Abstract
Seed dispersal is a key process that humans can influence in various ways. However, this type of interaction is less studied than dispersal by other animals. In addition, tracking seeds to determine dispersal pattern is a complicated task. We explored the effects of mowing machinery on seed dispersal in roadsides by testing two methods, (i) by marking fruits and diaspore of plants within the standing vegetation (ii) by making fake plants containing estimated quantities of marked seeds. We measured the pattern of dispersal by considering each seed and the centre of seed shadows of each species calculated by two means (i.e. the centroids and the gravity centres). We estimated the distance of dispersal from roadsides, and the deviation angle from the direction of the mowing. We then analysed the variation in dispersal patterns among species, seed mass and width/length ratio by using correlation tests. Seeds coloured within the standing vegetation were detected from 0.5 to 15.51 metres away from roadsides, with deviation angles from the mowing machinery ranging from –153.43° to 161.57°. With the other method we found a mean distance of dispersal ranging from 0.5 to 13.01 metres and deviation angle from –168.69° to 180°. We did not find strong variation in dispersal patterns among species and seed traits, but we demonstrated however that mowing machinery influenced both dispersal distance and deviation angles.
Introduction
Understanding plant dispersal is crucial for addressing questions about population and community dynamics (Levine and Murrell Citation2003). Dispersal is known as the process of a propagule moving through space and time from separation from the parent plant to a landing point. Propagule release from the parent plant can be passive, involving gravity – active, for instance, with structural adaptations allowing propulsion – or can be ensured by diverse biotic agents (vertebrates and invertebrates) and abiotic agents such as wind, water, desiccation, or human-related vectors. Within anthropochory, which is the dispersal of plant propagules by humans, vectors can be diverse and efficient in dispersing seeds far away from the parent plants (Petit et al. Citation2013; Pickering and Mount Citation2010; Pickering et al. Citation2011). For example, the most known and studied vectors are clothing (Pickering et al. Citation2011); footwear, which can transport seeds over 5 km (Clifford Citation1956; Ware et al. Citation2012); bikes, where seeds can be stuck on tires and then easily transported 50 to 500 metres away (Weiss, Brummer, and Pufal Citation2016); and cars (Von der Lippe et al. Citation2013; Zwaenepoel, Roovers, and Hermy Citation2006), which are able to transport seeds 250 km away from the initial site (Taylor et al. Citation2012). In agricultural landscapes, motor vehicles such as cars, mowers and harvesters can promote seed dispersal along roads (Auffret and Cousins Citation2013), from field to field (Strykstra, Verweij, and Bakker Citation1997) and within fields (Bischoff Citation2005; Rew, Froud-Williams, and Boatman Citation1996). In addition, it has been shown that mowers used for road verge management can transport seeds along roads (Vitalos and Karrer Citation2009). Each of these vectors influences, directly or indirectly, the spatial pattern of seed dispersal, which is represented by the different quantities of the landing seeds and propagules scattered away from the parent plants at different locations. This pattern depends on the interactions between the propagules or seed characteristics and the vectors. The analysis of seed traits has shown that seed mass is inversely correlated to dispersal distance, the lightest being dispersed farther (Tackenberg, Poschlod, and Bonn Citation2003). However, Thomson et al. (Citation2011) found that seed dispersal distances were principally determined by the height of the plants, rather than by seed mass. To our knowledge, the interactions between mowing and seed traits have not been considered, especially regarding mowing machinery and its effects on seed dispersal pattern.
Several methods are commonly used to measure spatial dispersal of seeds, whether directly – i.e. by trapping or tracking – or indirectly through genetic analyses (Bullock, Shea, and Skarpaas Citation2006; Nathan et al. Citation2003). Trapping methods allow quantification of propagules deposited at a particular location, irrespective of the dispersal vector (Bullock, Shea, and Skarpaas Citation2006), while tracking is generally specific to a particular vector, such as a bird species (Pons and Pausas Citation2007) or a vehicle (Taylor et al. Citation2012). Tracking propagules implies that dispersers or propagules are followed, potentially in real time (Skarpaas et al. Citation2004), from a source point to a final point where conditions for germination are appropriate. Methods for seed tracking use different seed tagging techniques, such as magnet locators (Alverson and Diaz Citation1989), radioactive marking (Vander Wall, Borchert, and Gworek Citation2006), wire tin-tagging and thread-marking (Xiao, Jansen, and Zhang Citation2006) and colour marking (Aerts et al. Citation2006; Isselin-Nondedeu, Rey, and Bédécarrats Citation2006; Lemke, von der Lippe, and Kowarik Citation2009).
In this study, we measured the effects of road verge mowing on the dispersal of seeds and addressed the following questions: (1) what are the spatial patterns of seed dispersal under the influence of mowing? and (2) do seed dispersal patterns differ among species and their related seed traits? To answer these questions, we developed and tested two simple techniques for tracking seeds; one by marking the propagules of individuals belonging to several species from the standing vegetation, and the other by constructing fake plants containing an estimated quantity of marked seeds. After the mowing, seeds were tracked in the road verge and adjacent arable field by collecting seeds from seed traps. For each species and both methods, we measured the deviation angle from the mowing machinery and the dispersal distance from the road verge. We tested whether mowing can influence the dispersal distance from road verges to field margins, and whether light seeds are dispersed further than heavy ones.
