Abstract
Individuals within wild populations differ substantially in their fitness as a result of either genetic differences or acclimation. Within the Charophyte algae, the two taxa Chara baltica and C. liljebladii predominate at different water depths of the same habitat. The two taxa are mainly distinguished by quantitative characteristics, pointing to light acclimation. In particular, they differ by a length of the internode and the bract cells, as well as the cortication type. Genetic analyses revealed that individuals of both morphotypes are genetically identical, and hence may belong to the same species. In the present paper, we tested a hypothesis that C. liljebladii is a low-light phenotype of C. baltica. Can a C. baltica phenotype be transferred into a C. liljebladii (and vice versa) by manipulation of the environmental conditions such as irradiance? We observed significant changes in the morphology of C. baltica: decreasing the irradiance significantly increased the growth of the branchlets and internodal cells. Consequently, the plants grew larger, changing their morphology in the direction of the C. liljebladii morphotype. In the reverse experiment, subjecting C. liljebladii to increasing irradiances, the individuals had slightly better growth, but none of the analysed morphological characteristics changed significantly. Both taxa have thus shown different adaptations to light limitation. Their morphologies cannot be transferred into each other by environmental factors. Thus, the presence of the different morphologies reflects the ecological characteristics of their habitats, such as light availability or turbidity.
Introduction
Individuals within wild populations can differ substantially in their fitness. It is well established that the phenotype (morphology and physiology) is far from homogeneous among specimens within populations. Such variations are usually driven by the genetic differences among individuals, given the populations’ ability to respond adaptively to natural selection caused by environmental changes (Burt Citation2000; Knight Citation1889). Alternatively, individuals with identical genotypes may exhibit distinct phenotypes when exposed to different environments. Such individual phenotypic plasticity can be defined as a reaction to changing environmental conditions by altering the individual’s morphological, physiological or developmental traits during its lifetime (e.g. Stearns Citation1989). In contrast to long-term genetic evolution, within-generation variation is almost always non-genetic, occurs in individuals, and is frequently adaptive, because it allows the individuals to adjust to environmental variation in their life time (Sultan Citation2003).
Sessile organisms like plants must acclimate to the specific conditions of their habitat, such as irradiance and nutrient availability (Pigliucci Citation2005). These adaptations include morphological changes, such as plasticity in size, shape or resource allocations. Consequently, a high phenotypic variation among individuals of the same species is often observed. However, this plasticity can lead to specific phenotypic appearances under specific environmental conditions (e.g. Munda and Kremer Citation1997; Sultan Citation2000). Such diversity of growth forms might be easily mistaken and, following the phenotypic species concept, regarded as a distinct species.
Light is a fundamental resource for plants and algae. In addition to physiological acclimation mechanisms, most plants exhibit a certain degree of morphological plasticity to adapt to the specific irradiance climate of their habitat. For instance, shading promotes stem elongation, increases in the chlorophyll level and the number of leaves, as well as changes in the leaf structure (Franklin and Whitelam Citation2005; Pigliucci Citation2005; Schneider, Ziegler, and Melzer Citation2006).
Several authors reported on the large morphological plasticity of a number of charophyte species (green algae group Charophyceae). Most of the literature concentrates on the light conditions (e.g. Küster, Schaible, and Schubert Citation2000, Citation2004; Schneider, Ziegler, and Melzer Citation2006), but also includes salinity and its cross-effects with irradiance (e.g. Blindow, Hargeby, and Andersson Citation2002; Blindow and Schütte Citation2007; Boegle et al. Citation2010a, Citation2010b).
These investigations are of special interest with respect to the ongoing discussion about the applicability of the two different taxonomic concepts in use for charophytes: The macrospecies concept of Wood (Citation1962) and the microspecies concept followed by Krause (Citation1997). The latter one is especially popular in Europe. Whereas Wood and Imahori (Citation1965) described 81 macrospecies world-wide in their monograph, Krause (Citation1997) already distinguished 45 microspecies in the very restricted region of Europe. This diversity of morphological detail was thought to be a result of acclimation (variety and forms) by Krause (Citation1997) or of a minor genetic variation (subspecies) by Wood and Imahori (Citation1965).
