calero, Colom, and Rodrigo (Citation2015) and Rojo et al. (Citation2015) have pointed out that phenology and biomass of charophytes and, particularly, C. vulgaris, might be directly affected by increased water-temperature regimes and climatic global warming. In general, phenotypic plasticity of charophytes caused by changes in light and salinity has been documented in detail by several authors (Wood and Imahori Citation1965; Küster, Schaible, and Schubert Citation2004; Schneider, Ziegler, and Melzer Citation2006; Schneider et al. Citation2015, Citation2016; Boszke and Bociąg Citation2008).
C. vulgaris specimens generally bear antheridia and oogonia together (Caisová and Gąbka Citation2009; Krause Citation1997; Wood and Imahori Citation1965). Morphological and life-cycle characteristics of Chara populations have been subject to little investigation in Egypt, although some studies on other aspects are available in the literature (El-Naggar Citation1995; Shaaban Citation1985; Zalat Citation1996). The fact that, in the Sinai specimens, antheridia are discarded before oogonia could be a mechanism limiting self-fertilization in this closed, isolated habitat. Holzinger and Pichrtová (Citation2016) reviewed self-protection of charophytes in their environments, and stated that Chara species have common stress-avoidance strategies that provide protection from multiple environmental stresses by reducing their reproductive structures. Early shedding of antheridia in the Springs of Moses can be considered as a kind of environmentally adapted mechanism.
In spite of the peculiar morphology and the isolated habitat, there were no major differences in the matK gene between our sample from the Springs of Moses and other samples of C. vulgaris worldwide. Overall, genetic divergence of the cosmopolitan species C. vulgaris was very low (Schneider et al. Citation2016). In many cases, putative cosmopolitan algal species colonizing habitats with contrasting characteristics turn out to be groups of species. Within the filamentous red alga Bangia atropurpurea, (Mertens ex Roth) C.Agardh, for instance, Müller et al. (Citation1998) showed that the freshwater populations formed a monophyletic clade, and Lynch, Sheath, and Müller (Citation2008) showed considerable genetic variation within an established Bangia morphospecies (cryptic diversity) even at a very low spatial scale. By contrast, however, Necchi et al. (Citation2013) recently demonstrated very low genetic diversity for a red alga (Compsopogon caeruleus) with cosmopolitan distribution in warm-climate freshwater habitats.
In summary, the overall morphology of the C. vulgaris sample from the Springs of Moses was different from “typical” Chara vulgaris populations. However, these differences were not reflected in the genome (at least not in the marker we studied). They may thus be interpreted as a phenotypic and physiological adaptation to the environment.
More Egyptian studies on Charales and on the effects of environmental conditions on their occurrence, morphology, and growth need to be implemented in the future to be able to suggest the suitable strategies to conserve and protect this neglected and not well investigated algal group in Egypt.
Notes on contributors
Abdullah Antar Saber collected Chara vulgaris specimens from Springs of Moses (Sinai, Egypt), cultivated the materials and followed their growth and life cycle, and wrote the draft manuscript.
Andreas Ballot performed the molecular analyses and contributed to editing the manuscript.
Susanne C. Schneider interpreted the results and contributed to editing the manuscript.
Marco Cantonati interpreted the results and edited the manuscript.
Disclosure statement
No potential conflict of interest was reported by the authors.
Funding
This work was supported by Norwegian Institute for Water Research (NIVA) through the strategic institute initiative “biodiversity”; the Autonomous Province of Trento and the Museo delle Scienze – MUSE, Limnology and Phycology Section, Trento, Italy.
Acknowledgements
Saber is very thankful to Prof. Shaaban A.S., The Botany Department, Faculty of Science, Ain Shams University, Cairo, Egypt, for his kind support on material sampling and cultivation. Marco Cantonati was partially funded by the Autonomous Province of Trento and the Museo delle Scienze, Limnology and Phycology Section, Trento, Italy. The phylogenetic analyses were financially supported by NIVA through the strategic institute initiative “biodiversity”.