Material and Methods
Study site
The study was conducted in central-western France, (Indre-et-Loire department). We realized the experiment on one road verge (47°05′ N, 1°10′ E) close to an arable field sown with winter wheat. The study site was chosen according to the mowing planning of the road service, and to farmer agreement. The zone between the road pavement and the ditch was 2 metres wide (0.5 m for the safety zone and 1.5 m for the berm), with a ditch of 0.7 m depth; the total width of the road verge was 3.4 m (Figure ). The distance between the end of the berm and the crop edge was 1.4 metres (Figure ). This type of road verge is representative of many road verges found in agricultural and semi-natural landscapes. Annual rainfall in the study site is 695.6 mm, minimum mean temperature is 1.9 °C, in February, and maximum mean temperature is 25.5 °C, in July (MétéoFrance, Citation2017). The mowing was done on 27 June, from 1300 to 2100. This date corresponded to the mowing period fixed by the road service. During this period, rainfall was null, mean temperature was 21.98 °C (± 0.89 SD) and mean wind ranged from 2 to 10 km.h−1 (Infoclimat database, Citation2017).
Figure 1. Sketch of seed trapping experiment. (a) Top view of the site, including the three blocks and the transects crossing the road verge and the first metres in the arable field, and (b) Position of sticky traps along one transect crossing the berm, ditch, embankment, crop edge and field margin.
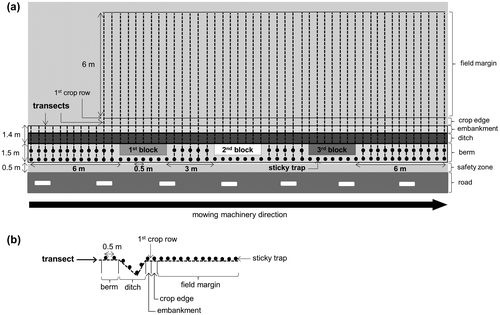
Experimental design
Hereafter, for convenience, we will use the word “seed” instead of the real unit “diaspore”. In order to measure the spatial patterns of seeds we combined two methods, both implemented on the same road verge.
Method 1: fake plants with coloured seeds
Most of the methods for studying plant dispersal pattern used tagging or painting on the entire fruits or the whole ear (for Poaceae species), and the seeds were therefore not marked. In this study we wanted to investigate the seed dispersal pattern under mowing, and for this purpose we designed fake plants that can be “planted” within the standing vegetation and mown. In addition, we filled their fake fruits with a known quantity of coloured seeds.
We selected seeds of three species, based on differences in form and length of the seed and their availability in large quantity in the market. The species were: Triticum aestivum L. (elongated seed, on average 7 mm length × 3 mm width, and 2.80 mg dry mass), Festuca rubra L. (elongated seed, 6 mm length × 1 mm width, and 0.44 mg dry mass) and Panicum miliaceum L. (spherical seed, 3 mm length × 2.6 mm width, and 3.28 mg dry mass). Sixty samples of 2 or 1 g of seeds for each species were prepared, i.e. 2 g of T. aestivum (45 seeds on average), and 1 g of F. rubra and P. miliaceum (110 and 450 seeds on average, respectively). For each species, the 60 samples were divided into six groups and coloured with fluorescent and shiny paint (i.e. 18 colours, Supplementary material I) applied with a spray gun of 0.3 mm needle size (Von der Lippe et al. Citation2013).
Each seed sample was then enclosed in fake fruits made of fabric of density 11 ± 1 g.m−2, which were enclosed and stapled at the base of a paper cone (9 cm height, 5 cm radius, Supplementary material II a). Each cone was then fixed with adhesive tape on the top of one reed stem, the overall forming a “fake plant” (Supplementary material II a). We made 60 fake plants for each of the three species, and tested two types: a small-sized type of 15 cm height, and a tall-sized type of 60 cm height (Supplementary material II b). Thirty fake plants of each size were thus tested with each of the three types of seeds.
To take into account possible changes in the speed of the mowing machinery along the road, the fake plants were planted in three blocks. Each block measured 3 × 0.5 m and was placed 3 m apart along the berm (i.e. the area between the safety zone and the ditch, Figure a). Within each block, 20 fake plants of each species were planted at random into the soil (i.e. 10 small fake plants of one colour and 10 tall fake plants of another colour, Supplementary material II b, colour code is given in Supplementary material I). Colours within each block were chosen in order to avoid confusion among species, between fake plants and between blocks (two colours for each species per block, six colours per block, 18 colours in total, Supplementary material I).
Method 2: marking of seeds
For this method, we applied the colouring directly to mature fruits and dispersal structures of species growing in the berm. Colouring was conducted on 27 June just prior to the start of mowing. Depending on the number of individuals per species, we coloured 20 and 50 individuals for frequent species and abundant species, respectively, and about 10 individuals for rare species. Within the three blocks, a total of 1040 individuals were marked corresponding to 20 species, including 16 species in block 1, 12 in block 2 and 14 in block 3 (Supplementary material III).
Three different colours were used (i.e. one colour per block, Supplementary material I) and the seeds of each individual were marked with a spray gun of 0.3 mm needle size (Von der Lippe et al. Citation2013). Two sides of the seeds were coloured at a distance of 10 cm from the individuals to avoid colouring diaspores outside the block. In order to examine possible links between seed traits and dispersal pattern under mowing, we collected a minimum of 20 seeds in the neighbouring vegetation for species that were in at least two blocks. We measured two main seed traits: dry mass (in mg) and width/length ratio, corresponding to the shortest length divided by the longest length of the seed (Table ).