A main focus in this ongoing discussion is the “Chara hispida group”. The differentiation between C. baltica (Hartman) Bruzelius 1852, C. intermedia Braun 1859, C. horrida Wahlstedt 1862 and C. liljebladii Wallman 1852 by means of molecular genetics and physiological parameters was not possible (Boegle et al. Citation2010a, Citation2010b). Thus the authors support the macrospecies concept of Wood (Citation1962) for this group of microspecies. Consequently, C. liljebladii is ranked as a subspecies even by the authors following the microspecies approach: C. baltica subsp. liljebladii (Wallman) Nyman 1884 or variety C. baltica var. liljebladii (Wallman) A.Braun 1859. In the macrospecies nomenclaure of Wood (Citation1962) the taxon is ranked as a form of C. hispida: C. hispida var. baltica f. liljebladii (Wallm.) R.D.W. comb & stat. nov..
However, further experiments focusing on the quantification of the morphological plasticity within the Chara hispida group are needed to complete the work done by Boegle et al. (Citation2010a). Chara baltica and C. liljebladii are very similar with respect to their morphology. They are distinguished mainly by their relative internode length, which is considerably prolonged for C. liljebladii compared to C. baltica; and the number of ecorticated branchlet segments (Schubert and Blindow Citation2004). In addition, the water depth (an ecological species approach parameter) is often employed for determination. C. liljebladii is found almost exclusively in deeper (0.5–1.5 m) and/or turbid water bodies, whereas C. baltica prefers more shallow waters (Blümel Citation2004; Krause Citation1997), with exception of transparent water bodies. All other parameters overlap between the two species, irrespective of the fact that some of them differ in their ranges.
These considerations suggest, but do not prove, that C. liljebladii might be a low-light acclimation morphotype of C. baltica. To verify this assumption, the conversion of one morphotype to the other must be shown by manipulation of irradiance.
The work presented here set out to demonstrate that a given genotype can be transferred from C. baltica morphology to C. liljebladii morphology – and vice versa – by manipulation of the irradiance conditions.
Materials and Methods
Samples from C. baltica were taken at “Große-Kirr” near Zingst, Germany (54°25ʹ697ʺN; 12°41ʹ404ʺE) at 0.5 m depth and from C. liljebladii at Michaelsdorf, Germany (54°22ʹ17ʺN; 12°34ʹ12ʺE) at 1 m depth. Both these sampling sites belong to a very shallow lagoon (Darss-Zingst Bodden Chain, DZBC) and differ mainly with respect to water depth. The DZBC, located at the southern Baltic Sea coast, contains a rich charophyte community dominated by C. baltica/C. liljebladii (Lindner Citation1972; Yousef and Schubert Citation2001). Large salinity fluctuations between 2.5 and 8.9 (Zingster Strom, means of monthly measurements in 1988–1992), high nutrient availability and pronounced seasonal temperature changes (including ice cover) are characteristic for the DZBC (Schlungbaum, Baudler, and Nausch Citation1994). Further, all parts of the DZBC are exposed to rapidly changing light climate with 1% depth for PAR (the depth at which 1% of subsurface photosynthetic active radiation can still be measured; see Kirk Citation2010) located between 0.8 and 6.0 m (Sagert and Schubert Citation1999).
For the acclimation experiment, 24 individuals identified as C. baltica and 27 as C. liljebladii were subdivided randomly into cylindrical glass vessels. The individual specimens were planted in a mixture of commercial acid-washed silica sand with crystalline tricalcium phosphate, topped with a layer of pure silica sediment (Wüstenberg, Pörs, and Ehwald Citation2011). The glass vessels were filled with a phosphorus-free medium with a salinity of 3.5. The temperature was kept constant at 15°C by external cooling. Plants were cultivated for 28–32 days in a dark-light regime of 9:15 h, using fluorescent tubes (Phillips, TLD 36 W/950). Neutrally absorbing filters were wrapped around the vessels to achieve different irradiances of 30, 90 and 240 μmol photons m−2 s−1. Incident irradiance was adjusted by using a LiCor LI1000 data logger equipped with a spherical underwater sensor LI193 (LiCor Inc., U.S.A.). The position of the glass vessels was changed every day to balance small-scale variations in light supply.
The experiments were started using 8–10 specimens for each species and irradiance level. The size of each specimen was recorded after a pre-incubation of five days and measured weekly with a ruler. The elongation rate (mm day−1) was calculated from the increase in length during the period of linear growth. The morphology was characterized before the acclimation experiment by means of an image-analysis program (ImageJ 1.46r, Abramoff, Magalhaes, and Ram Citation2004). The following morphological traits were quantified: the length of head, internodes, branchlets, end-cells, upper and lower stipulodes, bract cells and spine cells; the diameter of internodes and cortex cells; the number of ecorticated segments of branchlets – each for the first and second whorl following after the head of the Chara plant (for details of the morphology see Schubert, Holzhausen, and Nowak Citation2016). The individual plants were then cultivated at the three different irradiances and at the end of the incubation phase, morphological parameter acquisition for the first and second whorl (new grown parts) of 18 individuals of C. baltica and 24 of C. liljebladii was repeated, as described above.