References
- Abd El-Rahman, H. 2001. “Evaluation of Groundwater Resources in Lower Cretaceous Aquifer System in Sinai.” Water Resources Management 15 (3): 187–202.10.1023/A:1013021008462
- Andrews, M., S. McInroy, and J. A. Raven. 1984. “Culture of Chara hispida.” British Phycological Journal 19 (3): 277–280.10.1080/00071618400650291
- Azzella, M. M. 2014. “Italian Volcanic Lakes: A Diversity Hotspot and Refuge for European Charophytes.” Journal of Limnology 73 (3): 502–510.
- Ballot, A., M. Sandvik, T. Rundberget, C. J. Botha, and C. O. Miles. 2014. “Diversity of Cyanobacteria and Cyanotoxins in Hartbeespoort Dam. South Africa.” Marine and Freshwater Research 65 (2): 175–189.10.1071/MF13153
- Barinova, S., and R. Romanov. 2014. “Unique Locality with Charophytes in the Mount Arbel National Park, Israel.” Elixir Bio Diversity 77: 28932–28936.
- Barinova, S., and R. Romanov. 2015. “Charophyte Communities in the Ein Afeq Natural Reserve, Israel.” Natural Resources and Conservation 3 (2): 31–44.10.13189/nrc.2015.030202
- Blaženčić, J. 2014. “Overview of the Stoneworts (Charales) of Serbia with The Estimation of the Threat Status.” Botanica Serbica 38 (1): 121–130.
- Blindow, I. 1992. “Decline of Charophytes During Eutrophication: Comparison with Angiosperms.” Freshwater Biology 28 (1): 9–14.10.1111/fwb.1992.28.issue-1
- Bociag, K., A. Robionek, E. Rekowska, and K. Banas. 2013. “Effect of Hydrodynamic Disturbances on the Biomass and Architecture of the Freshwater Macroalga Chara globularis Thuill.” Acta Botanica Gallica 160 (2): 149–156.10.1080/12538078.2013.822826
- Boegle, M. G., S. Schneider, B. Mannschreck, and A. Melzer. 2007. “Differentiation of Chara intermedia and C. baltica compared to C. hispida Based on Morphology and Amplified Fragment Length Polymorphism.” Hydrobiologia 586 (1): 155–166.10.1007/s10750-006-0571-8
- Boszke, P., and K. Bociąg. 2008. “Morphological Variation of Oospores in The Population of Chara rudis A. Braun in a Mesotrophic Lake.” Polish Journal of Ecology 56 (1): 139–147.
- Braun, A. 1868. “Die Characeen Afrika’s [The Characeae of Africa].” Monatsbericht der Königlichen Preussischen Akademie der Wissenschaften zu Berlin 1867 (782–800): 873–944.
- Bravakos, P., G. Kotoulas, K. Skaraki, A. Pantazidou, and A. Economou-Amilli. 2016. “A Polyphasic Taxonomic Approach in Isolated Strains of Cyanobacteria from Thermal Springs of Greece.” Molecular Phylogenetics and Evolution 98: 147–160.10.1016/j.ympev.2016.02.009
- Caisová, L., and M. Gąbka. 2009. “Charophytes (Characeae, Charophyta) in the Czech Republic: Taxonomy, Autecology and Distribution.” Fottea 9 (1): 1–43.10.5507/fot.2009.001
- Calero, S., W. Colom, and M. A. Rodrigo. 2015. “The Phenology of Wetland Submerged Macrophytes Related to Environmental Factors.” Limnetica 34 (2): 425–438.