Table 1. Mean dry mass and morphological characteristics for most abundant seeds coloured within the standing vegetation. The total number of seeds trapped for each species during the experiment, as well as the total number of individuals of mature species growing within blocks, are specified.
Mowing
Twenty-seven metres of the berm were mown by mowing machinery usually used by local state agents (Thénor 560 PL, 70 horsepower). The mowing machinery was attached to a 90-horsepower tractor (CLAAS-ARION 410). The rotor was fitted with 120 flails, which had a rotational speed of 3000 rpm. Flail mowers are generally used to manage road verges in European agricultural landscapes. The cutting width was 1600 mm and the height of the stubble after cutting was 7 cm, while speed was around 5 km per hour. The mowing started 6 m before block 1 and stopped 6 m after block 3 (Figure a).
Seed sampling
Patterns of seed dispersal were investigated along 55 transects perpendicular to the road, and regularly placed every 0.5 m (Figure a). Transects positioned 6 m before the first block crossed the berm, the ditch and the embankment, while all other transects stretched 6 m into the field margin (Figure a). No transects into the field margin were set up before block 1 because our first tests highlighted that the machinery dispersed only a small quantity of plant material in the opposite direction of the mowing, and because of time constraints.
Along each transect, sticky traps were positioned regularly, every 0.5 m, except within experimental blocks (Figure a). The traps were made with 140 mm diameter plastic plates, each containing a paper filter coated with grease and fixed to the soil with skewer metallic pegs. Two traps were placed within the berm, four traps within the ditch, one trap within the embankment, one trap within the crop edge and 12 traps within the field margin (Figure b). A total of 949 traps were placed on the ground the day prior to mowing, and were collected just after the mowing. All coloured seeds were then identified to the species level and counted in the laboratory under a dissecting microscope.
Analysis of spatial pattern
For seeds coloured within the standing vegetation, we only retained species for which at least 35 seeds were trapped, and those for which their parent plants occurred in at least two blocks, in order to obtain sufficient statistical power. A total of nine species were analysed (Table and Supplementary material III).
Measurements of spatial dispersal pattern
We first computed two synthetic indexes that quantify the spatial pattern of the dispersed seeds: the centroid and the gravity centre (Supplementary material IV). We calculated these indexes for each species of the standing vegetation and for fake plants, as well as for each block, using the R software (R Development Core Team Citation2013) and the SDMTools package (VanDerWal et al. Citation2014). The centroid is the mean value of the x and y coordinates of the trapped seeds (Bischoff Citation2005), while the gravity centre was obtained by weighting the x and y coordinates by the number of seeds in each trap. The centroid allowed us to locate the centre of dispersal based only on the presence of seeds, while the gravity centre allowed us to locate the centre of dispersal weighted by the number of seeds caught in each trap.
First, we estimated the distance of dispersal of each species by calculating the length of the vector joining the centre of each block and the centroid or the gravity centre (Supplementary material IV). We also calculated the deviation angle between the mowing direction and each vector of dispersal (Supplementary material IV).
Second, we estimated the distance of dispersal and the deviation angle for each seed of each species by considering the x and y coordinates of every trap in which seeds were found. These metrics were obtained using Pythagoras.
Variation of the spatial pattern among species, blocks and seed traits
For this part, we relied only on the distance of dispersal and the deviation angle computed for each seed of each species.
To measure the variation in the spatial pattern of dispersal among species, we calculated the correlation report (hereinafter “cr”) between the quantitative variable “dispersal distance” and the qualitative variable “species name” (Maumy-Bertrand and Bertrand Citation2010). The p-value estimation was based on the chi-squared test. This procedure was also chosen to measure the variation in spatial pattern of dispersal of species among blocks and among heights of fake plants.
To measure the variation in spatial pattern of dispersal among seed traits, we considered the seed dry mass and the smallest/highest length ratio varying from 1, when both lengths are equal (spherical seed), to less than 1 (elongated seed). We then used the Kendall rank correlation (hereinafter “Tau”) between each quantitative trait and the dispersal distance. The p-value estimation was based on the z test.
All these analyses were performed using the BioStatR package (Bertrand and Bertrand Citation2012).
Results
For seeds coloured within the standing vegetation, a total of 5132 seeds were trapped. The nine most abundant species, which were therefore retained for the analyses, contributed to 92% of all seeds trapped (4708) and the number of seeds of Schedonorus arundinaceus (Schreb.) Dumort. was the highest (2440 seeds, 52%, Table ). For fake plants, 400 seeds were trapped, including 349 seeds of Festuca rubra, 46 of Triticum aestivum and 5 of Panicum miliaceum.
Variation in the spatial pattern among species and blocks
Considering seeds coloured within the standing vegetation, all seeds were trapped within road verges and no particular variation among species was observed for either dispersal distance and deviation angle (cr for each seed = 0.02 and 0.07, respectively, both p < 0.001, Figure and ). Based on each seed of each species, centroid and gravity centre, we found that the majority of seeds of all species were trapped between 0.5 m and 5 m from berms (97% of all seeds, Figure and Supplementary material V). Considering each seed, we observed that seeds of four species were trapped between 10.50 m and 15.51 m from berms, i.e. Arrhenatherum elatius (L.) P.Beauv.ex J.Presl & C.Presl, Dactylis glomerata L., Holcus lanatus L., and S. arundinacea (Figure ).