The individual morphological traits were analysed with the IBM SPSS Statistics software (Version 2.0 IBM, USA). Mann–Whitney U tests or Kruskal–Wallis tests were conducted for each single characteristic to test for significant differences between the analysed morphotypes of C. baltica and C. liljebladii or among the different irradiances. Statistical analyses of the full set of morphological characters, determined before and after the experiment, were conducted by the Primer 5 software (Clarke Citation2001). For the following tests, metric data were pretreated with square root transformation. These calculations were also based on Euclidean Distance matrices. The differences between data of both taxa, cultivated at the three irradiance intensities (corresponding to six different groups), were tested for statistical significance with the Analysis of Similarity (ANOSIM, Clarke and Green Citation1988). Here an R value indicates the difference between tested groups, taking into account within-group variability (Clarke and Warwick Citation2001). High R values are calculated for low variability within the groups tested, compared to the differences between the groups (Clarke and Warwick Citation2001). Additionally, the Similarity Percentages Analysis (SIMPER, Clarke Citation1993) was used to determine similarities and dissimilarities within and between the six groups. The principal component analysis (PCA) was employed to identify the decisive parameters for the detected differences.
Results
Growth/elongation during the experiment
Figure gives an overview of the irradiance dependency of growth for the two taxa. Shoot elongation of C. baltica specimens increased the most at the lowest light intensity (30 μmol photons m−2 s−1) with 1.9 ± 1.0 mm d−1. After the experiment, these algae were more than twice as long as the individuals incubated at 240 μmol photons m−2 s−1. The algae exposed to the highest light intensity of 240 μmol photons m−2 s−1 were showing an average growth of 0.4 ± 0.4 mm d−1. Thus, irrespective of the large differences in the growth rate of C. baltica individuals, a consistent trend of increasing shoot elongation with decreasing irradiance was obvious, resulting in a significant difference between growth rates at the highest and the lowest applied irradiances. In contrast, the growth of plants of C. liljebladii, exposed to the highest light intensity, increased with a rate of 1.4 ± 0.5 mm d−1. The specimens exposed to the other light intensities were only slightly shorter with an average growth of 1.1 ± 0.5 and 1.0 ± 0.4 mm d−1, respectively. After the experiment, algae exposed to 240 μmol photons m−2 s−1 were 25% longer than the individuals incubated with 30 μmol photons m−2 s−1. In contrast to C. baltica, neither an increased shoot elongation with decreasing light intensity nor any other significant irradiance dependency of elongation growth was observed in C. liljebladii. However, the growth rates of C. baltica and C. liljebladii differed significantly at the highest irradiance applied here.
Morphology before the experiment
While C. baltica and C. liljebladii specimens are quite similar with respect to their morphology, mean values of 25 of the 28 measured characteristics showed significant differences (Table ). For example, the relative internode length was considerably prolonged for C. liljebladii compared to C. baltica and the relative internode diameter was smaller for C. liljebladii compared with C. baltica. However, all the characteristics overlapped between the two groups. Thus, as it is the case for several charophyte taxa, a clear delineation between C. baltica and C. liljebladii cannot be made by means of a single morphological parameter.
Table 1. Overview of the measured characteristics before the experiments for both taxa.
Analysing the full set of parameters by means of PCA (Figure ), the individuals of the two taxa clustered in distinct groups, but almost all the parameters contributed equally to the discrimination between the groups (Table S1). A pairwise test of ANOSIM revealed a global R value of 0.423 that indicated significant differences between both taxa (Table ). While the limit for differentiation was 0.5, the divergence of C. baltica and C. liljebladii was rather low. SIMPER-Analysis confirmed these results; the average dissimilarity of 17.6% between both taxa was comparably low (Table ).
Figure 2. PCA of C. baltica (squares) and C. liljebladii (triangles) specimens before the acclimation experiment.