- Callahan, H. S., N. Dhanoolal, and M. C. Ungerer. 2005. “Plasticity Genes and Plasticity Costs: A New Approach using an Arabidopsis Recombinant Inbred Population.” New Phytologist 166 (1): 129–140.10.1111/j.1469-8137.2005.01368.x
- Cantonati, M., R. Gerecke, and E. Bertuzzi. 2006. “Springs of the Alps-Sensitive Ecosystems to Environmental Change: From Biodiversity Assessments to Long-Term Studies.” Hydrobiologia 562 (1): 59–96.10.1007/s10750-005-1806-9
- Cantonati, M., L. Füreder, R. Gerecke, I. Jüttner, and E. Cox. 2012. “Crenic Habitats, Hotspots for Freshwater Biodiversity Conservation: Toward An Understanding of Their Ecology.” Freshwater Science 31 (2): 463–480.10.1899/11-111.1
- Cantonati, M., J. Komárek, and G. Montejano. 2015. “Cyanobacteria in Ambient Springs.” Biodiversity and Conservation 24 (4): 865–888.10.1007/s10531-015-0884-x
- Cantonati, M., S. Segadelli, K. Ogata, H. Tran, D. Sander, R. Gerecke, E. Rott, M. Filippini, A. Gargini, and F. Celico. 2016. “A Global Review On Ambient Limestone-Precipitating Springs (LPS): Hydrogeological Setting, Ecology, and Conservation.” Science of the Total Environment 568: 624–637.10.1016/j.scitotenv.2016.02.105
- Corillion, R., and M. Guerlesquin. 1971. “Notes phytogéographiques sur les Charophycées d’Egypte. [Phytogeographical notes on Egypt Charophyceae].” Revue Algologique 10: 177–191.
- Corillion, R., and M. Guerlesquin. 1972. “Recherches sur les charophycées d’Afrique occidentale; Systématique, Phytogéographie et Ecologie, Cytologie [Research on the Charophytes of West Africa; Systematics, Phytogeography, Ecology and Cytology].” Travaux et Mémoires du laboratoire de Biologie végétale et Phytogéographie, fasc 25: 183.
- De Senerpont Domis, L. N., P. Fama, A. J. Bartlett, W. F. Prud’homme van Reine, and C. A. Espinosa. 2003. “Defining Taxon Boundaries in Members of The Morphologically and Genetically Plastic Genus Caulerpa (Caulerpales, Chlorophyta).” Journal of Phycology 39 (6): 1019–1037.10.1111/j.0022-3646.2003.02-203.x
- Doege, A., K. van de Weyer, R. Becker, H. Schubert. 2016. “Bioindikation mit Characeen.” [Bioindication of Characeae.] In Armleuchteralgen: Die Characeen Deutschlands [Charophyceae: The Characeae of Germany], edited by Arbeitsgruppe Characeen Deutschlands, 97–138. Berlin: Springer-Verlag.10.1007/978-3-662-47797-7
- El-Naggar, M. E. E. 1995. “Comparative Biochemical Studies on The Vegetative and Reproductive Stages of Chara vulgaris.” Journal of King Saud University-Science 7 (2): 191–204.
- Glazier, D. S. 2009. “Springs.” In Encyclopedia of Inland Waters. 1 vol, edited by G. E. Likens, 734–755. Amsterdam: Academic Press Elsevier.10.1016/B978-012370626-3.00259-3
- Holzinger, A., and M. Pichrtová. 2016. “Abiotic Stress Tolerance of Charophyte Green Algae: New Challenges for Omics Techniques.” Frontiers in Plant Science 7: 678. doi:10.3389/fpls.2016.00678.
- Hu, B., S. Xie, J. Feng, and M. Zhang. 2009. “Study on The Phenology of Chara vulgaris in Xin’an Spring, North China.” Frontiers of Biology in China 4 (2): 207–213.10.1007/s11515-009-0007-8
- Hume, W. E. 1906. The Topography and Geology of the Peninsula of Sinai (South Eastern Portion). Cairo, Egypt: Survey Department, 231–233.
- Kaiser, M. F., and S. Ahmed. 2013. “Optimal Thermal Water Locations Along the Gulf of Suez Coastal Zones, Egypt.” Renewable Energy 55: 374–379.10.1016/j.renene.2012.12.033
- Krause, W. 1997. “Charales (Charophyceae).” In Süsswasserflora von Mittleuropa. 18 vols, edited by H. Ettl, G. Gärtner, H. Heynig, and D. Mollenhauer, 202. Jena: Gustav Fischer Verlag.