Figure 2. Variation of seed dispersal distances among the nine most abundant species within the standing vegetation and the three blocks considering all coloured seeds found in the traps. The names of the species are abbreviated by keeping the first three letters of the family name and the first two letters of the gender name. The distances of dispersal from berms are in metres, n = 4708.
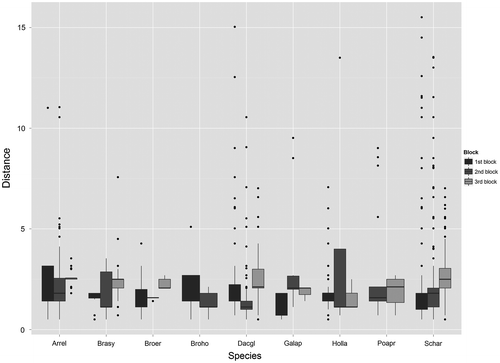
Figure 3. Variation of deviation angles among the nine most abundant species and the three blocks considering all coloured seeds found in the traps. The names of the species are abbreviated by keeping the first three letters of the family name and the first two letters of the gender name. Deviation angles close to zero correspond to seeds found parallel to the road verge, <0 seeds found on the road, >0 projected in the direction of the field. The deviation angles from the mowing direction are in degree, n = 4708.
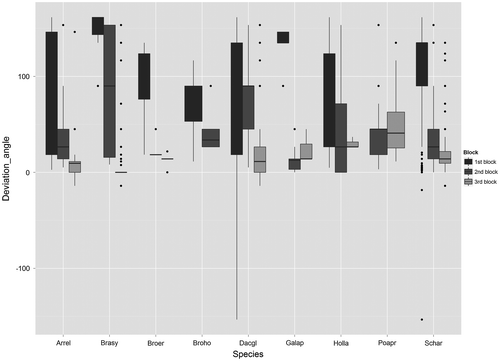
Based on each seed, centroid and gravity centre, almost all seeds of all species had a deviation angle higher than 10° from road verges (87% of all seeds, Figure , Supplementary material V). Globally, deviation angles were higher for block 1 than for blocks 2 and 3 (cr for each seed = 0.31, p < 0.001, Figure and Supplementary material V), while a slight variation was observed for dispersal distances (cr for each seed = 0.07, p < 0.001, Figure and Supplementary material V). Globally, we observed relatively higher deviation angles for block 1 than for blocks 2 and 3. Considering each seed, some seeds of A. elatius, Brachypodium sylvaticum (Huds.) P.Beauv, D. glomerata, H. lanatus, and S. arundinacea had a deviation angle of 162°, while a few seeds of D. glomerata, and S. arundinaceus had a deviation angle of –153° (Figure ).
For the fake plants, all seeds were trapped within road verges and slightly significant variation among species and heights was observed for both distances of dispersal and deviation angles (both cr for each seed = 0.01 and 0.05 for distances of dispersal and deviation angles, respectively; both p < 0.001, Figure and ). Based on each seed, centroid and gravity centre, we found that the majority of seeds were trapped between 0.5 m and 10 m from berms for all species (97% of all seeds, Figure and Supplementary material VI). Considering each seed, two seeds of tall fake plants of Triticum aestivum were found at 13 m from berms (Figure ).
Figure 4. Variation of seed dispersal distances among the three species and the three blocks for all coloured seeds of the fake plants. The names of the species are abbreviated by keeping the first three letters of the family name and the first two letters of the gender name. The distances of dispersal from berms are in metres, n = 400. For species height: H1 = 15 cm, H2 = 60 cm.
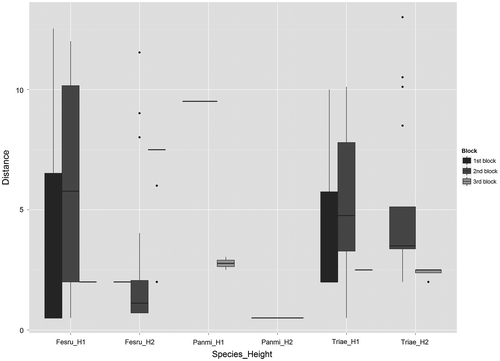
Figure 5. Variation of deviation angles among the three species and the three blocks for all coloured seeds of the fake plants. The names of the species are abbreviated by keeping the first three letters of the family name and the first two letters of the gender name. Deviation angles close to zero correspond to seeds found parallel to the road verge, <0 seeds found on the road, >0 projected in the direction of the field. The deviation angles from the mowing direction are in degrees, n = 400. For species height: H1 = 15 cm, H2 = 60 cm.