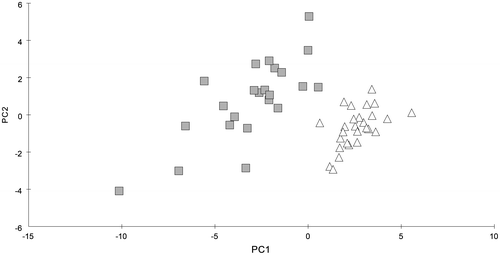
Table 2. Pairwise R values before (above diagonal) and after (below diagonal) the experiment for both taxa, incubated at three irradiances: 30, 90 and 240 μmol photons m−2 s−1.
Table 3. Mean dissimilarity (%) before (above diagonal) and after (below diagonal) the experiment for both taxa incubated at three irradiances of 30, 90 and 240 μmol photons m−2 s−1.
Morphology after the experiment
Chara baltica
After the acclimation experiment, the morphological differences between the two taxa were still detectable. For C. baltica characteristics, connected with the internode and branchlet parameters, an irradiance dependency was demonstrated (Table ). For the first and the second whorl, an increase in internode and branchlet length with decreasing irradiance was observed. Interestingly, the trend was more pronounced for the second whorl, indicating that the shoot elongation proceeds, while the next internode is under development. The Kruskal–Wallis tests for single parameters (Table ) revealed a significant difference between the morphological data of the highest and the lowest light intensities. The pairwise-tests of ANOSIM for all of the measured parameters indicated significant differences between the specimens of C. baltica incubated at 30 and 240 μmol photons m−2 s−1 (R value of 0.567). The R values for the comparison of the 90 μmol photons m−2 s−1 group with the 30 and 2400 μmol photons m−2 s−1 group (0.32 and 0.21, respectively) must be interpreted as a sign of similarity. This trend indicates that the differences between the highest and the lowest irradiance group, which allow for a clear distinction between the respective morphologies, are connected via the intermediate group. Herein we see a clear sign of a morphological continuum along the irradiance gradient of C. baltica. This finding is also reflected by the result from the PCA of the morphological data, measured after irradiance acclimation (Figure ). Except for one specimen, all the individuals of C. baltica acclimated to 30 μmol photons m−2 s−1 fall within one separate cluster. Looking at all the data, two more subdivisions can be distinguished: one group which includes most of the plants subjected to 90 μmol photons m−2 s−1 and another cluster composed mostly of plants exposed to 240 μmol photons m−2 s−1. However, the small distances between these subdivisions suggest that there are no significant differences between the high-light and intermediate-light acclimated C. baltica specimens.
Table 4. Kruskal–Wallis test of the measured characteristics after the experiment for the taxon C. baltica.
Chara liljebladii
The development of morphological parameters for C. liljebladii was clearly different in comparison to the results of C. baltica. A homogeneous distribution for the morphological parameters (length of internode) was detected and no significant differences among the three light intensities could be observed. Thus, an irradiance dependency of characteristics could not be demonstrated for C. liljebladii. Pairwise tests of ANOSIM, with R values between –0.048 and 0.048, showed a high degree of similarity among the analysed C. liljebladii plants, incubated at different light intensities. The PCA confirmed these results (Figure ). The individuals of C. liljebladii formed one single cluster without consistent differences between the specimens incubated at the three irradiances.
C. baltica vs. C. liljebladii
After the acclimation to the three light intensities, the differentiation between C. baltica and C. liljebladii was more pronounced than before the experiment. The Mann–Whitney U tests revealed a decreasing number of significantly different parameters between both taxa (Table ), but R values and averages of dissimilarity revealed an increased quality of those characteristics. The R values between 0.6 and 0.8 (Table ) and dissimilarities between 23.5 and 28.1% (Table ) between the C. baltica and C. liljebladii groups demonstrated differences among all the light intensities tested. In contrast, before the acclimation experiment the R values were ≤ 0.5 and dissimilarities ≤ 19% between the taxa (Tables and ). The PCA of the morphological characteristics after the acclimation consequently reveals two clearly separated clusters with C. baltica being more variable compared to C. liljebladii (Figure ). Again, no main parameter(s) contribute to the discrimination between the groups (Table S2).
Table 5. Overview of the measured characteristics after the experiments for both taxa.
Discussion
To distinguish between C. baltica and C. liljebladii, three morphological parameters are used in identification keys: length of internode, cortication and length of bract cells. All the other parameters found in the literature overlap to a degree, which does not allow discrimination between the taxa. Further, the parameters in use for discrimination also show some overlap, hampering clear separation of the specimens with intermediate morphology. Therefore a fourth parameter, water depth, is often employed to distinguish between the morphotypes (Boegle et al. Citation2010a). However, water depth is related to irradiance and can also be regarded as a trigger for morphological acclimation. To decide upon the taxonomic status of C. liljebladii, the effect of irradiance on morphology was tested.