- Küster, A., R. Schaible, and H. Schubert. 2004. “Light Acclimation of Photosynthesis in Three Charophyte Species.” Aquatic Botany 79 (2): 111–124.10.1016/j.aquabot.2004.01.010
- Lambert, E., D. Desmots, J. Le Bail, J.-B. Mouronval, and J.-C. Felzines. 2013. “Tolypella salina R. Cor. on the French Atlantic Coast: Biology and Ecology.” Acta Botanica Gallica 160 (2): 107–119.10.1080/12538078.2013.823105
- Langangen, A. 2008. “Charophytes from Some Aegean Islands (Khios, Lesvos and Limnos) in Greece.” Flora Mediterranea 18: 379–384.
- Langangen, A. 2016. Chara vulgaris L. (Charales) in Springs on Amorgos Island. (Greece). Botanica Serbica 40(2): 195–203.
- Lashin, A. 2013. “A Preliminary Study on the Potential of The Geothermal Resources Around the Gulf of Suez.” Egypt. Arab Journal of Geosciences 6 (8): 2807–2828.10.1007/s12517-012-0543-4
- Leliaert, F., H. Verbruggen, P. Vanormelingen, F. Steen, J. M. Lopez-Bautista, G. C. Zuccarello, and O. De Clerck. 2014. “DNA-Based Species Delimitation in Algae.” European Journal of Phycology 49 (2): 179–196.10.1080/09670262.2014.904524
- Logares, R., K. Rengefors, A. Kremp, K. Shalchian-Tabrizi, A. Boltovskoy, T. Tengs, A. Shurtleff, and D. Klaveness. 2007. “Phenotypically Different Microalgal Morphospecies with Identical Ribosomal DNA: A Case of Rapid Adaptive Evolution?” Microbial Ecology 53 (4): 549–561.10.1007/s00248-006-9088-y
- Lurling, M. 2003. “Phenotypic Plasticity in the Green Algae Desmodesmus and Scenedesmus with Special Reference to The Induction of Defensive Morphology.” Annales de Limnologie 39 (2): 85–101.10.1051/limn/2003014
- Lynch, M. D. J., R. G. Sheath, and K. M. Müller. 2008. “ISSR-Estimated Intraspecific Genetic Variation and The Phylogenetic Position of Bangia maxima (Bangiales, Rhodophyta).” Phycologia 47 (6): 599–613.10.2216/07-47.1
- McCourt, R. M., C. F. Delwiche, and K. G. Karol. 2004. “Charophyte Algae and Land Plant Origins.” Trends in Ecology and Evolution 19 (12): 661–666.10.1016/j.tree.2004.09.013
- Melzer, A. 1999. “Aquatic Macrophytes as Tools for Lake Management.” Hydrobiologia 395: 181–190.10.1023/A:1017001703033
- Merila, J., and A. Hendry. 2014. “Climate Change, Adaptation, and Phenotypic Plasticity: The Problem and The Evidence.” Evolutionary Applications 7 (1): 1–14.10.1111/eva.12137
- Morgan, P., K. Boulos, and C. A. Swanberg. 1983. “Regional Geothermal Exploration in Egypt.” Geophysical Prospecting 31 (2): 361–376.10.1111/gpr.1983.31.issue-2
- Müller, K. M., R. G. Sheath, M. L. Vis, T. J. Crease, and K. M. Cole. 1998. “Biogeography and Systematics of Bangia (Bangiales, Rhodophyta) Based on The Rubisco spacer, rbcL gene and 18S rRNA Gene Sequences and Morphometric Analyses. 1. North America.” Phycologia 37 (3): 195–207.10.2216/i0031-8884-37-3-195.1
- Necchi Jr, O., A. S. F. Garcia, E. D. Salomaki, J. A. West, M. Aboal, and M. L. Vis. 2013. “Global Sampling Reveals Low Genetic Diversity Within Compsopogon (Compsopogonales, Rhodophyta).” European Journal of Phycology 48 (2): 152–162.10.1080/09670262.2013.783626
- Noedoost, F., H. Riahi, M. Sheidai, and A. Ahmadi. 2015. “Distribution of Charophytes from Iran with Three New Records of Characeae (Charales, Chlorophyta).” Cryptogamie, Algologie 36 (4): 1–17.