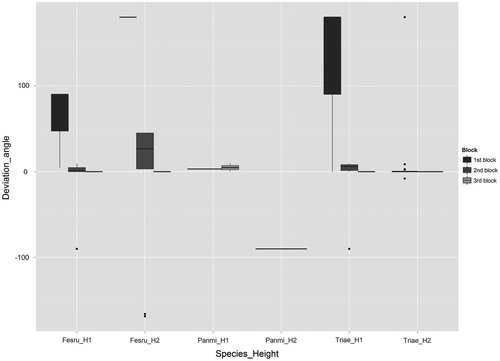
Based on each seed, centroid and gravity centre, a relatively high amount of seeds of all species had a deviation angle of 0° (59% of all seeds, Figure and Supplementary material VI). Overall, the main difference between blocks also concerned deviation angles (cr for each seed = 0.85, p < 0.001, Figure and Supplementary material VI), while a slight variation was observed for distances of dispersal (cr = 0.48, p < 0.001, Figure and Supplementary material VI). Similarly, deviation angles were higher for block 1 than for blocks 2 and 3 (Figure and Supplementary material VI). Considering each seed, some seeds of tall fake plants of Festuca rubra and of short fake plants of T. aestivum had a deviation angle of 180°, while a few seeds of tall fake plants of Festuca rubra had a deviation angle of –166 or –169° (Figure ).
Variation of spatial patterns among seed traits
Considering seeds coloured within the standing vegetation, slightly significant correlations were observed between distances of dispersal and seed mass or smallest/highest length ratio (Tau = 0.10 and –0.08, respectively, both p < 0.001, Figure ). We observed that the majority of seeds weighing more than 3 mg had a distance of dispersal lower than 5 m, while species weighing less than 1 mg had a distance of dispersal ranging from 0.5 m to 15.51 m (Figure ). This latter pattern of dispersal was also observed for seeds characterized by the smallest/highest length ratio, ranging from 0.15 to 0.20 (Figure ).
Figure 6. Relationship between dispersal distance, seed mass and width/length ratio for all coloured seeds of the standing vegetation. The x-axis corresponds to the distance of dispersal, the y-axis to the width/length ratio of seeds and the z-axis to the seed dry mass. n = 4708.
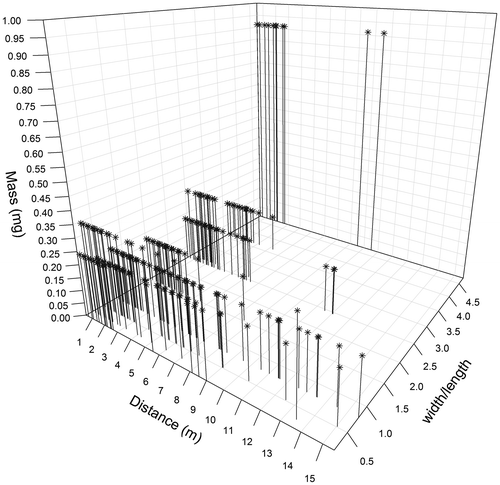
For the fake plants, in the same way, slightly significant correlations were observed between distances of dispersal and seed mass or smallest/highest length ratio (both Tau = –0.02 and p < 0.001, Figure ). In addition, no particular patterns of dispersal were observed (Figure ).
Discussion
In this work we investigated the understudied effects of mowing machinery on the dispersal of seeds along roadside vegetation and from roadside to adjacent arable fields. We tested these effects by setting up two original methods for tracking seeds from the mown parent plants to the landing sites. Our results showed that mowing had a slight influence on the dispersal and seed shadow of several species growing on roadsides. Although the direction of mowing was parallel to the road and the mower was protected, both our methods allowed us to track seeds and detect seeds from 0.5 to 13 metres away from the roadsides where seeds of the species were marked, with quite high deviation angles ranging from –153° to 162°.
These results support previous studies that have highlighted the influence of several anthropogenic disturbances associated with roads on seed dispersal patterns. These disturbances are in particular the increase of longitudinal and lateral movements of seeds due to airflow of vehicles (Von der Lippe et al. Citation2013), the transport of seeds through mud attached to vehicles (Zwaenepoel, Roovers, and Hermy Citation2006), and their transport by mowers (Vitalos and Karrer Citation2009). While it has been shown that different parts of a mowing machine can transport seeds (Strykstra, Verweij, and Bakker Citation1997), in this study we found that the machine can also project seeds in the direction of adjacent fields, and play a role in short-distance dispersal of seeds.
For the seeds coloured within the standing vegetation, the number of seeds found after 20 min. of mowing was higher (5132) than the number found in our previous study (Chaudron, Chauvel, and Isselin-Nondedeu Citation2016), in which 2224 and 2715 seeds, respectively, were found after three months, with and without one mowing. This previous study had been conducted in close sites with similar plant communities, although with no seed tracking.
As in our previous study, we also observed that the majority of species found in the seed traps after mowing belonged to the Poaceae family. Indeed, grasses were abundant in the standing vegetation and many grass species produce seeds abundantly; therefore, the probability of having more seeds in the traps is higher than for less abundant species. However, it is impossible to evaluate the number of seeds produced in the standing vegetation, and the number may be or may be not correlated to the number of individuals of a species within the standing vegetation. This is why we developed the method of tracking seeds by using fake plants. Compared to our experiment, relatively short dispersal distances were observed for several Poaceae species under a controlled environment and without any mechanical disturbance (Hensen and Müller Citation1997; Rabinowitz and Rapp Citation1981). In such studies, the dispersal ability of grass species was estimated by releasing seeds from heights of 1 to 4.2 m, with or without simulated winds, and resulting in maximum dispersal distances comprising between 0.11 to 0.46 m, and 0.22 to 2.50 m, respectively. Under the influence of mowing, we generally observed longer dispersal distances that ranged between 0.5 and 15.51 m. Even if painting seeds increased their weight and their terminal velocity (Von der Lippe et al. Citation2013), our results support the fact that the mowing machine indeed modified the distance of dispersal of these seeds, either because of the projecting effect of the rotating blades, or the related blowing air stream. This latter factor was experimentally tested by Hensen and Müller (Citation1997). We observed that seeds of Arrhenatherum elatius, Dactylis glomerata, Holcus lanatus and Schedonorus arundinaceus can have a relatively long dispersal distance. For D. glomerata, H. lanatus and S. arundinaceus, this could be related to their low weight, as previously suggested (Greene and Johnson Citation1993; Venable and Brown Citation1988). In addition, the lightest and most elongated seeds were found at both short and long distances, whereas the heaviest were mostly found at short distances. This suggests that there may be an interactive effect between mechanical disturbances and seed traits on the dispersal pattern.