Since in this study depth (water pressure) and turbulence were similar for all groups, the irradiance availability was assumed to be the decisive parameter for the morphological differences between the two morphotypes.
In our experiments, the individuals that were clearly determined as C. baltica, show a distinct change of their morphology with respect to irradiance conditions. Thus, C. baltica individuals seem to be able to exhibit variable morphotypes under different environmental conditions. Depending on irradiance, C. baltica changed the length of their internodes and bract cells significantly. Furthermore, light-dependent differences in cortication and the length of bract cells were observed and are in good agreement with published data (Wood and Imahori Citation1965). Interestingly, the differences between the experimental groups with regard to the internode and branchlet length are most pronounced at the second whorl. This finding indicates a progressive and reproducible acclimation in response to a change in the environment, which is in a good agreement with the assumption of an ongoing acclimation during the experiments. With decreasing irradiance availability, the shoot and branchlet elongation increased. This response is a typical low-light acclimation strategy, known from many terrestrial higher plants as well as aquatic macrophytes (e.g. Barko, Hardin, and Matthews Citation1982), including charophytes (e.g. Blindow et al. Citation2003; Küster, Schaible, and Schubert Citation2004; Schaible et al. Citation2012).
In contrast, the individuals that were clearly determined as C. liljebladii, showed a slightly improved growth after their acclimation to an increasing light intensity, but none of the analysed morphological parameters exhibited a correlation with irradiance. The results indicate that C. liljebladii individuals are relatively insensitive to differences in the light conditions. They do not respond to the increased light conditions by shortening of their internodes and bract cells and by reducing their stem length, a typical protection scenario against high light, observed for charophytes (e.g. Blindow, Dietrich, Möllmann et al. 2003) as well as for higher plants (e.g. Kurepin and Pharis Citation2014). Such an adaptation strategy is less frequent in the literature, but was observed in some species within the genus Chara for C. intermedia (Schneider, Ziegler, and Melzer Citation2006) and a parthenogenetic population of C. canescens (Schaible et al. Citation2012). It is remarkable that a sessile plant, which adapts to low-light conditions like C. liljebladii, does not avoid high-light conditions by, for instance, reducing elongation growth and higher branching. Possibly, the individuals follow another strategy to avoid damage by high-light conditions by changing the photosystem PSII:PSI ratio or chlorophyll (Chl) a:b ratio or by an increased production of light protecting pigments like carotenoids. Such a physiological adaptations were observed in Chara, but mostly combined with reduced growth characteristics (e.g. Küster, Schaible, and Schubert Citation2004; Schaible and Schubert Citation2008; Schaible et al. Citation2012). While we did not analyse the pigmentation and the photosynthetic adaptation, the increased growth at highest irradiance indicated that C. liljebladii specimens were not light saturated within the chosen irradiances, whereas the C. baltica individuals were strongly light saturated.
In contrast to C. baltica individuals, for C. liljebladii individuals no change in their phenotype could be observed, if the morphology before and after the experiment was compared. While no genetic differences could be found so far between the field-grown individuals of the two morphological appearances (Boegle et al. Citation2010a), we could observe clear differences in morphology and a taxa-specific response in changing irradiance conditions: C. liljebladii produces a specific and light-insensitive morphotype, whereas for C. baltica variable and light-sensitive morphotypes could be observed.
Although the above-mentioned observations seem contradictory and puzzling at first, we will try to give two short hypothetical and possible explanations:
1. In the genus Chara, high morphological variation, combined with a genetic uniformity, was observed across several species (see Boegle et al. Citation2010a; Nowak, Schubert, and Schaible Citation2016). Since the whole genomes have not been compared for the two taxa, genetic variation cannot be completely excluded, such that the significant morphological differences between C. liljebladii and C. baltica could also be due to the genetic differences between the taxa.