- Nowicka-Krawczyk, P., and J. Żelazna-Wieczorek. 2016. “Dynamics in Cyanobacterial Communities from A Relatively Stable Environment in an Urbanised Area (Ambient Springs in Central Poland).” Science of the Total Environment 579: 420–429.
- Palacio-López, K., B. Beckage, S. Scheiner, and J. Molofsky. 2015. “The Ubiquity of Phenotypic Plasticity in Plants: A Synthesis.” Ecology and Evolution 5 (16): 3389–3400.10.1002/ece3.2015.5.issue-16
- Rojo, C., C. Martínez-Ruiz, M. Carramiñana, and M. A. Rodrigo. 2015. “Foreseeable Global Warming Will Differentially Affect Chara vulgaris Populations from Different Altitudes.” Aquatic Botany 122: 20–26.10.1016/j.aquabot.2014.12.002
- Rosati, M., M. Cantonati, R. Primicerio, and G. Rossetti. 2014. “Biogeography and Relevant Ecological Drivers in Spring Habitats: A Review on Ostracods of the Western Palearctic.” International Review of Hydrobiology 99 (6): 409–424.10.1002/iroh.v99.6
- Saber, A. A., K. Ichihara, and M. Cantonati. 2016. “Molecular Phylogeny and Detailed Morphological Analysis of Two Freshwater Rhizoclonium Strains from Contrasting Spring Types in Egypt and Italy.” Plant Biosystems. doi:10.1080/11263504.2016.1211195.
- Saber, A. A., M. Cantonati, M. L. Vis, A. Anesi, and G. Guella. 2016. “Multifaceted Characterization of a Lemanea fluviatilis Population (Batrachospermales, Rhodophyta) from A Glacial Stream in The South–Eastern Alps.” Fottea 16 (2): 234–243.10.5507/fot.2016.014
- Sakayama, H., Y. Hara, and H. Nozaki. 2004. “Taxonomic Re-Examination of Six Species of Nitella (Charales, Charophyceae) from Asia, and Phylogenetic Relationships within the Genus Based on rbcL and atpB Gene Sequences.” Phycologia 43 (1): 91–104.10.2216/i0031-8884-43-1-91.1
- Sakayama, H., F. Kasai, H. Nozaki, M. M. Watanabe, M. Kawachi, M. Shigyo, J. Nishihiro, I. Washitani, L. Krienitz, and M. Ito. 2009. “Taxonomic Reexamination of Chara globularis (Charales, Charophyceae) from Japan Based on Oospore Morphology and rbcL Gene Sequences, and the Description of C. leptospora sp. nov.” Journal of Phycology 45 (4): 917–927.10.1111/j.1529-8817.2009.00700.x
- Sanjuan, J., A. Vincente, N. Flor-arnau, T. Monleón, J. Cambra, and C. Martín-Closas. 2017. “Effects of Light and Temperature on Chara vulgaris (Charophyceae) Gyrogonite Productivity and Polymorphism – Palaeoenvironmental Implications.” Phycologia 56 (2): 204–212.10.2216/15-140.1
- Sato, M., H. Sakayama, M. Sato, M. Ito, and H. Sekimoto. 2014. “Characterization of Sexual Reproductive Processes in Chara braunii (Charales, Charophyceae).” Phycological Research 62 (3): 214–221.10.1111/pre.2014.62.issue-3
- Schneider, S., C. Ziegler, and A. Melzer. 2006. “Growth Towards Light as an Adaptation to High Light Conditions in Chara Branches.” New Phytologist 172 (1): 83–91.10.1111/nph.2006.172.issue-1
- Schneider, S. C., A. García, C. Martín-Closas, and A. R. Chivas. 2015. The Role of Charophytes (Charales) in Past and Present Environments: An Overview. Aquatic Botany 120 (A): 2–6.10.1016/j.aquabot.2014.10.001
- Schneider, S. C., D. E. Pichler, T. Andersen, and A. Melzer. 2015. “Light Acclimatation in Submerged Macrophytes: The Roles of Plant Elongation, Pigmentation and Branch Orientation Differ among Chara Species.” Aquatic Botany 120(A): 121–128.10.1016/j.aquabot.2014.05.002
- Schneider, S. C., A. Rodrigues, T. F. Moe, and A. Ballot. 2015. “DNA Barcoding the Genus Chara: Molecular Evidence Recovers Fewer Taxa Than The Classical Morphological Approach.” Journal of Phycology 51 (2): 367–380.10.1111/jpy.12282
- Schneider, S. C., P. Nowak, U. Ammon, and A. Ballot. 2016. “Species Differentiation in the Genus Chara (Charophyceae): Considerable Phenotypic Plasticity Occurs Within Homogenous Genetic Groups.” European Journal of Phycology 51 (3): 282–293.10.1080/09670262.2016.1147085
- Shaaban, A. S. 1985. “The Algal Flora of Egyptian oases. II- On the Algae of Siwa Oasis.” Proceedings of Egyptian Botanical Society 4: 1–10.