Despite the protection provided by the deck assembly (which encloses the blades), we found that the mowing machine could influence the deviation angle from the mowing direction, which was particularly observed for the experiment with seeds coloured within the standing vegetation, revealing a dispersal in the direction of the field margin (87% of deviation angles were higher than 10° from road verges). Nevertheless, these preferential directions of dispersal could have been increased by a potential sticky effect of the paint during the colouring of seeds in the relatively dense vegetation. In addition, we observed relatively higher deviation angles for seeds of block 1 than for blocks 2 and 3. One explanation could be that even if the mowing machine started to cut 6 m before block 1 and controlled the cutting height, the blades may have been a little higher in the first block than in the others, projecting the seeds through a larger angle.
With the fake plants, a low quantity of seeds was trapped in the sampled area and no seeds were observed on the paved road area. This could be explained either by dispersal having occurred mainly on the opposite road verge, or, more likely, seeds having been released in too low quantities, potentially due to the exaggerated resistance of the fabric used to design the fake fruits and/or to a high resistance of the staples. A very low quantity of seeds of Panicum miliaceum was trapped while several fruits were found during identification, suggesting that their coloured coat was destroyed by the mowing machinery. Fifty-nine per cent of all seeds were dispersed with a null deviation angle from road verges, revealing a preferential dispersal in the direction of the mowing. In comparison with real plants, the resistance of the adhesive tape used to stick the fake fruits onto the stem, and the ease with which the fake plants could be dug out of the soil, may have facilitated transport of the fake plants by the mowing machine. Indeed, mowers can accumulate and transport a large quantity of plant material (Strykstra, Verweij, and Bakker Citation1997), and in our case it could have torn apart the fake fruits further along the verge. During preliminary tests, we observed that stems of smaller diameters were slightly folded by the machine while larger stems were more easily broken, which could also favour accumulation of material on the machine. The majority of trapped seeds were F. rubra (i.e. 87% of all seeds trapped), and, interestingly, the centroids measured for tall fake plant of F. rubra showed a mean distance of dispersal comparable to S. arundinaceus tall fescue (i.e. 3.62 ± 1.59 m, and 3.55 ± 0.86 m, respectively). These results suggest that fake plants provide a reliable estimate of the distance of dispersal in the case of tall plant species producing elongated seeds.
In our study, we described the pattern of seed dispersal by considering each seed trapped, centroid and gravity centre. While estimating the pattern of dispersal of each seed allowed us to highlight some particular patterns of dispersal, the centroids and gravity centres provided more general information about seed shadows. This stresses the need to consider several metrics for better understanding of seed dispersal patterns.
Our analysis of the spatial dispersal patterns showed that although the mowing machinery used on berms projected seeds in the direction of the adjacent fields, no seeds reached the field margins. However, in other sites the distance between the mown berm and the arable field can be shorter than 1.4 m; therefore, similar studies should be conducted in other environmental contexts, including species producing seeds adapted to wind dispersal (e.g. with a pappus), which may behave differently. Furthermore, our results suggest that when the embankment is mown, dispersal of seeds within the first metres of the field margin is likely to occur, which stresses the importance of repeating the experiment both in space and time. In the present study, the setting up of the experiment depended on the period of mowing designated by the local authority, and on farmers’ agreement, which strongly limited the choice of site and the day of the experiment. In this context, the colouring of seeds within the standing vegetation was limited to species producing seeds at the time of the experiment, which restrained the type of seed morphologies tested. In addition, the colouring of each seed was particularly difficult and a correct assessment of the number of seeds was impossible and too time consuming. At first glance, colouring of plant seeds within the standing vegetation is quite quick and easy to set up, but the main disadvantage is that when seeds are enclosed in fruits or other structures, they cannot be painted. However, even if the fruit is the main dispersal unit, the mower’s blades destroy this unit to release the seeds. Fake plants, such as those used in this study, provide a way to overcome these drawbacks in order to study the influence of seed dispersal on plant population and community dynamics, and have proven valuable here. Thus, fake plants could be improved by (1) using a less rigid stem of small diameter, such as stubble, (2) planting stems at greater depth and (3) improving the design of the fake fruits to mimic real diaspores structures as far as possible.
Notes on contributors
Clémence Chaudron is a teaching assistant at Polytech Tours. The main focus of her research activity is the effects of environmental factors at landscape scale over time and at local scale (management practices) on plant communities. Contribution: She designed the research, collected and analysed the data, and wrote the draft version.
Francis Isselin-Nondedeu is an associate professor in ecology, conducting research in plant community ecology, dispersal ecology and restoration ecology. Contribution: He co-designed the research, reviewed the manuscript, and was the scientific coordinator of the project.