2. In contrast, the production of both light-insensitive and light-sensitive phenotype could imply an adaptive life history strategy, controlled by the same genotype. The production of such exceptionally different phenotypes could be favoured by a very heterogeneous irradiance conditions. In our experiments, using plant material as a starting point, post-germinated predefinition of morphological acclimation ranges cannot be excluded. Whereas the C. baltica-morphotype still bears the full range of low-light acclimation, the C. liljebladii morphotype, once initiated, might be locked in the low-light acclimation mechanisms. Regarding the high degree of variability in the underwater light climate, such a fixed low-light acclimation for the deep-water growing individuals will secure correct acclimation, even during periods of low turbidity or bright weather, and satisfies the demand and availability of energy for adaptive acclimation. The different morphotypes are adapted to a narrow range of ecological conditions and, depending on their niche type, could be found locally abundant. The variable morphotypes of C. baltica, growing in a niche with a better energy supply, are able to acclimate almost in “real time”, responding to water-level changes and extreme variation of light conditions. The light-insensitive morphotype of C. liljebladii starts its growth under very low and relatively invariant light conditions. It does not invest in the high-light acclimation in the periods of sporadically increasing light and consequently demonstrates higher fitness in deeper waters. This reasoning is supported by the observation of recent studies that C. liljebladii is restricted to light-limited habitats, because of either water depth or turbidity (Blümel Citation2004; Boegle et al. Citation2010a). Additionally, such relationships have already been described for other Chara species that are genetically uniform (Nowak, Schubert, and Schaible Citation2016), for instance, calcium-rich or -poor habitat conditions for C. strigosa and C. virgata (Rey-Boissezon and Auderset Joye Citation2015).
Since both genetic variation and environmental variation contribute to the different expression of phenotypes, future studies will determine which component is more important for Chara. In such studies, it should be tested whether higher irradiances could create changes in the morphology of C. liljebladii. Furthermore, it is also important to analyse whether other single-stress treatments or combined-stress environments provoke differences in C. liljebladii morphology. Importantly, such experiments should be done with adult plants and with freshly germinated prochara.
The results of the acclimation experiment revealed a sudden shift in morphology of C. baltica, most pronounced with respect to the internode and branchlet length, at an irradiance below 90 μmol photons m−2 s−1. The result of this shift coincides with the characteristics used for the species delineation between C. baltica and C. liljebladii. Such significant shifts in morphology owing to certain light intensities were not observed in C. liljebladii. However, the results for the acclimation experiments of C. liljebladii revealed significant differences between C. baltica and C. liljebladii. Rather, both taxa, while morphologically very similar, showed no overlapping forms and were significantly different from each other. Thus, we suggest that genetically identical taxa should not be grouped together per se as one single species.
Disclosure statement
We confirm that there are no known conflicts of interest associated with this publication.
Funding
The work was supported in part by a BMBF-grant to HS 03F0665 (BACOSA) and by the European Regional Development Fund (ERDF, UHRO26).
Notes on contributors
Petra Nowak (corresponding author) is a marine biologist specializing in systematic of macroalgae and working at the University of Rostock. Author’s contribution: general concept and research question, data analysis, interpretation of results, manuscript preparation.
Hendrik Schubert is a marine biologist specializing in aquatic ecology and working at the University of Rostock. Author’s contribution: general concept and research question, data analysis, interpretation of results, manuscript preparation.
Anja Holzhausen is a marine biologist specializing in benthic ecology and working at the University of Rostock. Author’s contribution: general concept and research question, data analysis, interpretation of results, manuscript preparation.
Veronika Sommer is a marine biologist specializing in phycology and working at the University of Rostock. Author’s contribution: general concept and research question, data analysis, interpretation of results, manuscript preparation.
Ralf Schaible is a marine biologist specializing in evolutionary biodemography and working at the Max-Planck-Institute for Demographic Research. Author’s contribution: general concept and research question, interpretation of results, manuscript preparation.
Supplemental material
The supplemental material for this paper is available online at https://doi.org/10.1080/23818107.2017.1374209
TABG_A_Table_S1_S2.doc
Download MS Word (103.5 KB)Acknowledgements
The authors are very grateful to I. Blindow for fruitful discussion about experimental design and analysis procedures. We would like to thank Arne Schoor for the laboratory and technical assistance. We gratefully acknowledge the valuable comments of two anonymous reviewers and the editors, Elisabeth Dodinet and Andrzej Pukacz, for their helpful suggestions. We also thank Mary Beilby for thorough language editing.
References
- Abramoff, M. D., P. J. Magalhaes, and S. J. Ram. 2004. “Image Processing with ImageJ.” Biophotonics International 11 (7): 36–42.