- Shaaban, A. S. 1994. “Freshwater Algae of Egypt. UN Environmental Program, National Biodiversity Unit.” Biological Diversity of Egypt. GF/6105-92-02-2205: 150.
- Shaaban, A. S., H. A. Mansour, and A. A. Saber. 2015. “Unveiling Algal Biodiversity of El-Farafra Oasis (Western Desert, Egypt) and Potential Relevance of Its Use in Water Bio-Assessment: Special Interest on Springs and Drilled Wells.” Egyptian Journal of Phycology 16: 47–75.
- Sturchio, N. C., and G. B. Arehart. 1996. “Composition and Origin of Thermal Waters in the Gulf of Suez area, Egypt.” Applied Geochemistry 2: 471–479.10.1016/S0883-2927(96)00025-X
- Tamura, K., G. Stecher, D. Peterson, A. Filipski, and S. Kumar. 2013. “MEGA6: Molecular Evolutionary Genetics Analysis Version 6.0.” Molecular Biology and Evolution 30 (12): 2725–2729.10.1093/molbev/mst197
- Timme, R. E., T. R. Bachvaroff, and C. F. Delwiche. 2012. “Broad Phylogenomic Sampling and The Sister Lineage of Land Plants.” PLoS ONE 7: Article Number e29696.
- Urbaniak, J. 2010. “Analysis of Morphological Characters of Chara baltica, C. hispida, C. horrida and C. rudis from Europe.” Plant Systematics and Evolution 286 (3): 209–221.10.1007/s00606-010-0301-6
- Urbaniak, J., and M. Combik. 2013. “Genetic and Morphological Data Fail to Differentiate Chara intermedia from C. baltica, or C. polyacantha and C. rudis from C. hispida.” European Journal of Phycology 48 (3): 253–259.10.1080/09670262.2013.805249
- Verbruggen, H. 2014. “Morphological complexity, Plasticity, and Species Diagnosability in The Application of Old Species Names in DNA-based Taxonomies.” Journal of Phycology 50 (1): 26–31.10.1111/jpy.2014.50.issue-1
- Von Hustedt, F. 1949. “Diatomeen von der Sinai-Halbinsel und aus dem Libanon-Gebiet.” [Diatoms from the Sinai Peninsula and from the Lebanon Area.] Hydrobiologia 2 (1): 24–55.10.1007/BF00117694
- Wood, R. D., and K. Imahori. 1965. A Revision of the Characeae. In First Part: Monograph of the Characeae. Weinheim: J. Cramer Verlag, 904.
- Yehuda, G., S. Barinova, T. Krugman, T. Pavlicek, Y. Nov, and E. Nevo. 2013. “Microscale Adaptive Response of Charophytes of the Negev Desert, Israel: Species Divergences by AFLP.” Natural Resources and Conservation 1 (3): 55–64.
- Zahran, M. A., and A. J. Willis. 2009. “The Sinai Peninsula.” In The Vegetation of Egypt. 2nd ed., edited by M. A. Zahran, 213–249. Dordrecht: Springer Science+Business Media B.V. 10.1007/978-1-4020-8756-1
- Zalat, A. A. 1996. “Charophyte Gyrogonites from Holocene Lacustrine Sediments of the Fayoum Depression, Egypt.” Neues Jahrbuch für Geologie und Paläontologie H (8): 502–516.