Disclosure statement
No potential conflict of interest was reported by the authors.
Funding
This work was supported by the Région Centre Council under Grant number IRMA.
Supplemental data
The supplemental material for this paper is available online at https://doi.org/10.1080/23818107.2017.1369901
TABG_1369901_Supplementary_material.zip
Download Zip (157.5 KB)Acknowledgements
This study was supported by the international cluster Vegepolys. We also thank L. Frindel, N. Bonvallet, M. Thomas, V. Bouthonnier, M. Guimier and L. Albert for their help in the field survey; L. Ridet for his help in the planning of the experiment; and A. Mullot, for letting us work in his field. We also thank the two anonymous reviewers who helped us improve the manuscript.
References
- Aerts, R., W. Maes, E. November, M. Behailu, J. Poesen, J. Deckers, M. Hermy, and B. Muys. 2006. “Surface Runoff and Seed Trapping Efficiency of Shrubs in a Regenerating Semiarid Woodland in Northern Ethiopia.” Catena 65 (1): 61–70. doi:10.1016/j.catena.2005.09.004.
- Alverson, W. S., and A. G. Diaz. 1989. “Measurement of the Dispersal of Large Seeds and Fruits with a Magnetic Locator.” Biotropica 21:61-63.10.2307/2388443
- Auffret, A. G., and S. A. O. Cousins. 2013. “Grassland Connectivity by Motor Vehicles and Grazing Livestock.” Ecography 36 (10): 1150–1157. doi:10.1111/j.1600-0587.2013.00185.x.
- Bertrand, F., and M. F. Bertrand. 2012. “Package ‘BioStatR’.” Europe 5: 1.
- Bischoff, A. 2005. “Analysis of Weed Dispersal to Predict Chances of Re-Colonisation.” Agriculture, Ecosystems & Environment 106 (4): 377–387. doi:10.1016/j.agee.2004.09.006.
- Bullock, J. M., K. Shea, and O. Skarpaas. 2006. “Measuring Plant Dispersal: An Introduction to Field Methods and Experimental Design.” Plant Ecology 186 (2): 217–234. doi:10.1007/s11258-006-9124-5.
- Chaudron, C., B. Chauvel, and F. Isselin-Nondedeu. 2016. “Effects of Late Mowing on Plant Species Richness and Seed Rain in Road Verges and Adjacent Arable Fields.” Agriculture, Ecosystems & Environment 232: 218–226. doi:10.1016/j.agee.2016.03.047.
- Clifford, H. T. 1956. “Seed Dispersal on Footwear.” Proceedings of the Botanical Society of British Isles 2: 129–131.
- Greene, D. F., and E. A. Johnson. 1993. “Seed Mass and Dispersal Capacity in Wind-Dispersed Diaspores.” Oikos 67 (1): 69–74. doi:10.2307/3545096.
- Hensen, I., and C. Müller. 1997. “Experimental and Structural Investigations of Anemochorous Dispersal.” Plant Ecology 133 (2): 169–180. doi:10.1023/A:1009744518223.
- Infoclimat. 2017. “Association Infoclimat.” Accessed March 10, 2017. https://www.infoclimat.fr/climatologie/annee/2014/tours-parcay-meslay/valeurs/07240.html.
- Isselin-Nondedeu, F., F. Rey, and A. Bédécarrats. 2006. “Contributions of Vegetation Cover and Cattle Hoof Prints towards Seed Runoff Control on Ski Pistes.” Ecological Engineering 27 (3): 193–201. doi:10.1016/j.ecoleng.2006.02.006.
- Lemke, A., M. von der Lippe, and Ingo Kowarik. 2009. “New Opportunities for an Old Method: Using Fluorescent Colours to Measure Seed Dispersal.” Journal of Applied Ecology 46 (5): 1122–1128. doi:10.1111/j.1365-2664.2009.01691.x.
- Levine, J. M., and D. J. Murrell. 2003. “The Community-Level Consequences of Seed Dispersal Patterns.” Annual Review of Ecology, Evolution and Systematics 34: 549-574. doi: 10.1146/annurev.ecolsys.34.011802.132400
- Maumy-Bertrand, M., and F. Bertrand. 2010. Initiation à La Statistique Avec R: Cours, Exemples, Exercices Et Problèmes Corrigés [Inititation to Statistics with R: Lessons, Examples, Exercises and Corrected Problems]. Dunod.
- Météo France. 2017. Accessed March 10, 2017. https://www.meteofrance.com/climat/france/tours/37179001/normales.
- Nathan, R., G. Perry, J. T. Cronin, A. E. Strand, and M. L. Cain. 2003. “Methods for Estimating Long-Distance Dispersal.” Oikos 103 (2): 261–273. doi:10.1034/j.1600-0706.2003.12146.x.
- Petit, S., A. Alignier, N. Colbach, A. Joannon, D. Le Cœur, and C. Thenail. 2013. “Weed Dispersal by Farming at Various Spatial Scales. a Review.” Agronomy for Sustainable Development 33 (1): 205–217. doi:10.1007/s13593-012-0095-8.
- Pickering, C., and A. Mount. 2010. “Do Tourists Disperse Weed Seed? A Global Review of Unintentional Human-Mediated Terrestrial Seed Dispersal on Clothing, Vehicles and Horses.” Journal of Sustainable Tourism 18 (2): 239–256. doi:10.1080/09669580903406613.