- Barko, J. W., D. G. Hardin, and M. S. Matthews. 1982. “Growth and Morphology of Submersed Freshwater Macrophytes in Relation to Light and Temperature.” Canadian Journal of Botany 60 (6): 877–887.10.1139/b82-113
- Blindow, I., and M. Schütte. 2007. “Elongation and Mat Formation of Chara aspera under Different Light and Salinity Conditions.” Hydrobiologia 584 (1): 69–76.10.1007/s10750-007-0578-9
- Blindow, I., A. Hargeby, and G. Andersson. 2002. “Seasonal Changes of Mechanisms Maintaining Clear Water in a Shallow Lake with Abundant Chara Vegetation.” Aquatic Botany 72 (3-4): 315–334.10.1016/S0304-3770(01)00208-X
- Blindow, I., J. Dietrich, N. Möllmann, and H. Schubert. 2003. “Growth, Photosynthesis and Fertility of Chara aspera under Different Light and Salinity Conditions.” Rostocker Meeresbiologische Beiträge 76 (3): 213–234.
- Blümel, C. 2004. “Chapter 4.3 Chara baltica Bruzelius 1824.” In Charophytes of the Baltic Sea, edited by H. Schubert and I. Blindow, 53–63. Ruggell: Gantner.
- Boegle, M. G., S. C. Schneider, A. Melzer, and H. Schubert. 2010a. “Distinguishing Chara baltica, C. horrida and C. liljebladii – Conflicting Results from Analysis of Morphology and Genetics.” Charophytes 2 (2): 53–58.
- Boegle, M. G., S. C. Schneider, H. Schubert, and A. Melzer. 2010b. “Chara baltica Bruzelius 1824 and Chara intermedia a. Braun 1859 - Distinct Species or Habitat Specific Modifications?” Aquatic Botany 93 (3): 195–201.10.1016/j.aquabot.2010.07.001
- Burt, A. 2000. “Perspective: Sex, Recombination, and the Efficacy of Selection – Was Weismann Right?” Evolution 54 (2): 337–351.
- Clarke, K. R. 1993. “Non-Parametric Multivariate Analyses of Changes in Community Structure.” Austral Ecology 18 (1): 117–143.10.1111/aec.1993.18.issue-1
- Clarke, K. R. 2001. Primer v5: User Manual/Tutorial. UK: Plymouth.
- Clarke, K. R., and R. H. Green. 1988. “Statistical Design and Analyses for ‘Biological Effects’ Study.” Marine Ecology Progress Series 46 (1): 213–226.10.3354/meps046213
- Clarke, K. R., and R. M. Warwick. 2001. Change in Marine Communities: An Approach to Statistical Analysis and Interpretation. UK: Plymouth.
- Franklin, K. A., and G. C. Whitelam. 2005. “Phytochromes and Shade-Avoidance Responses in Plants.” Annals of Botany 96 (2): 169–175.10.1093/aob/mci165
- Kirk, J. T. O. 2010. Light and Photosynthesis in Aquatic Ecosystems, 662. Cambridge University Press.10.1017/CBO9781139168212
- Knight, Bert Cyril James Gabriel 1889. “The Significance of Sexual Reproduction in the Theory of Natural Selection.” In Essays upon Heredity and Kindred Biological Problems, edited by S. S. E. B. Poulton, and A. E. Shipley, 330. Oxford: Clarendon Press.10.5962/bhl.title.101564
- Krause, W. 1997. “Charales (Charophyceae).” In Süßwasserflora Von Mitteleuropa [Freshwater Flora of Central Europe], edited by A. Ettl, G. Gärtner, H. Heynig, and D. Mollenhauer, 202. Jena: Fischer.
- Kurepin, L. V., and R. P. Pharis. 2014. “Light Signalling and the Phytohormonal Regulation of Shoot Growth.” Plant Science 229 (1): 280–289.10.1016/j.plantsci.2014.10.006
- Küster, A., R. Schaible, and H. Schubert. 2000. “Light Acclimation of the Charophyte Lamprothamnium papulosum.” Aquatic Botany 68 (3): 205–216.10.1016/S0304-3770(00)00122-4
- Küster, A., R. Schaible, and H. Schubert. 2004. “Light Acclimation of Photosynthesis in Three Charophyte Species.” Aquatic Botany 79 (2): 111–124.10.1016/j.aquabot.2004.01.010
- Lindner, A. 1972. “Soziologisch-ökologische Untersuchungen an der submersen Vegetation der Boddenkette südlich des Darß und des Zingst [Socio-Ecological Studies on the Submerged Vegetation of the Bodden Chain South of Darss and Zingst].” Ph.D dissertation., University of Rostock, Rostock.
- Munda, I. M., and B. P. Kremer. 1997. “Morphological Variation and Population Structure of Fucus spp. (Phaeophyta) from Helgoland.” Nova Hedwigia 64 (1): 67–86.
- Nowak, P., H. Schubert, and R. Schaible. 2016. “Molecular Evaluation of the Validity of the Morphological Characters of Three Swedish Chara Sections: Chara, Grovesia, and Desvauxia (Charales, Charophyceae).” Aquatic Botany 134: 113–119.10.1016/j.aquabot.2016.08.001
- Pigliucci, M. 2005. “Evolution of Phenotypic Plasticity: Where Are We Going Now?” Trends in Ecology and Evolution 20 (9): 481–486.10.1016/j.tree.2005.06.001
- Rey-Boissezon, A., and D. Auderset Joye. 2015. “Habitat Requirements of Charophytes – Evidence of Species Discrimination through Distribution Analysis.” Aquatic Botany 120: 84–91.10.1016/j.aquabot.2014.05.007
- Sagert, S., and H. Schubert. 1999. “Unterwasserlichtklima der Darss-Zingster-Boddenkette [Underwater Light Climate of the Darss-Zingst Bodden Chain].” Rostocker Meeresbiologische Beiträge 7 (1): 135–155.
- Schaible, R., and H. Schubert. 2008. “The Occurrence of Sexual Chara canescens Populations (Charophyceae) is Not Related to Ecophysiological Potentials with Respect to Salinity and Irradiance.” European Journal of Phycology 43 (3): 309–316.10.1080/09670260801930348
- Schaible, R., A. Gerloff-Elias, F. Colchero, and H. Schubert. 2012. “Two Parthenogenetic Populations of Chara canescens Differ in Their Capacity to Acclimate to Irradiance and Salinity.” Oecologia 168 (2): 343–353.10.1007/s00442-011-2113-y
- Schlungbaum, G., H. Baudler, and G. Nausch. 1994. “Die Darß-Zingster Boddenkette – ein typisches Flachwasserästuar an der südlichen Ostseeküste [The Darss-Zingst Bodden Chain – A Typical Shallow Water Estuary on the Southern Baltic Sea Coast].” Rostocker Meeresbiologische Beiträge 2 (1): 5–26.
- Schneider, S., C. Ziegler, and A. Melzer. 2006. “Growth towards Light as an Adaptation to High Light Conditions in Chara Branches.” New Phytologist 172 (1): 83–91.10.1111/nph.2006.172.issue-1
- Schubert, H., and I. Blindow, eds. 2004. Charophytes of the Baltic Sea. Ruggell: Gantner.
- Schubert, H., A. Holzhausen, and P. Nowak. 2016. “Kapitel 6 Individualentwicklung der Characeen [Chapter 6 Ontogenesis].” In Armleuchteralgen. Die Characeen Deutschlands [Stoneworts. Characeae of Germany], edited by A. G. Chara, 57–78. Berlin Heidelberg: Springer.
- Stearns, S. C. 1989. “The Evolutionary Significance of Phenotypic Plasticity: Phenotypic Sources of Variation among Organisms Can Be Described by Developmental Switches and Reaction Norms.” BioScience 39 (7): 436–445.10.2307/1311135
- Sultan, S. E. 2000. “Phenotypic Plasticity for Plant Development, Function and Life History.” Trends in Ecology and Evolution 5 (12): 537–542.
- Sultan, S. E. 2003. “Phenotypic Plasticity in Plants: A Case Study in Ecological Development.” Evolution and Development 5 (1): 25–33.10.1046/j.1525-142X.2003.03005.x
- Wood, R. D. 1962. “New Combinations and Taxa in the Revision of Characeae.” Taxon 11 (1): 7–25.10.2307/1216853
- Wood, R. D., and K. Imahori. 1965. A Revision of the Characeae. First Part: Monograph of the Characeae. Weinheim: Verlag von J. Cramer.
- Wüstenberg, A., Y. Pörs, and R. Ehwald. 2011. “Culturing of Stoneworts and Submersed Angiosperms with Phosphate Uptake Exclusively from an Artificial Sediment.” Freshwater Biology 56 (8): 1531–1539.10.1111/fwb.2011.56.issue-8
- Yousef, M. A. M., and H. Schubert. 2001. “Assessment of the Occurrence of Charophytes in Shallow Coastal Waters of Mecklenburg-Vorpommern, Germany.” Schriftenreihe Landschaftspflege Naturschutz 72 (1): 9–16.