- Pickering, C. M., A. Mount, M. C. Wichmann, and J. M. Bullock. 2011. “Estimating Human-Mediated Dispersal of Seeds within an Australian Protected Area.” Biological Invasions 13 (8): 1869–1880. doi:10.1007/s10530-011-0006-y.
- Pons, J., and J. G. Pausas. 2007. “Acorn Dispersal Estimated by Radio-Tracking.” Oecologia 153 (4): 903–911. doi:10.1007/s00442-007-0788-x.
- R Development Core Team. 2013. R: A Language and Environment for Statistical Computing. Vienna, Austria: R foundation for statistical computing.
- Rabinowitz, D., and J. K. Rapp. 1981. “Dispersal Abilities of Seven Sparse and Common Grasses from a Missouri Prairie.” American Journal of Botany 68 (5): 616–624. doi:10.2307/2442788.
- Rew, L. J., R. J. Froud-Williams, and N. D. Boatman. 1996. “Dispersal of Bromus sterilis and Anthriscus sylvestris Seed within Arable Field Margins.” Agriculture, Ecosystems & Environment 59 (1-2): 107–114. doi:10.1016/0167-8809(96)01038-9.
- Skarpaas, O., O. E. Stabbetorp, I. Ronning, and T. O. Svennungsen. 2004. “How Far Can a Hawk’s Beard Fly? Measuring and Modelling the Dispersal of Crepis praemorsa.” Journal of Ecology 92 (5): 747–757. doi:10.1111/j.0022-0477.2004.00915.x.
- Strykstra, R. J., G. L. Verweij, and J. P. Bakker. 1997. “Seed Dispersal by Mowing Machinery in a Dutch Brook Valley System.” Acta Botanica Neerlandica 46 (4): 387–401. doi:10.1111/plb.1997.46.4.387.
- Tackenberg, O., P. Poschlod, and S. Bonn. 2003. “Assessment of Wind Dispersal Potential in Plant Species.” Ecological Monographs 73 (2): 191–205. doi:10.1890/0012-9615(2003)073[0191:AOWDPI]2.0.CO;2.
- Taylor, K., T. Brummer, M. L. Taper, A. Wing, and L. J. Rew. 2012. “Human-Mediated Long-Distance Dispersal: An Empirical Evaluation of Seed Dispersal by Vehicles.” Diversity and Distributions 18 (9): 942–951. doi:10.1111/j.1472-4642.2012.00926.x.
- Thomson, F. J., A. T. Moles, T. D. Auld, and R. T. Kingsford. 2011. “Seed Dispersal Distance is More Strongly Correlated with Plant Height than with Seed Mass.” Journal of Ecology 99 (6): 1299–1307. doi:10.1111/j.1365-2745.2011.01867.x.
- Vander Wall, S. B., M. I. Borchert, and J. R. Gworek. 2006. “Secondary Dispersal Of Bigcone Douglas-Fir (Pseudotsuga Macrocarpa) Seeds.” Acta Oecologica 30 (1): 100–106. doi:10.1016/j.actao.2006.02.004.
- VanDerWal, J., L. Falconi, S. Januchowski, L. Shoo, C. Storlie, and M. J. VanDerWal. 2014. “SDMTools: Species Distribution Modelling Tools: Tools for Processing Data Associated with Species Distribution Modelling Exercises.” R package version 1: 1–221.
- Venable, D. L., and J. S. Brown. 1988. “The Selective Interactions of Dispersal, Dormancy, and Seed Size as Adaptations for Reducing Risk in Variable Environments.” American Naturalist:360-384.10.1086/284795
- Von der Lippe, M., J. M. Bullock, I. K. owarik, T. Knopp, and M. Wichmann. 2013. “Human-Mediated Dispersal of Seeds by the Airflow of Vehicles.” PLoS ONE 8 (1): e52733. doi:10.1371/journal.pone.0052733.
- Vitalos, M., and G. Karrer. 2009. “Dispersal of Ambrosia Artemisiifolia Seeds along Roads: The Contribution of Traffic and Mowing Machines.” In Biological Invasions: Towards a Synthesis, Proceedings, edited by P. Pyšek, and J. Pergl 8:53-60.
- Ware, C., D. M. Bergstrom, E. Muller, and I. G. Alsos. 2012. “Humans Introduce Viable Seeds to the Arctic on Footwear.” Biological Invasions 14 (3): 567–577. doi:10.1007/s10530-011-0098-4.
- Weiss, F., T. J. Brummer, and G. Pufal. 2016. “Mountain Bikes as Seed Dispersers and Their Potential Socio-Ecological Consequences.” Journal of Environmental Management 181: 326–332. doi:10.1016/j.jenvman.2016.06.037.
- Xiao, Z., P. A. Jansen, and Z. Zhang. 2006. “Using Seed-Tagging Methods for Assessing Post-Dispersal Seed Fate in Rodent-Dispersed Trees.” Forest Ecology and Management 223 (1-3): 18–23. doi:10.1016/j.foreco.2005.10.054.
- Zwaenepoel, A., P. Roovers, and M. Hermy. 2006. “Motor Vehicles as Vectors of Plant Species from Road Verges in a Suburban Environment.” Basic and Applied Ecology 7 (1): 83–93. doi:10.1016/j.baae.2005.04.003.